1.1 Selecting the Correct Imaging Modality
Orthopedics and trauma surgery, rheumatology, the vast field of oncology and, to a degree, even biopsy-based pathology must rely heavily on skeletal imaging. Radiologists must be well versed in these areas to ensure that their services will continue to be relevant. What, then, do these specialties require in terms of a good diagnosis? And how should a “good diagnosis” be defined?
First and foremost, a radiologic diagnosis must be useful. It must alter the treatment strategy for a patient. On the other hand, it does not necessarily have to be definitive; it does not have to be the final diagnosis like that supplied by histology, clinical chemistry, molecular biology, or the clinical course. Above all it must be logical and well founded and it must reflect due diligence. That is the only justifiable aspect of a radiologic diagnosis—not its absolute correctness, which may draw even highly specialized experts into a dispute that no judge could resolve.
Every radiologist must provide two essential services:
Selecting the correct imaging modality
Image interpretation
The following imaging modalities should be available and selectively utilized at centers where skeletal examinations are performed:
Plain radiography
CT (computed tomography)
Bone scintigraphy including SPECT (single-photon emission computed tomography)
MRI (magnetic resonance imaging)
PET (positron emission tomography) and PET-CT fusion
Ultrasound
The patients who are seen in the everyday practice of skeletal radiology fall into one of three scenarios:
A patient undergoes initial imaging for the investigation of a clinical problem. The patient has had no previous imaging studies, and ideally the radiologist is challenged to tailor the imaging study to the specific problem, using his or her skills and experience.
A patient is referred for the further investigation of findings noted in one or more previous imaging studies. In this situation the radiologist must select the study that can supply the fastest and most accurate, clinically useful diagnosis.
A patient with an established diagnosis presents for follow-up imaging.
1.1.1 Available Imaging Studies
What, then, are the most suitable and rewarding imaging studies for a particular problem? Which of the procedures listed above will yield the desired information most rapidly and accurately while causing the least amount of patient compromise?
To answer these questions, it is helpful to review briefly the capabilities and limitations of the imaging modalities listed above.
Plain Radiography
Plain radiography creates an image based on differences in the X-ray absorption characteristics of different tissues. Thus, bone tissue or any bony or calcified structures are portrayed in high contrast while most soft tissues are imaged in low contrast, unless contrast medium is used. The main disadvantage of plain radiography in skeletal studies is the presence of summation effects, since radiographs give only a superimposed, two-dimensional view of three-dimensional body structures. Thus, from a modern-day perspective (and based on current requirements), plain radiography is unsuitable for detecting focal lesions (1.5–2 cm in diameter) in body regions that are sensitive to projection effects such as the thoracic and lumbar spine, sacrum, and skull base. On the other hand, there are systemic bone diseases with more or less fine structural changes (e.g., osteopathies such as osteoporosis, hyperparathyroidism, osteomalacia, Paget disease) in which summation effects are diagnostically useful and may detect structural changes that would often be missed in sectional imaging procedures.
Plain radiography is inherently unsuitable for the evaluation of soft-tissue structures such as bone marrow, muscle, tendons, ligaments, cartilage, etc. A major advantage of skeletal plain radiography, which has been in use for over a century, is that valid pattern-recognition algorithms and engrams (cognitive links) have been established that permit the fast and accurate detection of abnormalities. This capability is useful, for example, in the analysis of osteolytic areas by Lodwick grading and for recognizing ossification patterns in a tumor matrix, such as the “ground-glass” pattern for woven bone (see Case 139) and the “popcorn” pattern for cartilaginous matrix (see Case 108).
Computed Tomography
CT basically works like plain radiography by converting tissue X-ray absorption values into an image. The advantage of CT is that it can generate sectional, nonsuperimposed images of body structures with a contrast resolution many times higher than that of conventional radiographs. As a sectional modality, it is particularly useful for providing nonsuperimposed views of the axial skeleton and of complex processes in the appendicular skeleton. It can also provide limited views of soft-tissue structures like muscle, fat, and hematomas. Scanning after the administration of intravenous contrast medium can significantly expand the ability of CT to define soft-tissue structures, especially when the proper window setting is used. CT densitometry permits the accurate identification of fat, fluid, and other media based on their attenuation values. Modern multidetector-row scanners can produce three-dimensional images that are essential for the accurate localization of structures and lesions. From a physical standpoint, the indications for CT are the same as those for plain radiographs, aside from its sectional imaging capabilities. Also, owing to the establishment of pattern-recognition algorithms and engrams with high diagnostic specificity, CT, which is slightly less than 40 years old, has features in common with plain radiography in skeletal examinations and offers numerous advantages over the “younger” modalities of MRI and PET-CT.
