This article explores the role of PET in the diagnosis and treatment of schizophrenia. PET imaging can reveal neurobiologic aspects such as cerebral blood flow, glucose metabolism, receptor function, and neuroinflammation in schizophrenia. It has supported the dopaminergic hypothesis and helped distinguish symptom types and severity. Diagnostic biomarkers from PET could differentiate schizophrenia from other disorders, while predictive biomarkers might allow earlier targeted treatments. Despite significant promises, the application of PET imaging in schizophrenia is still in its nascent stage, requiring well-designed multicenter studies with large sample sizes to fully realize its clinical potential.
Key points
- •
Reliable clinical tests for the accurate diagnosis of schizophrenia are lacking.
- •
PET imaging has demonstrated the potential to provide valuable insights into schizophrenia.
- •
Diagnostic biomarkers may distinguish schizophrenia from other psychiatric disorders.
- •
PET has shown the potential to differentiate the nature and intensity of symptoms.
- •
Predictive biomarkers may enable the initiation of targeted treatments earlier.
Introduction
Functional neuroimaging can be traced back to the early 1900s, when Walter Dandy, a pioneering American neurosurgeon, introduced pneumoencephalography. This procedure involved the extraction of cerebrospinal fluid (CSF) from around the brain and its subsequent replacement with air through a lumbar puncture, thereby altering the relative density of the brain and its surrounding structures and facilitating their visualization on an X-ray. In a similar vein, Egas Moniz, a renowned neurologist, introduced cerebral angiography in 1927, which allowed for the precise visualization of both normal and pathologic arteries in and around the brain. These seminal contributions represented small yet significant steps toward the eventual advancement of neuroimaging practices.
The emergence of PET as an important functional imaging technique has revolutionized the specialty of nuclear medicine by enabling direct visualization of tissue function through the use of radiotracers. Notably, PET has demonstrated a unique capability to detect aberrant function preceding structural changes observable on computed tomography (CT) scans and/or MR imaging. The selection of the PET radiotracer is determined by the specific biologic processes under investigation. For example, [ 18 F]-fluorodeoxyglucose ([ 18 F]FDG) is commonly employed in studies of cancer and inflammation, [ 18 F]-sodium fluoride ([ 18 F]-NaF) is utilized to investigate bone metabolism, and oxygen-15 water is employed to assess blood flow. Although PET has traditionally been applied in oncology, cardiology, and rheumatology, it has also found increasing utility in neurology and psychiatry. In particular, [ 18 F]FDG PET has been employed in numerous studies of depression, bipolar disorder, schizophrenia, and other neuropsychiatric conditions. Additionally, radiotracers that bind to the receptors of neurotransmitters (such as dopamine and serotonin) have shown potential for the investigation of psychiatric conditions like schizophrenia.
Chronic or recurrent psychoses are a hallmark of schizophrenia, a crippling psychiatric disorder that often shows symptoms in late adolescence or early adulthood, resulting in substantial limitations in one’s ability to function effectively. The diagnosis of schizophrenia requires the presence of at least 2 of the 5 symptoms: delusions, hallucinations, disorganized speech, disorganized or unusual movements, and negative symptoms. The World Health Organization has ranked it as one of the top 10 diseases contributing to the global disease burden, underscoring the need for improved understanding and treatment options. The economic burden of schizophrenia is also staggering, with estimates suggesting an annual cost of $158 billion to the US healthcare system alone. Despite extensive ongoing research, treatment outcomes have been less than optimal, highlighting the urgent need for new approaches.
Quantitative imaging techniques, such as PET, offer a promising avenue for advancing our understanding of the complex pathophysiology underlying the diverse manifestations of schizophrenia. In particular, PET has the potential to provide valuable insights into the biologic basis of schizophrenia, aiding in the development of novel treatment options, and establishing biologic diagnostic criteria. In this educational review, we aim to explore the current applicability of PET for diagnosing and managing schizophrenia, with the ultimate goal of improving clinical outcomes for affected individuals.
