Abstract
Objective
As a reversible condition at its early stages, liver fibrosis can progress to cirrhosis and hepatocellular carcinoma, underscoring the importance of early detection for preventing severe outcomes and improving prognosis. To address this issue, we developed a platelet-derived growth factor receptor β (PDGFRβ)-targeted nanoscale phase-change contrast agent to target activated hepatic stellate cells (aHSC) and enable ultrasound imaging as a foundation for the early evaluation of liver fibrosis.
Methods
PDGFR-β antibody-modified phase-change contrast agents (PPCAs) were synthesized utilizing film hydration and ultrasonic emulsification with perfluoropentane (PFP) encapsulated. PPCAs were specifically conjugated to aHSC with high PDGFR-β expression, whose targeting ability was evaluated using fluorescence confocal microscopy and flow cytometry. Phase transition at different temperatures and mechanical indices (MIs), as well as contrast-enhanced ultrasound imaging were analyzed.
Results
PPCAs had an average diameter of 283.6 ± 11.3 nm with good dispersibility and relative stability, and the echo intensity increased correspondingly with increasing MIs. PPCAs exhibited both excellent biocompatibility and imaging ability when excited by high-frequency ultrasound set to an MI of 1.0 at 37°C, and simultaneously showed strong specific targeting ability to aHSC, with cellular uptake reaching 56.67 ± 5.96%.
Conclusion
As a new imaging avenue, PPCAs have the potential to enhance ultrasound imaging and establish the basis for diagnosis by targeting aHSC specifically with good biocompatibility and stability.
Introduction
Liver fibrosis is a chronic disease caused by repetitive liver injury, which can progress to cirrhosis and hepatocellular carcinoma, leading to significant morbidity and mortality worldwide [ ]. The early stages of liver fibrosis are reversible and curable; hence, early detection is essential to prevent the progression of liver fibrosis and improve patient prognosis [ , ]. Although liver biopsy remains the gold standard for assessing liver fibrosis, its associated risks and limitations have prompted extensive research into alternative noninvasive methods for its assessment. Ultrasound elastography and magnetic resonance have demonstrated high accuracy in quantifying liver stiffness, enabling accurate diagnosis of advanced liver fibrosis and cirrhosis [ ]. However, liver stiffness is an indirect measure of fibrosis and can be influenced by factors such as inflammation, hemodynamic changes, and cholestasis [ ]. Therefore, it is imperative to develop no-invasive and reproducible methodologies for early diagnosis of liver fibrosis and monitoring disease progression or regression due to therapeutic interventions.
As reported, molecular targeting imaging technologies such as MRI, SPECT, PET, and US have shown promising potential for visualizing the pathological mechanisms of liver fibrosis [ , ]. However, the clinical application of these technologies is limited by certain drawbacks, including radiation exposure (particularly with SPECT and PET) and high costs. In contrast, ultrasound offers several advantages, including being cost-effective, no-invasive, free from ionizing radiation, and capable of providing real-time imaging [ ]. However, traditional ultrasound microbubble contrast agents still face limitations in molecular targeting imaging due to their relatively large size and lack of stability [ ]. In recent years, phase-change nanoscale droplets equipped with specific targeting antibodies, drugs, or genes have emerged as a novel class of ultrasound contrast agents [ , ]. These nanodroplets undergo phase transitions into gas upon exposure to ultrasound or heat, thereby enhancing ultrasound echo and improving tissue contrast [ ]. However, their excitation phase transition mainly relies on high-intensity focused ultrasound (HIFU) [ , ], near infrared spectrum (NIR) [ ], low intensity focused ultrasound (LIFU) [ ], and other methods [ , ]. These methods may pose risks such as thermal and mechanical effects on normal tissues, high equipment costs, and limited clinical applicability. PFP was identified as ideal ultrasound contrast agent with a low boiling point of approximately 29°C, demonstrating exceptional stability, low toxicity, and biocompatibility [ ]. Under diagnostic ultrasound, PFP can rapidly vaporize to form echogenic microbubbles across a range of MIs and temperatures [ , ]. Additionally, their nanoscale size allows the nanodroplets to take advantage of the enhanced permeability and retention (EPR) effect [ ]. This enables more effective accumulation in liver fibrosis tissues, offering great potential for the early diagnosis of the condition.