Bone Scintigraphy
Bone scintigraphy with Tc-99m (technetium-99m methylene diphosphonate) is an imaging technique based on radioactive tracer uptake in bones and adjacent soft tissues. The amount of tracer uptake is dependent on local bone metabolism, local perfusion, and regional tracer affinity, so that planar “bone scans” with a gamma camera supply information on global, regional, and focal bone metabolism. This is helpful in determining, for example, whether a systemic process with increased bone turnover, such as hyperparathyroidism (see Case 15 and Fig. 6.30 g in Case 111), is present as opposed to a focal or a multifocal process representing a bone metastasis. Bone scans can reliably determine whether a sclerotic lesion is active or not, which may be particularly helpful in oncologic evaluations (see Case 145 and Case 149). Bone scintigraphy, then, is a functional imaging study. Today there is no radiologic technique that can supply such precise information on bone metabolism with “one look” and with a relatively simple protocol. In patients thought to have smaller and less active lesions, another sectional imaging technique, SPECT, is of great value in many cases owing to its ability to provide nonsuperimposed three-dimensional views.
Unfortunately, bone scintigraphy is not widely practiced today despite the fact that it is widely available and supplies functional information not obtainable with CT or MRI. In recent years we have been able to define more than 20 tracer distribution patterns which are highly specific for certain skeletal diseases and refute the common argument against bone scans, namely that “they are not specific enough.”1 The radiation safety aspects of bone scintigraphy will not be explored here, but suffice it to say that radiation exposure is relatively low and such risk is greatly outweighed by the benefits of the study for sick patients.
Magnetic Resonance Imaging
MRI, in which the relaxation times and proton content of body tissues are measured, is a sectional imaging modality that has fundamental physical differences from the X-ray absorption modalities. It is the method of first choice for imaging soft-tissue structures (bone marrow, muscle, tendons, fascia, neurovascular bundles, cartilage, synovial membrane, intra-articular fluid) and currently has a relatively broad role in skeletal investigations. Yet the initial euphoria that this modality could handle all skeletal imaging tasks without X-rays has been tempered by the results of clinical imaging studies. Meanwhile, sound and appropriate indications for MRI have been crystalizing, especially in the diagnosis of articular, bone-marrow and muscular diseases and in the staging of skeletal tumors. It should be noted that bony structures (especially healthy cortical bone) appear as signal voids on MR images and thus can be identified only indirectly; this may lead to problems of differential diagnosis. The same applies to processes that are associated with more or less subtle ossifications or calcifications, which are clearly demonstrable by CT.
The information content of MRI can be increased by dynamic contrast-enhanced imaging (e.g., to assess the perfusion of a lesion) and by diffusion-weighted imaging. But these techniques cannot in themselves provide reliable information on general and local bone metabolism in the strict sense, and unlike bone scintigraphy they cannot answer the question of whether a sclerotic lesion, for example, is active. Unlike plain radiographs, CT scans, and bone scans, MRI still does not have well-established pattern recognition algorithms and engrams (e.g., Lodwick grades, ground-glass appearance, systemic sclerosing diseases), apart from joint trauma, which could guide the radiologist quickly and confidently to a correct diagnosis. Moreover, there is still no good, comprehensive catalog of normal variants like that available for the more conventional modalities.
Not infrequently, problems in the availability of MRI can distort its range of indications. This means that other imaging studies are ordered (e.g., radiographs of the spine and sacroiliac joints for suspected early spondyloarthritis) even though it is known that early enthesitic changes are not detectable on plain radiographs. Or perhaps a pelvic radiograph is ordered for suspected early osteonecrosis of the femoral head, even though ARCO stage I or II disease (ARCO = Association for Research of Circulation Osseous) can be detected only by MRI. At the same time, more or less useless MRIs are being scheduled for, say, lumbago without neurologic deficits and are depriving patients who could truly benefit from an early MRI examination.
Note
A very important rule for MRI protocols: If scanning is performed with contrast medium, the sequences before and after contrast administration should be identical (e.g., T1 weighting before and after contrast injection). Otherwise, possible enhancement cannot be evaluated. Unfortunately this technical principle is often violated in clinical practice. For example, a T1-weighted (T1w) sequence is performed before contrast administration but a fat-suppressed T1w sequence or water-sensitive sequence is performed afterward.
Positron Emission Tomography (and PET-CT)
PET, like bone scintigraphy, is a functional imaging modality. Today, over 90% of all PET scans employ the glucose analog 18F-FDG (18F-2-fluoro-2-deoxyglucose), tagged with the fluorine radioisotope 18F. The method is based on the imaging of a circumscribed change in specific cellular functions, such as the intensity of glucose metabolism as determined by 18F-FDG PET. Increased glucose metabolism is a relatively nonspecific indicator, however, which is seen in inflammatory processes as well as neoplasms and tumorlike lesions. The standard uptake value (SUV) provides a semiquantitative indicator of tumor metabolism (see below).
