A Pathologist’s Overview of the Pathology of Breast Disease
Joseph Rabban
In general, pathologists classify breast lesions according to the microscopic architecture and cytologic features of the cells that compose the lesion. Diagnostic breast pathology is complex, and a detailed discussion is beyond the scope of this text. Excellent reference sources are widely available, including the recently issued 2003 version of the World Health Organization Classification of Tumors (1,2,3,4,5,6). The goals of this section, which draws from these sources, are (a) to introduce the process of preparing core needle biopsy samples for microscopic evaluation and ways in which radiologists may help optimize this process, (b) to broadly introduce the general classification of common breast pathology seen in routine practice, and (c) to familiarize the radiologist with the terminology and structure of the pathology report of a typical breast core biopsy.
Tissue Processing
To microscopically visualize the tissue that is obtained by breast core biopsy or surgical excision, the tissue must be cut into a sheet approximately 5 μm in thickness, and this sheet must be mounted on a glass slide. Multiple steps are involved in preparing such slides from the freshly biopsied tissue, and, in most laboratories, two days are required (i.e., biopsies are submitted on one day, and slides are available the next day). These steps include fixing the tissue; processing the tissue; embedding the tissue in wax (paraffin) for sectioning; sectioning the tissue; mounting the sections on the glass slides; and staining the sections.
Tissue fixation refers to exposing the tissue to chemicals that harden the tissue and preserve the three-dimensional structure of the cells and extracellular stromal components. In the United States, commonly used fixatives are formaldehyde-based. Penetration of formaldehyde into the tissues occurs at a rate of approximately 1 mm per hour, so most small biopsies require at least several hours of fixation. Tissue that is suboptimally fixed (i.e., exposed to fixative for too short a duration) or not quickly placed into fixative will degenerate and can be uninterpretable under the microscope. The radiologist can help to achieve optimal fixation by immediately and directly placing biopsy material (Fig. 2B-1) into containers with adequate volume of fixative solution prior to submitting them to the pathology laboratory. A volume ratio of fixative to tissue of 10:1 is commonly recommended; basically, the more fixative, the better. Wrapping tissue biopsy cores in gauze or other material is not advised because this may lead to damage or fragmentation of the cores when the pathologist or technician unwraps the gauze to evaluate the specimen. The cores may stick to the gauze or even get lost in the material. Placing cores directly in the fixative solution is best. Of note, saline
is not a fixative and may cause artifacts in the tissue. If a delay is anticipated before fixation, tissue can be refrigerated in an airtight container, although this is not advisable for periods of more than several hours.
is not a fixative and may cause artifacts in the tissue. If a delay is anticipated before fixation, tissue can be refrigerated in an airtight container, although this is not advisable for periods of more than several hours.
Tissue dehydration is an automated process in which the fixed tissue is exposed to sequential dilutions of alcohol-based solutions to remove water and allow the tissue to be embedded in paraffin wax. The biopsy cores (Fig. 2B-1) are first loaded into plastic cassettes. A cassette is a small container, approximately 3 cm by 2 cm by 0.3 cm, with perforated sides to allow the solutions to pass freely and bathe the tissue (Fig. 2B-2). Hundreds of cassettes can be loaded into an automated tissue processor which can run through a programmed series of various dehydration solutions. The process generally runs overnight in most pathology laboratories.
Tissue sectioning is accomplished by embedding the biopsy cores in a block of paraffin wax. The paraffin block containing the tissue is then sequentially shaved down using a blade mounted in a microtome. The microtome allows for precisely shaved sections to be cut at a thickness of only 2 to 10 μm. Generally, 5 μm is the optimal thickness for visualizing cytoplasmic and nuclear detail with routine stains. These paper-thin sections are then placed onto a glass slide and allowed to dry (Fig. 2B-3).