Hepatic stellate cells assume a pivotal role in the pathogenesis of liver fibrosis [ ]. They are activated from a quiescent state to an actively proliferating state and are regulated by multiple factors, among which PDGF is particularly important [ ]. PDGFR-β receptors are abundantly expressed in aHSC-T6, making them a highly suitable target for imaging applications [ , ]. Given the central role of PDGFR-β in HSC activation and its potential as an imaging target, it is crucial to develop targeted imaging agents that specifically bind to these receptors.
In this study, we designed and fabricated a novel phase-change contrast agent (PPCA) consisting of liposome-encapsulated PFP, modified with PDGFR-β antibodies to specifically target PDGFR-β receptors in aHSC-T6. This study validated the performance of PPCA in vitro imaging under medical ultrasound excitation and systematically assessed its targeting capabilities and biocompatibility. The findings demonstrated the contrast agent’s favorable safety profile and potential efficacy, highlighting its promise as a strategy for the early diagnosis of liver fibrosis.
Materials and methods
1,2-dipalmitoyl-sn-glycero-3-phosphocholine (DPPC) was obtained from Aldrich Chemical Co. (St. Louis, MO, USA). 1,2-dipalmitoyl-sn-glycerol-3-phospho-lipid ethanolamine-polyethylene glycol-2000 (DPPE-mPEG2000) was acquired from Aladdin Biochemical Technology Co., Ltd. (Shanghai, China). DSPE-PEG2000-biotin and DC-Chol were purchased from Macklin Biochemical Technology Co., Ltd. (Shanghai, China). Biotin labeling kit was obtained from Friendbio Science & Technology Co., Ltd. (Wuhan, China). Perfluoropentane (PFP) was sourced from Acmec Biochemical Co., Ltd. (Shanghai, China). 1′,1-Dioctadecyl-3,3,3′,3′-tetramethylindocarbocyanine perchlorate (DiI) was purchased from MedChenExpress Co., Ltd. (Shanghai, China). FITC-labeled goat anti-rabbit IgG was obtained from Boster Biological Technology Co., Ltd. (Wuhan, China). iFluor TM 594 Conjugated Goat anti-rabbit IgG, PDGF Receptor Beta Monoclonal Antibody, α-SMA Monoclonal Antibody, and GAPDH were purchased from HuaAn Biotechnology Co., Ltd. (Hangzhou, China). TGF-β1 was purchased from Abbkine Scientific Co., Ltd. (Wuhan, China). CCK-8 assays were purchased from Beyotime Biotechnology Co., Ltd. (Shanghai, China). DMEM and FBS were purchased from Gibco (Thermo Fisher, USA). 4′,6-diamidino-2-phenylindole (DAPI) was purchased from Solarbio Science & Technology Co., Ltd. (Beijing, China). RIPA buffer and SDS-PAGE were purchased from Epizyme Biomedical Technology Co., Ltd. (Shanghai, China). HUVEC cells obtained from the North Sichuan Medical College Innovation Centre for Science and Technology. Rat HSC-T6 cells were purchased from Pricella Biotechnology Co., Ltd. (Wuhan, China). Trypan Blue Staining solution was purchased from Biosharp Co., Ltd. (Shanghai, China).
Preparation of PPCA
The phase-transitional contrast agent (PCA) was prepared according to a previously described methods [ , ]. Briefly, DPPC, DPPE-mPEG2000, DSPE-PEG2000-biotin, and cholesterol were dissolved in 5 mL of anhydrous ethanol at a mass ratio of 5:2:1:0.5 and thoroughly mixed using a vortex mixer. The resulting solution was transferred to a round-bottom flask and rotary-evaporated for 30 minutes at 40°C. Subsequently, 5 mL of PBS solution was added for hydration. The solution was dispersed by ultrasonication (3 s on, 2 s off, 200 W) for 5 minutes, followed by centrifugation (12,000 rpm, 4°C, 5 min). The supernatant was removed and the pellet was resuspended in 4 mL of PBS. The liposome solution (1 mL) was subsequently chilled on ice, followed by the addition of PFP (100 μL). Emulsification was achieved through sonication in an ice bath for 60 seconds using a cycle of 3 seconds on and 2 seconds off at a power output of 200 W. After centrifugation (12,000 rpm, 4°C, 5 min), the supernatant was carefully discarded, and the pellet underwent three washes with PBS to eliminate any residual free liposomes and unencapsulated PFP.