A brief history of imaging in psychiatry and schizophrenia
Lassen and Ingvar were pioneers in the field of neuroimaging, introducing a groundbreaking technique for measuring regional cerebral blood flow (rCBF) through the use of Xenon-133, a radioactive isotope of xenon gas. This technique produced accurate 2- and 3-dimensional images of the physiology of the brain, replicating the distribution of neuronal activity during various forms of cerebral activity, including mental activity. Their research paved the way for the potential implementation of neuroimaging in psychiatric diagnoses, particularly in identifying cerebral circulatory abnormalities in organic dementia, a diffuse brain disorder.
Through the use of a multi-detector rCBF technique, Lassen and Ingvar were able to quantify the cerebral repercussions of organic dementia and pinpoint the most affected brain regions to some extent. , Similarly, their studies on patients with schizophrenia revealed significant reductions in frontal rCBF, a finding mainly observable in patients who had negative symptoms, inactivity, autism, disturbed cognition, and speech. They coined this abnormality as ‘hypofrontality’.
The advent of computerized axial tomography scans, combined with the development of radioligands, ushered in a new era in which neuroimaging could aid in identifying mental diseases and elucidating their pathophysiology. Later, in 1976, patients with multi-episode schizophrenia were found to exhibit ventricular enlargement in comparison to the control group of the study, marking a significant breakthrough in understanding the neural basis of mental illnesses.
Principles of PET in brain imaging
Emerging and promising applications of PET increasingly concentrate on developing diagnostic and prognostic biomarkers, which could lead to more accurate diagnoses and treatment response predictions. Often, a fusion of structural and functional imaging procedures, such as PET/CT and PET/MR imaging, characterizing gray and white matter, are used in imaging schizophrenia. Biologic substances labeled with positron emitters like oxygen-15, carbon-11, nitrogen-13, and fluorine-18 are used as radiotracers in the subsequent detection of these materials, allowing for image acquisition. PET imaging can then leverage certain radiotracers (eg, fluorodihydroxyphenylalanine/[ 18 F]-FDOPA, [ 18 F]-setoperone) to investigate the activities of neurotransmitter analogs such as dopamine and serotonin to explore their roles in disease pathogenesis; other PET radiotracers ([ 18 F]FDG, [ 15 O]-H 2 O) can reflect physiologic functions such as metabolism, and blood flow. , ,
[ 18 F]-fluorodeoxyglucose PET in schizophrenia
PET imaging has yielded significant knowledge about the changes in brain function. The introduction of PET in the 1970s, pioneered by David Townsend and Ron Nutt, and the subsequent utilization of [ 18 F]FDG by Alavi and colleagues in 1976, brought about a remarkable transformation in the realm of brain imaging. , The early 1980s saw the widespread use of PET in clinical studies, which led to a flurry of research on schizophrenia using [ 18 F]FDG PET. These studies provided compelling evidence for the theory of hypofrontality, which was further strengthened by subsequent studies that measured brain activity during cognitive tasks. Specifically, PET scans have revealed that increased glucose metabolism in specific brain regions is linked with positive symptoms in schizophrenia.
Conversely, negative symptoms of schizophrenia are correlated with reduced glucose metabolism across all brain regions. , A signed differential mapping investigation using the Montreal Neurologic Institute and Hospital coordinate system (MNI) or Talairach peak coordinates for analyzing subregional alterations revealed areas with significantly lower [ 18 F]FDG uptake in patients with schizophrenia when compared to matched healthy controls. Although it may be central to the etiopathogenesis of schizophrenia, the exact extent and particularity of impaired brain metabolism in relation to the development of schizophrenia remains largely unknown. A recent meta-analysis comprising 1335 subjects from 36 studies reported indications of hypometabolism in the frontal cortex among individuals with schizophrenia. However, there was no consistent evidence of metabolic changes in other brain regions, further supporting the hypofrontality hypothesis in schizophrenia ( Fig. 1 ).