Processing of excisional biopsies is essentially the same as that for core biopsies except that the larger size of the tissue requires the pathologist to dissect the tissue and sample areas of interest (Fig. 2B-4). Detailed discussion of the
handling of excisional specimens is beyond the scope of this section because the approach depends on the clinical scenario. It is now generally accepted that an excisional biopsy of breast tissue should have its surfaces painted with “ink” before it is cut into sections for review. The goal of the surgical resection of potential breast cancer is to remove the lesion with a rim of normal breast tissue all the way around. Once the surface of the excised tissue has been inked, the pathologist can determine whether neoplastic cells are at or near the margin of resection by measuring the microscopic distance between the tumor and the ink. If tumor comes to the resection margin (the inked surface), the obvious inference is that there is tumor on the other side of this margin (still in the breast). Although it cannot always be achieved, the ideal goal of surgery is to remove a cancer with “clear margins.” The exact definition of clear margins is controversial, varying between 0.1 and 1 cm depending on the lesion and the clinician. In any case, the pathologist should ink the surfaces of any specimen other than a core biopsy.
handling of excisional specimens is beyond the scope of this section because the approach depends on the clinical scenario. It is now generally accepted that an excisional biopsy of breast tissue should have its surfaces painted with “ink” before it is cut into sections for review. The goal of the surgical resection of potential breast cancer is to remove the lesion with a rim of normal breast tissue all the way around. Once the surface of the excised tissue has been inked, the pathologist can determine whether neoplastic cells are at or near the margin of resection by measuring the microscopic distance between the tumor and the ink. If tumor comes to the resection margin (the inked surface), the obvious inference is that there is tumor on the other side of this margin (still in the breast). Although it cannot always be achieved, the ideal goal of surgery is to remove a cancer with “clear margins.” The exact definition of clear margins is controversial, varying between 0.1 and 1 cm depending on the lesion and the clinician. In any case, the pathologist should ink the surfaces of any specimen other than a core biopsy.
The surgeon may decide to orient an excisional specimen in three dimensions, thereby allowing the pathologist to define six margins (anterior, posterior, superior, inferior, medial, and lateral). The pathologist can preserve the orientation in the sectioned tissue by inking the margins with different color inks. One way that the surgeon can orient the excised specimen is by affixing sutures of different length to the particular tissue margins. If the specimen is not oriented, the specimen should still be inked by the pathologist, but, if tumor cells are seen microscopically at an inked margin, it will not be possible for the pathologist to determine which margin is involved and hence what portion of the resection cavity might harbor residual tumor.
After inking the specimen is sliced into parallel sections, each ideally 3 mm thick. Any grossly visible abnormality in the tissue slices can then be further trimmed to be loaded into cassettes for processing. The algorithm for deciding how much and which areas of tissue to sample is complex and depends on the size of the specimen, the presence of grossly visible abnormalities, and the clinical context (i.e., mammographic calcifications, palpable mass, nonpalpable mass, prior cancer, etc.). There is always the possibility of a sampling error when tissues are examined microscopically. The process is designed to thoroughly sample tissues, but even pathological evaluation is a sampling. The pathologist cannot possibly review all of the excised tissue in a given case. For example, it would require approximately 2,000 slides to completely review a very small 1 cc sample of tissue using serial sections that are only microns thick. This is clearly impractical. Consequently, pathologists review several (hopefully) representative slides from each subsample of the tissue. When large amounts of tissue are removed, it may not be practical to even process all of the tissue so that it is critical to provide the pathologist with sufficient clinical details so that the correct sampling can be performed efficiently.
When core biopsies are performed to determine the cause of mammographically detected calcifications, it is helpful if the radiologist can separate the tissue cores containing calcifications (proved by specimen radiography) into a separately labeled container from the cores that do not contain calcifications. Although all of the tissue will be processed and examined microscopically, sometimes calcifications are not present in the initial slides. Knowing which cores contain the calcifications will allow the pathologist to be more efficient in the search for the calcifications; this will be discussed in detail later in this chapter.
Tissue staining is required to visualize the cells once the tissue section is mounted on the glass slide. The most commonly used stain is a combination of hematoxylin and eosin, referred to as H&E stain. Hematoxylin is a basic dye and therefore has affinity for nucleic acids. Hematoxylin will stain the cell nucleus dark blue or purple. Eosin is an acidic dye and stains the cell cytoplasm and stroma pink.