To obtain PPCAs, 1 mL of PFP liposome suspension was incubated with 30 µL of streptavidin for 20 minutes at room temperature, followed by washing with PBS via centrifugation at 8,000 rpm for 30 minutes. Subsequently, 10 µL of biotinylated labeled antibody was added, mixed, then incubated at 4°C for 2 h and washed with PBS at 8000 rpm for 3 minutes to remove free antibodies. Fluorescently labeled nanodroplets were prepared by adding DiI (0.2 mg per 10 mg of liposomes) fluorescent dye to the lipid solution under light-protection conditions. FITC-IgG was introduced to label the PDGFRβ antibody attached to the liposome, and the mixture was washed with PBS three times at a speed of 12,000 rpm. The morphology of the PPCA was observed using transmission electron microscopy (TEM, HT7700, Hitachi, Tokyo, Japan). Particle size and zeta potential analyses were performed using a laser particle size analyzer and zeta potential analyzer, respectively, on PPCA samples. Subsequently, changes in particle size of PPCA were monitored over a specified period.
PPCA nanodroplet distribution in fibrotic liver
Following fibrosis induction, DiI-labeled PPCA nanodroplets (2.5 mg/kg) were administered. Thirty minutes postinjection, the rats were euthanized, and liver tissues were collected, fixed in 4% paraformaldehyde, and processed into frozen sections. The sections were stained with DAPI and imaged using confocal microscopy for fluorescence visualization of the contrast agent. This allowed for a detailed assessment of nanodroplet distribution within the fibrotic liver tissue.
Ultrasound imaging
The contrast-enhanced ultrasound imaging (CEUI) capability of PPCA was assessed using an ultrasound scanner (LOGIQ E11, GE, USA) equipped with a linear array probe L6-24 operating at a frequency of 18 MHz, with a focus set at 1.5 cm and depth of 2.5 cm, and a dynamic range of 63 dB. The amplitude modulation (AM) contrast mode was utilized for imaging. For in vitro ultrasound imaging, PPCAs and a deionized water solution were loaded into a 3 mL pasteurized pipette and immersed in deionized water at 37°C, followed by ultrasonic exposure at varying mechanical indices (MI: 0.2, 0.4, 0.8, 1.0, and 1.2). To further investigate the temperature-dependent phase transition of PPCA, samples were placed in a water tank set to 22°C, 37°C, and 50°C, and observed for 20 minutes using the LOGIQ E11 ultrasonic imager.
Activation of the HSC-T6 strain
Rat HSC-T6 cells were cultured at 37°C in 5% carbon dioxide in medium supplemented with 5% heat-inactivated fetal bovine serum (FBS) and 1% penicillin/streptomycin. HSC-T6 were activated into aHSC-T6 by treating them with different concentrations of TGF-β1 (0, 1, 2.5, 5, and 10 ng/mL) and at varying stimulation times (0, 6, 12, and 24 h) to identify the optimal conditions for activation.
Western blotting
To measure the expression of potential biomarkers, total proteins of HSCs were extracted using radioimmunoprecipitation assay (RIPA) lysis buffer and separated by sodium dodecyl sulfate–polyacrylamide gel electrophoresis (SDS‒PAGE). After transfer to a polyvinylidene difuoride (PVDF) membrane, western blotting was performed using antibodies against biomarkers and their corresponding secondary antibodies. Glyceraldehyde-3-phosphate dehydrogenase (GAPDH) served as a loading control.
Immunofluorescent staining
HSC-T6 cells were stimulated with 5 ng/ml of TGF-β1 for 12 h, followed by detection of α-SMA expression by immunofluorescence cytochemistry. The cells were fixed with 4% paraformaldehyde for 10 min, treated with 0.1% Triton X-100 for 20 min and blocked with 1% BSA for 40 min at room temperature. Subsequently, cells were incubated with primary antibodies against PDGFR-β and α-SMA, respectively, at 4°C overnight. After incubation with fluorescent secondary antibodies (FITC-IgG) and iFluor TM 594 IgG respectively for 1 h at room temperature, then incubated with 40,6-diamino-2-phenylindole (DAPI) for 10 min before being photographed by confocal fluorescence microscopy.