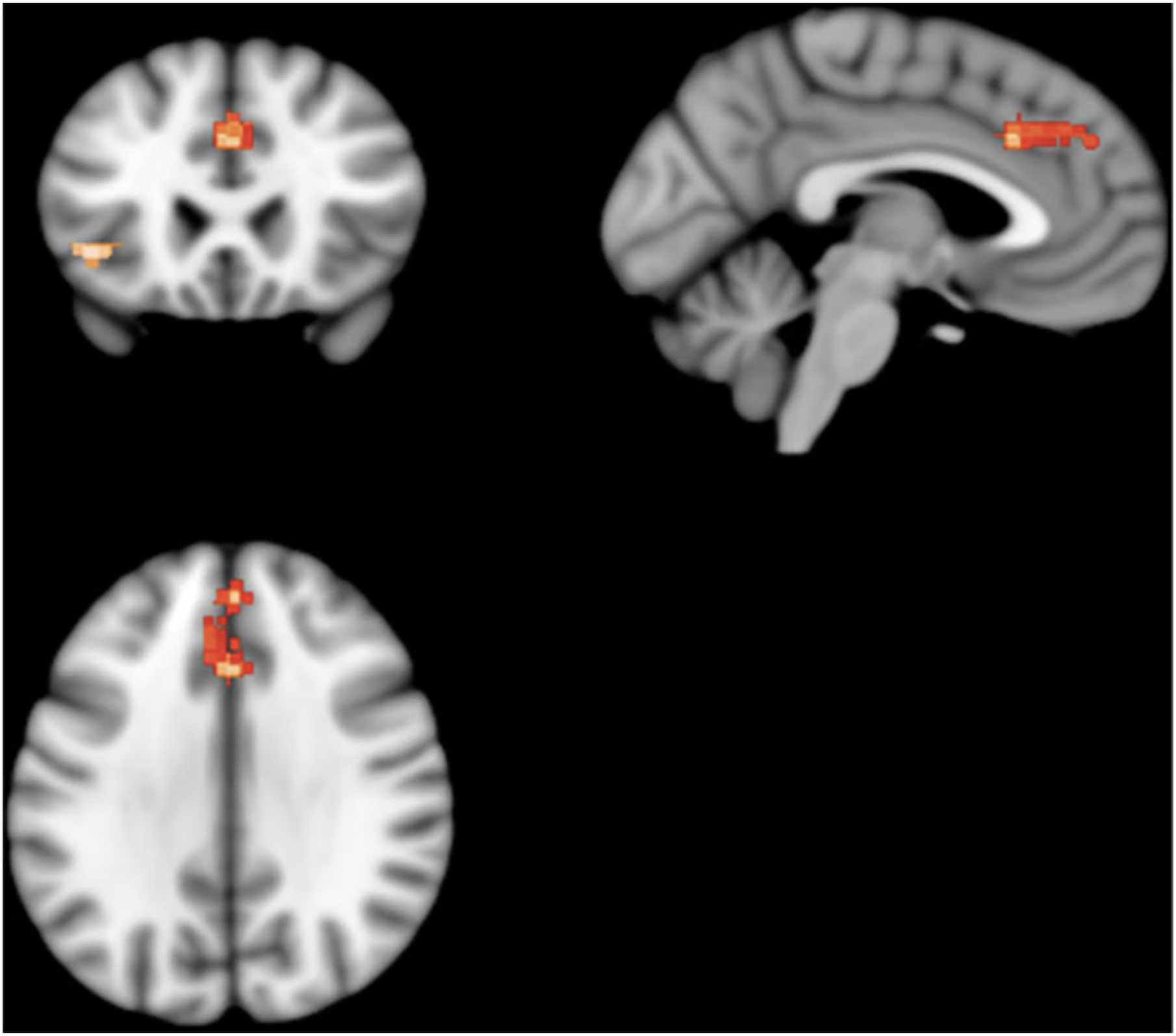
The neuropathology of schizophrenia
Variation in the clinical manifestations of schizophrenia presents difficulty in ascertaining the precise neuropathology involved. Nevertheless, a considerable number of researches has concentrated on abnormalities within the frontal and temporal cortical regions. PET-based studies with [ 18 F]FDG and the dopamine D2 receptors/3R antagonist [ 18 F]-fallypride corroborate the role of dopaminergic anomalies in the disease’s pathogenesis.