The H&E stain itself does not differentiate cell types (e.g., epithelial cell versus fibroblast); the nucleus of an epithelial cell is basophilic (blue) just as is the nucleus of a myoepithelial cell. In certain circumstances, it may be necessary for the pathologist to confirm the cell type present, for example, myoepithelium or epithelium. Since the myoepithelial cell layer is in the outer wall of the duct, ductal carcinoma in situ (DCIS) is distinguished from invasive ductal carcinoma by the presence of a myoepithelial cell layer surrounding the tumor proliferation. To confirm the presence of myoepithelium, immunohistochemical stains can be performed to detect protein antigens that are expressed by myoepithelium but not by epithelium.
Immunohistochemical Stains
Immunohistochemistry (IHC) uses antibodies directed against the antigen of interest. These antibodies are linked to chemical complexes that can react, for example, in a colorimetric enzymatic reaction that ultimately can be seen under the microscope. The antibody binds to the antigen of the cell, and the chemical complex that is bound to the antibody can be processed so that the pathologist sees the color decorating that cell under the microscope. Immunohistochemical evaluation may be helpful in various settings. Common examples include (a) distinguishing ductal differentiation versus lobular differentiation in a carcinoma by evaluating E-cadherin expression (lobular carcinoma does not express E-cadherin); (b) distinguishing in situ versus invasive carcinoma (myoepithelium is preserved around in situ tumor, therefore expression of myoepithelial antigens, such as calponin, myosin heavy chain, or p63 excludes invasion); and (c) evaluating potential response to tamoxifen by determining estrogen receptor expression in the tumor cell nuclei. Generally, routine processing of tissue for H&E staining is an overnight process from the time the tissue arrives at the pathology laboratory to the
time the glass slide is stained and available. Immunohistochemical staining may take an additional 1 to 2 days.
time the glass slide is stained and available. Immunohistochemical staining may take an additional 1 to 2 days.
Pathologic Categorization of Breast Disease
The pathologic categorization of breast disease is based on a combination of the architecture, cytologic features, and cell type of the lesion. As described in the sections on the normal anatomy of the breast, the basic cell types are the epithelial lining of the ducts and lobules, the myoepithelium surrounding the ducts and lobules, the fibroblasts of the stroma, stromal blood vessels and nerves, and the adipocytes, fat cells that make up the bulk of the breast tissue. In addition, reactive or inflammatory processes may recruit histiocytes (tissue macrophages) and inflammatory cells to the breast. Broadly speaking, each of these normal cell types can give rise to pathology, in addition to ectopic and metastatic entities. It is important to acknowledge, however, that the histogenesis of some pathologic entities is as yet undefined, and pathologists may sometimes attribute a particular lesion to a corresponding cell type merely out of its visual similarity rather than out of established biologic evidence. The following is a summary of the more commonly encountered breast lesions. The World Health Organization published its most currently revised classification of breast neoplasms in 2003 (1), and it includes the more unusual subtypes of lesions that will not be addressed here.
Normal Histology
The basic microscopic unit of the breast is the terminal duct lobular unit (TDLU). The bulk of the breast parenchyma consists of adipose (fat tissue) and fibrous stroma within which are embedded the TDLUs (Fig. 2B-5). Each TDLU consists of a round aggregate of tubular glands called acini that are arranged around a terminal duct (Figs. 2B-6,2B-7,2B-8). Functionally, during lactation, each acinus will secrete milk into the terminal duct of that lobule (Fig. 2B-9). The terminal ducts will then sequentially converge on larger ducts (Fig. 2B-10) that ultimately empty into the lactiferous sinus (Fig. 2B-11). The lactiferous ducts (Fig. 2B-12) then communicate directly with the nipple (Fig. 2B-13) to form the channels through which milk is expelled. Smooth muscle lining surrounding the lactiferous ducts aids in this process.