Cell targeting efficiency and flow cytometry
aHSC-T6 in a temperature incubator of 37°C with volume fraction of 5% CO 2 , after inoculation in laser confocal dishes, aHSC-T6 cells were incubated with DiI-labeled PPCAs or DiI-labeled PCAs. After six hour, the cells were gently washed three times. The targeting effect was assessed using fluorescence microscopy (Olympus). In addition, the cellular uptake of PPCAs was quantitatively analyzed by flow cytometry. Excitations: 549 nm for DiI and emissions: 565 nm for DiI.
In vitro cytotoxicity
To evaluate the phase transition of nanodroplets and their potential cytotoxicity, a series of experiments were conducted. HSC-T6 cells were seeded into 96-well plates and allowed to adhere. These cells were then exposed to medical sonication, either alone or in combination with nanodroplets (0.25 mg/ml), for treatment durations of 10, 30, 60, 90, and 120 seconds. Following ultrasound exposure, the cells were examined and visualized using a light microscope. Trypan blue staining was performed to assess cell membrane integrity. Additionally, the CCK-8 assay was employed to quantitatively assess cell viability post-treatment.
HSC-T6 or HUVEC cells were seeded into 96-well plates and incubated overnight to allow for proper adhesion. After incubation, the culture medium was replaced with fresh medium containing different concentrations of the nanotargeted contrast agents. Following 24 h of treatment, the medium was removed, and cell viability was assessed using the CCK-8 assay.
Hemolysis assay
In this study, hemolysis of mouse blood was evaluated by observing PPCA at different concentrations. Each sample was mixed with hematocrit suspension solution and placed on a shaker at 37°C for 1 h. After incubation, samples were centrifuged, and photographs were taken for comparison.
To further investigate the hemolytic potential of medical ultrasound alone and the impact of nanodroplet phase transition on hemolysis, additional experiments were conducted. Erythrocyte suspensions and erythrocyte mixtures with PPCA (0.25 mg/ml) were subjected to a diagnostic ultrasound device at 37°C. Ultrasound was administered for 10, 30, 60, 90, and 120 seconds, respectively. Following ultrasound treatment, the samples were centrifuged. The optical density (OD) of the supernatant was measured at 545 nm using a spectrophotometer, and the hemolysis rate was calculated using the following formula: Hemolysis rate (%) = ([OD sample – OD negative] / [OD positive – OD negative]) × 100%.
Statistical analysis
Statistical analyses were performed using GraphPad Prism 8 software. Measurement data that followed a normal distribution were expressed as mean ± standard deviation (x ± s). Comparisons between two groups were conducted using an unpaired t-test, while comparisons among multiple groups were performed using one-way or multifactorial ANOVA. A p -value of less than 0.05 was considered indicative of a statistically significant difference. The levels of statistical significance were defined as follows: * p < 0.05, ** p < 0.01, and *** p < 0.001, with “ns” indicating no significant difference.
Results
Characterization of physical and chemical properties of PPCA
PPCA displayed a spherical shape with uniform size and a monodisperse state in transmission electron microscopy (TEM) imaging ( Fig. 1 a). The average particle size of PPCA in deionized water at room temperature was measured using a laser particle sizing instrument as 283.6 ± 11.3 nm ( Fig. 1 a). The surface potential of simple liposomes was positive at -2.25 ± 0.78 mV, which increased to 24.9 ± 0.52 mV upon encapsulation with PFP nanodroplets, and further to 12.16 ± 0.55 mV after antibody attachment using the affinity streptavidin method ( Fig. 1 b). The contrast agent solution appeared as a milky white liquid and remained stable upon continuous observation for 72 h; slight delamination was observed after two days, which reverted to a milky white suspension upon slight shaking ( Fig. 1 c, d). Confocal laser scanning microscopy (CLSM) images demonstrated significant overlap between DiI-labeled liposomes (red) and FITC-labeled antibodies (green), resulting in bright yellow overlapping areas ( Fig. 1 e). These experiments collectively confirm the successful synthesis of PPCA ultrasound contrast agent, demonstrating good stability and effective antibody binding.