PET can be combined with simultaneous CT imaging in two interconnected scanners to produce a system known as PET-CT. This integrated system can generate a PET-CT fusion image without having to change the patient position, respiratory position, slice thickness, etc. Increasingly, PET-CT is becoming an established tool in oncologic musculoskeletal studies, as in the diagnosis of lymphoma and plasmacytoma2 , 3 and skeletal metastases. In a recent meta-analysis of 23 studies, however, Liu et al4 found that MRI was superior to 18F-FDG PET and bone scintigraphy for detecting skeletal metastases in breast cancer patients (pooled sensitivity on a per-patient basis: 97.1% for MRI, 83.3% for PET, and 87.0% for bone scintigraphy; pooled sensitivity on a per-lesion basis: 97% for MRI, 94.5% for PET, and 88.1% for bone scintigraphy).
As for the diagnosis of primary bone tumors,5 , 6 to date there have been only scattered publications, based largely on case reports, which do not provide conclusive information (see below). But given the generally low incidence of bone tumors, it is still too early to expect definitive answers.
FDG-PET can be very useful for monitoring bone tumors during treatment, since by its very nature it is accurate in assessing tumor viability. The SUV for FDG provides a semiquantitative measure of metabolic tumor activity. Because tumor metabolism is usually heterogeneous, the SUV in a region of interest (ROI) within the tumor provides an excellent index for evaluating tumor metabolism. Hawkins et al7 investigated the maximum SUV before and after neoadjuvant chemotherapy for the Ewing sarcoma family of tumors. Patients with an SUV of less than 2 after therapy tended to have an excellent response (10% or less viable tumor) with a 4-year disease-free survival. Hawkins et al8 found similar results for osteosarcoma.
Gaston et al9 obtained somewhat different results in their recent study: the change in maximum SUV between baseline examination and post-treatment scanning was not significantly associated with histologic response for either osteosarcoma or Ewing sarcoma. The metabolic tumor volume and the percentage of injected 18F-FDG dose were different in the osteosarcoma and Ewing sarcoma response subgroups. A 50% reduction in metabolic tumor volume was found to be significantly associated with a good histologic response in osteosarcoma but not in Ewing sarcoma. When the cut-off value for Ewing sarcoma was increased to a 90% reduction in metabolic tumor volume, a good correlation was found with histologic response.
These essentially positive results of FDG-PET scans cannot be applied directly to the problem of benign/malignant differentiation (see above). Aoki et al5 found no significant difference between the SUVs of osteosarcoma and giant cell tumor or between fibrous dysplasia and chondrosarcoma; this is not surprising (see above). Feldman et al,6 on the other hand, studied 29 cartilage tumors (11 enchondromas, 6 osteochondromas, 11 chondrosarcomas) and found that 18FDG-PET, using a maximum SUV cut-off of 2.0, could discriminate between malignant and benign lesions with a sensitivity of 90.9%, a specificity of 100%, and an accuracy of 96.6%. On closer analysis, the patients in their study comprised a very heterogeneous group. Bredella et al10 evaluated FDG-PET in differentiating benign from malignant compression fractures (33 patients with 43 fractures). In a total of 14 malignant and 29 benign compression fractures, five cases with a benign fracture were falsely classified as malignant (false-positive). Three of those patients had undergone prior treatment with bone-marrow stimulating agents. There were two false-negative results. Sensitivity, specificity, and accuracy were in the range of approximately 70 to 90%. In our view these results are not too significant when we consider that the patients participating in the study had multiple fractures that most likely had the same cause. Moreover, only nine of the cases were histologically confirmed, and the tumor entities underlying the malignant fractures were extremely heterogeneous. The authors state that the difference between the SUV values for benign and malignant fractures was statistically significant (1.9 ± 0.97 and 3.9 ± 1.52, respectively). Shin et al11 found basically the same results in a similar population, although they performed all examinations with PET-CT and obtained measurements separately for the cortex and bone marrow.
Thus, we find no real consensus in the current literature on the efficacy of FDG-PET in diagnosing bone tumors, aside from initially positive results in the follow-up of Ewing sarcoma and osteosarcoma during treatment. This is to be expected, since this modality is relatively recent and the spectrum of primary and secondary bone tumors is very broad with respect to tumor dynamics and tumor biology. One critical note on the above follow-up studies is that none has yet compared PET with traditional modalities such as MRI, CT, scintigraphy, and plain radiography.