![]() Figure 2B-7 Normal acinus. Two cell layers compose the normal acinus, an outer myoepithelial cell layer and an inner glandular epithelial cell layer. |
![]() Figure 2B-9 Lactation. The acini are hyperplasic and dilated from secreting milk globules (white round globules) into the lumens. |
![]() Figure 2B-10 Normal segmental duct. The lining consists of an inner epithelial cell layer and an outer myoepithelial layer, similar to that of the acinus. |
![]() Figure 2B-13 Normal nipple. Lactiferous ducts drain directly to the nipple via multiple channels through the skin. |
![]() Figure 2B-14 Normal myoepithelium. Immunohistochemical stains for smooth muscle antigens highlight the myoepithelial layer of these acini while the inner epithelial layer is unstained. |
The cellular composition is essentially the same from the acinus to the lactiferous duct. It is a dual population lining, consisting of a single layer of inner ductal epithelium that lines the acini and ducts and an outer myoepithelial layer. The acinar epithelium is usually cuboidal in shape in the TDLU (Fig. 2B-7). The cytoplasm is clear to eosinophilic, and the nuclei are bland. The myoepithelium is usually cuboidal in shape and can be immunohistochemically distinguished from the epithelium by its expression of myoepithelial antigens (Fig. 2B-14). During the luteal phase of the menstrual cycle, myoepithelial cells may exhibit more abundant, clear cytoplasm, and prominent nucleoli may be seen in the epithelium (Fig. 2B-15). During lactation, large vacuoles of milk form within the acini and are expelled into the lumen (Fig. 2B-9). Once breastfeeding has stopped, the lobules gradually involute over time and return to their normal appearance; involuting post-lactation changes can be seen during this process, including variably dilated acini, residual secretions, and attenuated epithelial lining (Fig. 2B-16).
As mentioned earlier, breast parenchyma contains abundant fibrous stroma, referred to as nonspecialized stroma in contrast to the specialized stroma within the TDLU. The cells include predominantly adipocytes (fat cells) and fibroblasts, with small blood vessels and occasional peripheral nerves (Figs. 2B-17 and 2B-18). Although there is no formal
boundary or capsule defining the TDLU, it maintains a well-defined lobular architecture. In between the acini within the TDLU is specialized fibrous stroma.
boundary or capsule defining the TDLU, it maintains a well-defined lobular architecture. In between the acini within the TDLU is specialized fibrous stroma.
![]() Figure 2B-18 Normal peripheral nerves. These well-circumscribed bundles of wavy spindle cells are small peripheral nerves. A small vein is positioned between them. |
Atrophy of the TDLU can occur in normal postmenopausal aging as well as following radiation or chemotherapy exposure. Atrophic TDLUs show decreased size and number of acini, fibrosis of the basement membrane surrounding the acini, and fibrosis of the specialized stroma (Fig. 2B-19). Additional findings following exposure to radiation or chemotherapy include atypical epithelial changes, such as prominent nucleoli and increased nuclear size (Figs. 2B-20 and 2B-21).
Male gynecomastia consists of duct proliferation with variable fibrosis and inflammation. Lobules are absent. Variable degrees of ductal proliferation may be seen at different stages of gynecomastia (Figs. 2B-22 and 2B-23).
Inflammatory Lesions
Healing of Biopsy Site
Following a core biopsy or excisional biopsy, the wound healing process in the breast begins immediately and evolves over several weeks. This evolution can be witnessed in the histologic findings at the biopsy site. Initially, red blood cells and fibrin fill the space created by the wound (Fig. 2B-24). Within the first few days, histiocytes (tissue macrophages) aggregate at the site along with inflammatory cells. Multinucleated giant histiocytes may also be present. Granulation tissue consists of neovascularization and collagen deposition, manifested by an abundance of small blood vessels, fibroblasts, and collagen (Fig. 2B-25). Over the ensuing weeks, the number of histiocytes diminishes, although residual histiocytes laden with hemosiderin (breakdown products of hemoglobin) are a telltale sign of
prior bleeding. Over these weeks, fibroblasts and collagen fill the space, creating the scar (Fig. 2B-26). Residual changes of a healing biopsy site are often not detectable after several months following biopsy or excision. Clips placed at the time of the core biopsy become embedded in a collagen matrix consisting of bovine collagen. This can be seen microscopically (Fig. 2B-27). Usually, this material is not resorbed or broken down by histiocytes, and it often elicits a robust multinucleated giant cell histiocytic response. Suture material from a prior surgical procedure also elicits a similar response (Fig. 2B-28).