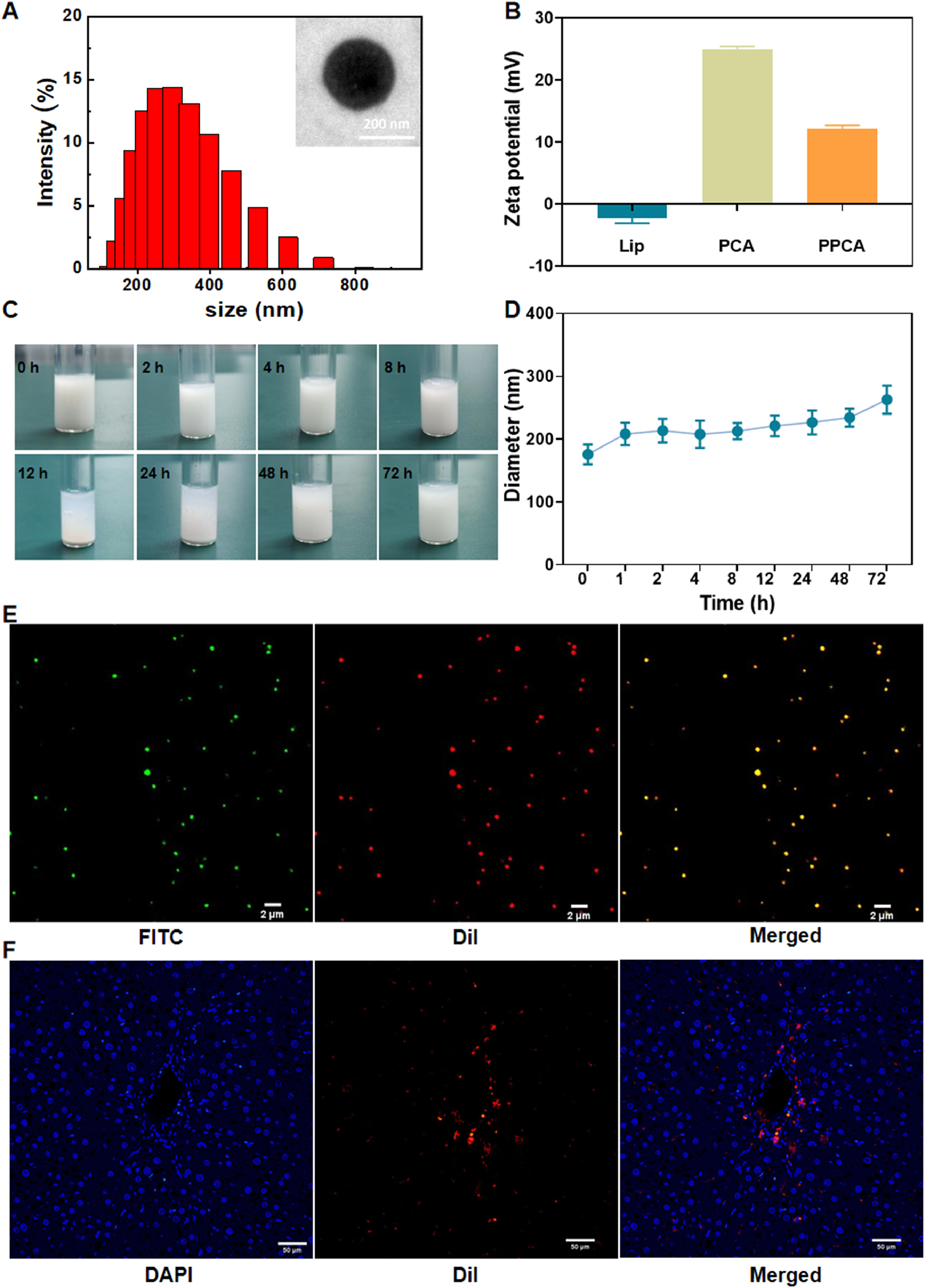
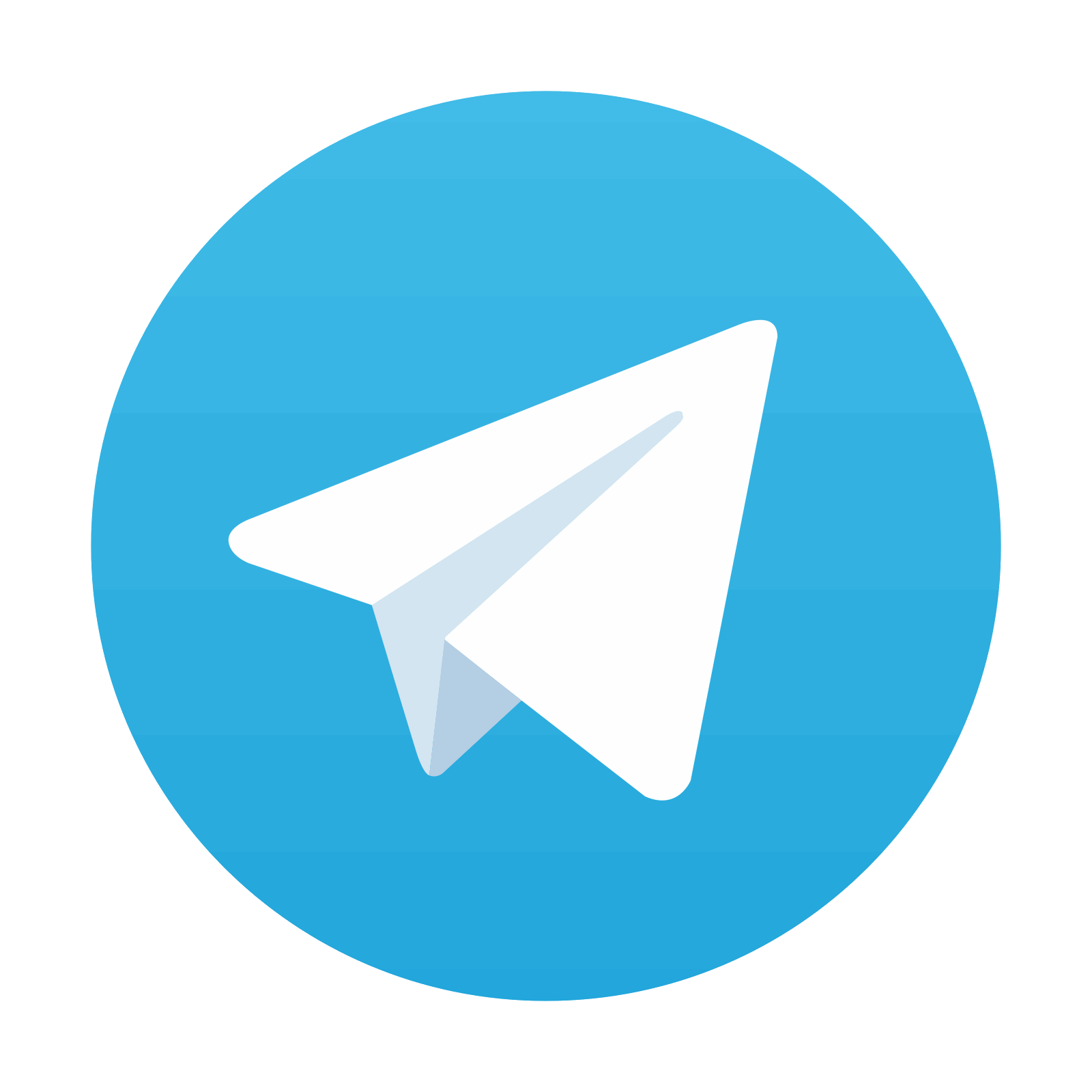
Stay updated, free articles. Join our Telegram channel

Full access? Get Clinical Tree
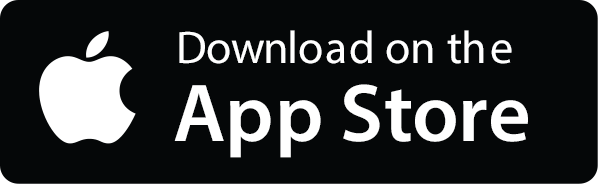
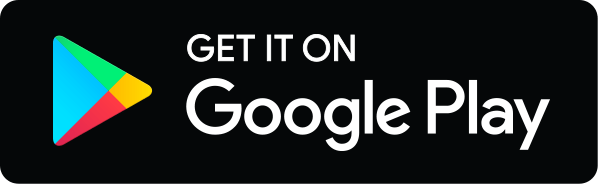