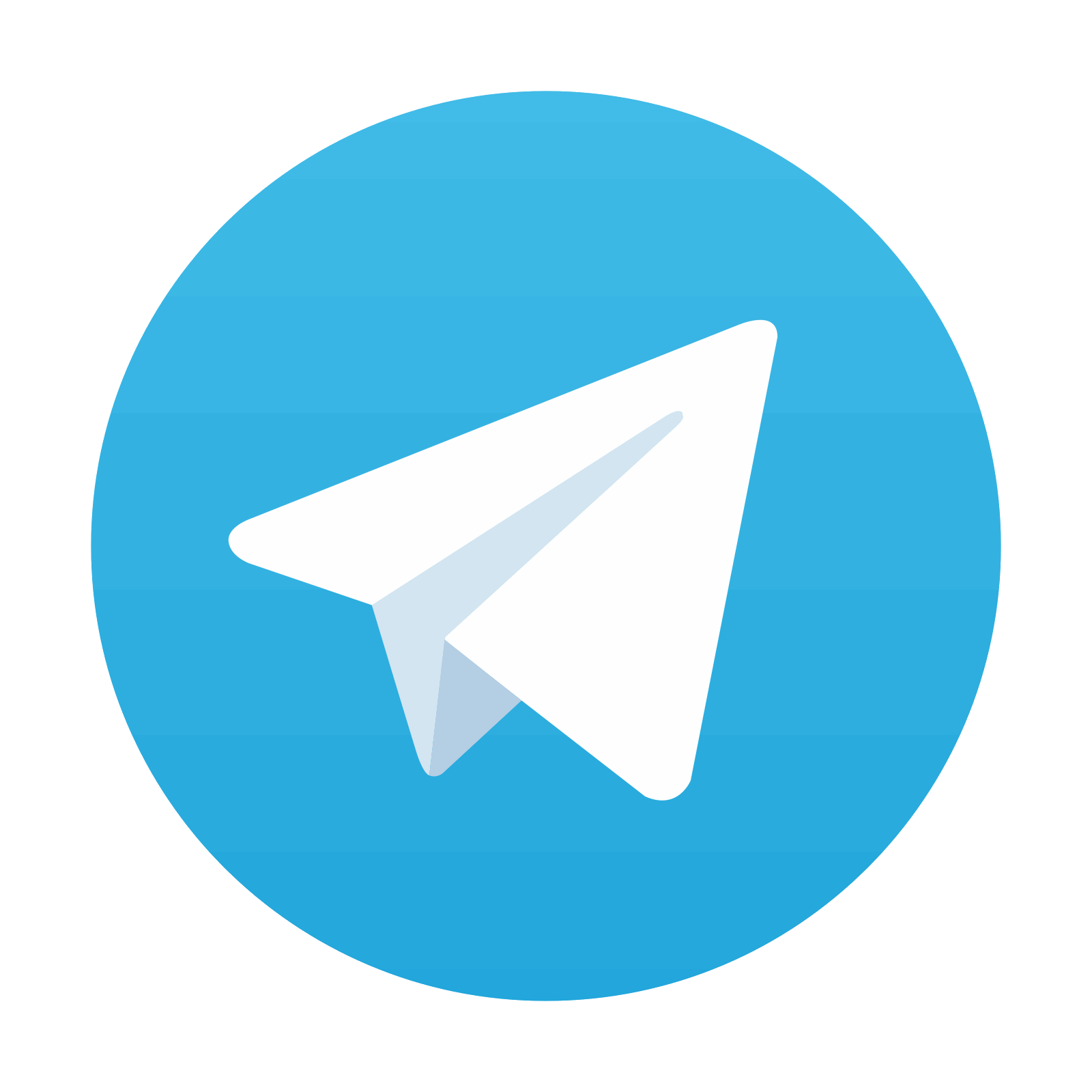
Stay updated, free articles. Join our Telegram channel

Full access? Get Clinical Tree
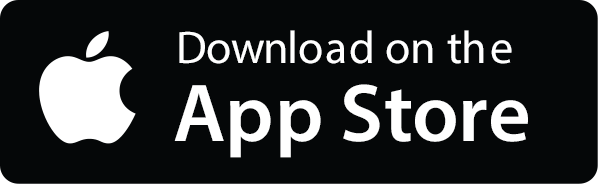
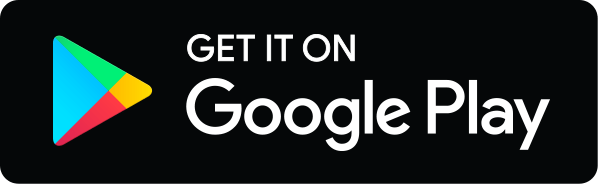