prior bleeding. Over these weeks, fibroblasts and collagen fill the space, creating the scar (Fig. 2B-26). Residual changes of a healing biopsy site are often not detectable after several months following biopsy or excision. Clips placed at the time of the core biopsy become embedded in a collagen matrix consisting of bovine collagen. This can be seen microscopically (Fig. 2B-27). Usually, this material is not resorbed or broken down by histiocytes, and it often elicits a robust multinucleated giant cell histiocytic response. Suture material from a prior surgical procedure also elicits a similar response (Fig. 2B-28).
Subareolar Abscess
Duct obstruction during lactation can lead to rupture, resulting in inflammation and abscess formation. This process is usually subareolar and consists of acute inflammatory cells and necrotic debris accumulating within and around the duct. Because the abscess is based within a duct,
there is usually a distinct finding of a reactive metaplastic change of the normally columnar cell duct epithelial lining to a stratified squamous epithelial duct lining. Exposure of the metaplastic squamous cells to the surrounding stroma elicits a foreign-body type reaction, and multinucleated giant histiocytes may aggregate at the abscess site.
there is usually a distinct finding of a reactive metaplastic change of the normally columnar cell duct epithelial lining to a stratified squamous epithelial duct lining. Exposure of the metaplastic squamous cells to the surrounding stroma elicits a foreign-body type reaction, and multinucleated giant histiocytes may aggregate at the abscess site.
![]() Figure 2B-26 Scar. This well-healed site of a prior core biopsy shows spindle shaped fibroblasts arranged in bundles with abundant extracellular collagen deposition. |
Mammary Duct Ectasia
The etiology of duct ectasia is unclear. Typically, duct ectasia affects middle- to older-aged patients. It is not uncommon to see large ducts by mammography or ultrasound. Duct ectasia may present clinically by nipple discharge or bleeding. Microscopically, the central, larger ducts are involved by dilatation (ectasia) and are filled with a granular, proteinaceous material (Fig. 2B-29). Foamy histiocytes, so-called because of the foamy intracytoplastic accumulation of lipid breakdown products, are present in the duct lumen and along the duct wall. Squamous metaplasia of the duct lining, as seen in subareolar abscess, may be present. Chronic inflammatory cells and histiocytes aggregate in the periductal stroma. If the duct ruptures, a granulomatous multinucleate giant cell histiocytic response may ensue (Fig. 2B-30). Over time, the inflammation resolves and periductal stromal fibrosis becomes prominent.
Diabetic Mastopathy
Diabetic mastopathy is a stromal proliferation associated with perivascular lymphocytic collections (Fig. 2B-31) that can form a mass within the breast of diabetic patients, including male diabetics. Although this is quite uncommon, the constellation of pathologic findings is distinct. The stroma is markedly collagenized (scarlike) (Fig. 2B-32) and there is increased stromal cellularity, consisting of spindle cells. This fibrous stromal proliferation can produce a firm mass measuring up to several centimeters. The second component of diabetic mastopathy is a perivascular lymphocytic
infiltrate. Dense collections of B-cell and T-cell lymphocytes aggregate around small vessels in the extralobular and intralobular stroma. This is not a vasculitis because there is no damage to these blood vessels. The etiology of diabetic mastopathy is unclear, but it has been suggested to be a component of HLA-associated autoimmune disease. Lymphocytic infiltrates have been noted in breast specimens of other autoimmune disease patients, such as those with lupus and rheumatoid arthritis.
infiltrate. Dense collections of B-cell and T-cell lymphocytes aggregate around small vessels in the extralobular and intralobular stroma. This is not a vasculitis because there is no damage to these blood vessels. The etiology of diabetic mastopathy is unclear, but it has been suggested to be a component of HLA-associated autoimmune disease. Lymphocytic infiltrates have been noted in breast specimens of other autoimmune disease patients, such as those with lupus and rheumatoid arthritis.