Dopamine Hypothesis of Schizophrenia
The classical dopamine hypothesis of schizophrenia is associated with the hyperactivity of dopamine in subcortical regions, such as the striatum, leading to positive symptoms. The effectiveness of antipsychotic medications in treating schizophrenia with PET lends credence to this hypothesis. , Other studies have suggested that elevated norepinephrine and down-regulation of cholinergic activity signaling may also be involved. , Utilizing these and other biomarkers, scientists have attempted to develop analogous radiotracers for PET. Notably, diagnostic and prognostic biomarkers for schizophrenia are most useful if they predate behavioral symptoms so that earlier treatment may prevent or delay onset.
Although the leading hypothesis of schizophrenia involves cortical abnormalities leading to striatal dopamine dysfunction, there is an emerging evidence that the pathophysiology of treatment-responsive schizophrenia (non-TRS) may differ from treatment-resistant schizophrenia (TRS). In a recent study, the correlation between striatal dopamine production and the structural frontostriatal connection was assessed. Dopamine synthesis capacity was assessed using PET with 18 F-6-L-[ 18 F]-FDOPA, while structural connectivity in 2 primary frontostriatal tracts was measured using 3T MR imaging. This evaluation was conducted on 24 individuals diagnosed with schizophrenia and 12 healthy individuals serving as controls. As was predicted, the dorsolateral prefrontal cortex-associative striatum’s connection to the associative striatum’s ability to synthesize dopamine correlated negatively in the non-TRS group but not in the TRS group. Besides extending the dopamine hypothesis of schizophrenia, these findings suggest distinct pathophysiology of TRS and non-TRS.
Adenosine A2A and N-Methyl-D-Aspartate Receptors
Additionally connected to the pathophysiology of schizophrenia are adenosine A2A receptors. Due to the significant presence of adenosine A2A receptors in the basal ganglia system, a region functionally associated with schizophrenia, and previous studies indicating a cross-regulation between adenosine A2A and dopamine D2 receptors in this area, as well as their involvement in dopamine system sensitization, the availability of A2A receptors holds specific importance in the context of schizophrenia. Recently, a study compared 12 male chronic schizophrenics (all on antipsychotic medication and none of whom had motor or extrapyramidal symptoms) to 13 matched healthy controls and measured the availability of the A2A receptor using the PET radiotracer [ 11 C]SCH442416. There were no differences between the 2 groups when A2A receptor binding potential (BPND, a ratio of the measure between specific and non-specific uptake of tracer) was examined in the caudate, putamen, accumbens, and globus pallidus regions of the basal ganglia. Furthermore, [ 11 C]SCH442416 binding and the severity of psychotic symptoms or antipsychotic dosage were also not correlated. Although the evidence from this study was not supportive of the involvement of A2A receptors in the pathogenesis of schizophrenia, future studies with larger sample sizes may provide better insight into the context.
Schizophrenia has also been found to be associated with hippocampal N-Methyl-D-Aspartate Receptors (NMDAR) hypofunction and replay-associated high-frequency ripple oscillation abnormalities in clinical samples and mouse models. A study was conducted using the multimodal human neuroimaging data from 16 patients (7 nonclinical and 9 with schizophrenia) to test the relationship between these 2 hippocampal functions. Each subject underwent a PET scan using [ 18 F]-N-fluoroethyl-labeled GE-179 ([ 18 F]GE-179) to measure the availability of NMDAR, while offline neural replay and high-frequency ripple oscillations were measured using Temporally Delayed Linear Modeling with a magnetoencephalography scan. Hippocampal NMDAR availability was found to positively correlate with replay-associated ripple power in both groups. These findings have significance for the NMDAR hypofunction theory of schizophrenia, as they indicate a connection between the availability of NMDAR in the hippocampus and the replay-associated ripple power.