Fat Necrosis
Fat necrosis in the breast is fairly common. It may be spontaneous or may result from trauma, surgery, or radiation treatment. Acutely, the adipocytes (fat cells) are disrupted, and this is accompanied by hemorrhage and histiocytic infiltration (Fig. 2B-33). Over time, a lymphoplasmacytic infiltrate develops around the degenerating adipocytes, and histiocytes engulf the lipids released by the adipocytes; such lipid-laden histiocytes (macrophages) are referred to as lipophages (Fig. 2B-34). Multinucleated giant histiocytes are commonly found admixed in the inflammatory response. Cysts containing gelatinous material may develop in the center of the degeneration. Calcification and stromal fibrosis become prominent. Fibrosis and calcification may produce a firm masslike lesion that, on clinical examination, can be suspected for carcinoma, though the size of such lesions typically is small (on the order of 1 or 2 cm). In a core biopsy showing only fat necrosis, the possibility of a nearby occult tumor should be considered since stromal inflammation and degeneration can be seen adjacent to invasive cancer. As with any biopsy, the pathology results should be viewed in light of the imaging findings, and, if the two are not concordant, rebiopsy may be indicated.
Silicone Mastitis
Direct injection or implant leakage of silicone into the breast elicits a characteristic granulomatous inflammatory
response of histiocytes, multinucleated giant cells, and lymphoplasmacytic inflammation (Fig. 2B-35). Histiocytes ingest the silicone, but it is lost during tissue processing, leaving distinct rounded white spaces or vacuoles within the histiocytes and multinucleated giant cells (Fig. 2B-36). The features are similar to those of fat necrosis, with the addition of the telltale vacuoles within the histiocytes. As in fat necrosis, calcification, cystic degeneration, and stromal fibrosis may develop over time, resulting in firm masslike lesions.
response of histiocytes, multinucleated giant cells, and lymphoplasmacytic inflammation (Fig. 2B-35). Histiocytes ingest the silicone, but it is lost during tissue processing, leaving distinct rounded white spaces or vacuoles within the histiocytes and multinucleated giant cells (Fig. 2B-36). The features are similar to those of fat necrosis, with the addition of the telltale vacuoles within the histiocytes. As in fat necrosis, calcification, cystic degeneration, and stromal fibrosis may develop over time, resulting in firm masslike lesions.
![]() Figure 2B-35 Silicone mastitis. This is similar to fat necrosis with the addition of nondigestible silicone droplets. Over time, degeneration leads to cyst (C) formation. |
A second component of implant-related pathology is peri-implant capsule formation. The capsule is basically a band of fibrosis, often calcified, that surrounds the implant (Fig. 2B-37). Reactive inflammation is seen between the capsule and the implant. Sometimes this inflammatory reaction will organize into a pattern referred to as synovial metaplasia. The fibrohistiocytic cells line up perpendicular to the capsule surface, forming a “picket fence” arrangement reminiscent of the synovial membrane lining of joint spaces.