Neuroinflammation and Translocator-Protein
Psychiatric disorders are generally heterogeneous without consistent neuropathology, which is difficult to capture; however, some consistency exists. The pathophysiology of schizophrenia may be influenced by both immunologic and neuroplasticity-related etiologic variables through the proximal mechanism of microglial activation. It is widely documented that schizophrenia and other related psychotic illnesses are associated with increased activation of microglia and the neuroinflammation that follows. [ 11 C]PBR28 is a marker of microglial activity, and the distribution volume ratio of [ 11 C]PBR28 in the frontal lobe, temporal lobe, and overall gray matter can be significantly different in ultra-high-risk individuals of schizophrenia compared to healthy subjects. Similarly, people with schizophrenia and persistent psychosis, first-episode psychosis, or those who are at heightened risk for acquiring psychosis show enhanced radiotracer binding of the translocator protein (TSPO) radioligand, [ 11 C](R)-PK11195, another tracer that reflects microglial activity. ,
The diverse clinical manifestations of schizophrenia
According to a study, schizophrenic individuals had aberrant brain asymmetry with a preferential decrease in brain volume in the left temporal and right frontal regions. It was also discovered that attention problems in schizophrenia are caused by damage to the medial superior frontal gyrus and the lateral inferior temporal gyrus. Another study found that people with schizophrenia do not activate the anterior cingulate gyrus during selective attention performance.
A study performed to demonstrate memory impairment in schizophrenia during the identification of novel visual stimuli demonstrated reduced activation in the right thalamic region and right prefrontal region. In contrast, left prefrontal cortical activation was increased during impaired episodic recognition of earlier seen visual stimuli. Similarly, another study suggests that memory loss in schizophrenia is mainly caused by frontal lobe failure rather than hippocampal damage. According to a PET study using [ 15 O]water, when the memory load in schizophrenia is raised, the cerebral blood flow rate in the right dorsolateral prefrontal cortex region is dramatically reduced.
Apart from attention and memory, cognition is also impaired in schizophrenia. According to a meta-analysis, schizophrenic patients have a significantly lower availability of thalamic dopamine D2-receptors, resulting in thalamocortical connection breakdown, which may be connected to reduce cognitive function in schizophrenia. Another research conducted on cognitive dysmetria in schizophrenia revealed that people with the condition experienced a lack of activation of the cortical-cerebellar-thalamic-cortical circuitry while recalling familiar or new word lists. Additionally, [ 15 O]water PET imaging demonstrated reduced flow, particularly noticeable in the right anterior cingulate, right thalamus, and both sides of the cerebellum (with the left side showing greater reduction than the right side) compared to individuals without schizophrenia who participated in the novel memory task. Out of 18 patients in a research study with [ 18 F]FDG PET, 4 showed a global drop in metabolism, 5 showed decreased uptake in all regions except occipital, and 6 showed a specific regional decrease in brain glucose metabolism in patients with schizophrenia. The same study showed 8 patients with increased uptake in basal ganglia and 10 patients with reduced cerebellar uptake ( Fig. 2 ).