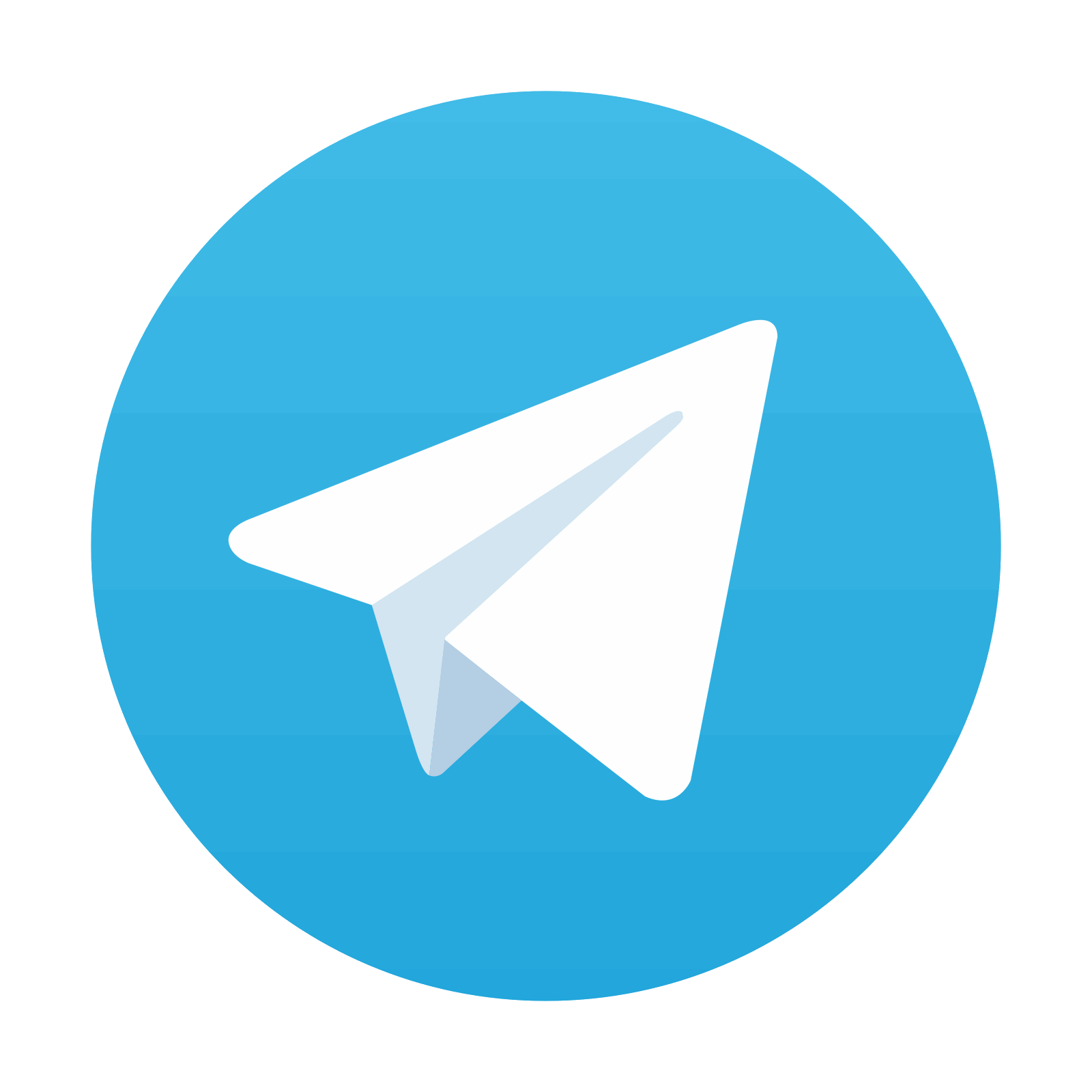
Stay updated, free articles. Join our Telegram channel

Full access? Get Clinical Tree
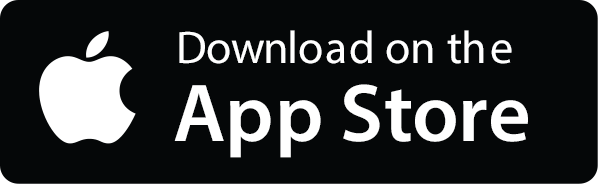
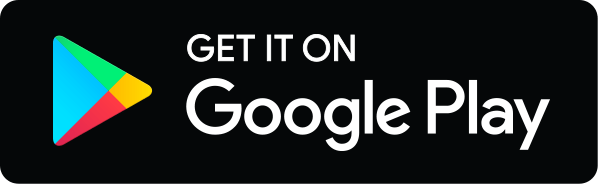