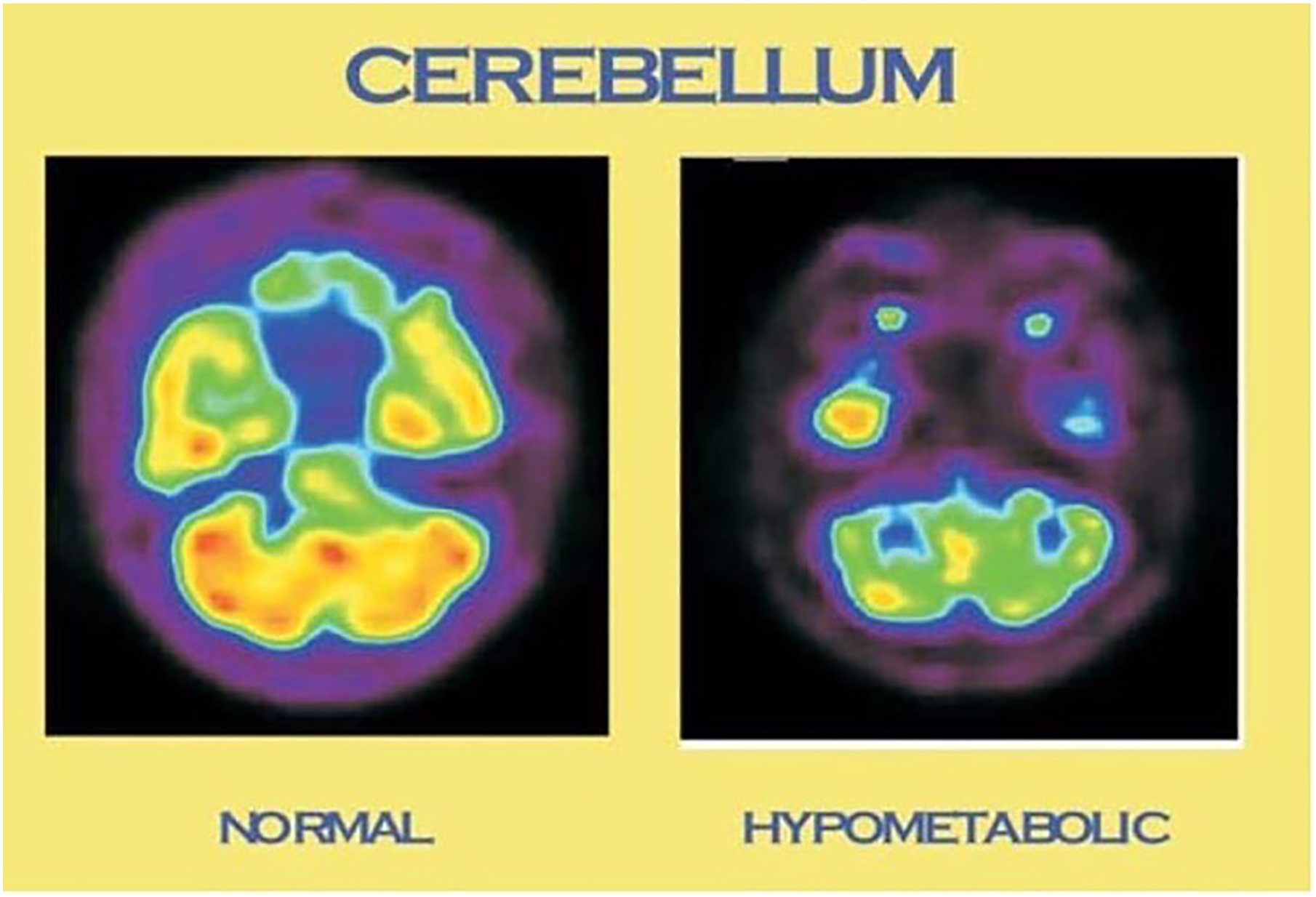
One study focused on schizophrenia participants with poor word generation; PET demonstrated less activation in left hemisphere regions and significant cerebral blood flow alterations as a compensatory functional pattern in negative schizophrenic patients. Regardless of the subtype of schizophrenia, a decrease in parenchymal volume and an increase in CSF volume in the left temporal lobe were related to the severity of negative symptoms. In contrast, right frontal lobe volume reduction was linked to disease duration. Moreover, in schizophrenics with negative symptoms, a strong inverse association between the degree of negative symptoms and [ 18 F]-FDOPA-specific uptake throughout the striatum has been identified and replicated. The specific uptake of [ 18 F]-FDOPA was lower in individuals with a higher preponderance of negative symptoms ( Fig. 3 ).
