Wrist and elbow MR imaging technology is advancing at a dramatic rate. Wrist and elbow MR imaging is performed at medium and higher field strengths with more specialized surface coils and more variable pulse sequences and postprocessing techniques. High field imaging and improved coils lead to an increased signal-to-noise ratio and increased variety of soft tissue contrast options. Three-dimensional imaging is improving in terms of usability and artifacts. Some of these advances have challenges in wrist and elbow imaging, such as postoperative patient imaging, cartilage mapping, and molecular imaging. This review considers technical advances in hardware and software and their clinical applications.
Key points
- •
Positioning of the subject near isocenter of the scanner results in the best image quality in terms of artifacts and fat suppression.
- •
High field imaging (>1.5 T) with multichannel phased array coils results in the best anatomic and contrast resolution.
- •
New methods for motion compensation, imaging around metal, and evaluation of cartilage are becoming more widely available.
Introduction
Since its introduction in the 1970s, MR imaging has revolutionized the diagnosis and treatment of musculoskeletal disorders. MR imaging has proven to be a valuable imaging tool in almost every joint in the body as a result of its ability to assess a wide range of anatomy and pathologies, ranging from ligamentous injuries to articular cartilage lesions. With its multiplanar capabilities and excellent soft tissue contrast, MR imaging has established itself as one of the most promising modalities for noninvasive evaluation of the musculoskeletal system.
The wrist and elbow are now evaluated commonly with MR imaging. Because of the mobility and location of the wrist and elbow, both can be imaged in a variety of positions using different types of coils. These locations have trade-offs in terms of patient motion and image artifacts. Most issues with imaging of these joints have been mitigated with technical advances in scanner hardware and software. Along with these improvements in technology, however, come various new technical challenges that need to be considered and understood.
Low field imaging
MR imaging at field strengths below 1.5 T is becoming less common, but is still frequently used for wrist and elbow imaging. Dedicated low field strength scanners such as the Artoscan (Esaote, Inc, Indianapolis, IN, USA) are able to produce intermediate quality images of these joints, and can be more comfortable for the patient. In addition, specialized coils can produce high-resolution images, despite the relatively low field strength ( Fig. 1 ). Low field MR imaging of the wrist is common in the evaluation of rheumatoid arthritis and is useful in scoring bony erosions and synovitis. It has also been demonstrated to be useful after wrist trauma. Examination of the elbow with low field systems is less common than the wrist.
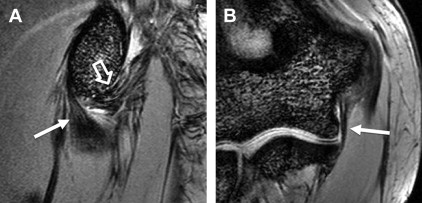
Introduction
Since its introduction in the 1970s, MR imaging has revolutionized the diagnosis and treatment of musculoskeletal disorders. MR imaging has proven to be a valuable imaging tool in almost every joint in the body as a result of its ability to assess a wide range of anatomy and pathologies, ranging from ligamentous injuries to articular cartilage lesions. With its multiplanar capabilities and excellent soft tissue contrast, MR imaging has established itself as one of the most promising modalities for noninvasive evaluation of the musculoskeletal system.
The wrist and elbow are now evaluated commonly with MR imaging. Because of the mobility and location of the wrist and elbow, both can be imaged in a variety of positions using different types of coils. These locations have trade-offs in terms of patient motion and image artifacts. Most issues with imaging of these joints have been mitigated with technical advances in scanner hardware and software. Along with these improvements in technology, however, come various new technical challenges that need to be considered and understood.
Low field imaging
MR imaging at field strengths below 1.5 T is becoming less common, but is still frequently used for wrist and elbow imaging. Dedicated low field strength scanners such as the Artoscan (Esaote, Inc, Indianapolis, IN, USA) are able to produce intermediate quality images of these joints, and can be more comfortable for the patient. In addition, specialized coils can produce high-resolution images, despite the relatively low field strength ( Fig. 1 ). Low field MR imaging of the wrist is common in the evaluation of rheumatoid arthritis and is useful in scoring bony erosions and synovitis. It has also been demonstrated to be useful after wrist trauma. Examination of the elbow with low field systems is less common than the wrist.
High field imaging
MR imaging of the wrist and elbow is now commonly performed at intermediate field strengths of 1.5 T or higher. Imaging at 3.0 T has become increasingly common for clinical evaluation, and even higher field systems (7.0 T) are being evaluated in the research realm. Although initially used for neurologic imaging, numerous studies have confirmed the benefits and abilities of higher field systems in musculoskeletal imaging. In particular, the development of dedicated coils has increased the utility of high field imaging. The most valuable benefit is an improved signal-to-noise ratio (SNR), which can result in increased image resolution ( Fig. 2 ). Additionally, increased SNR affords the opportunity to shorten the examination time. However, with the increase in field strength to 3.0 T or higher, numerous technical factors must be considered to optimize its intrinsically superior imaging capabilities.
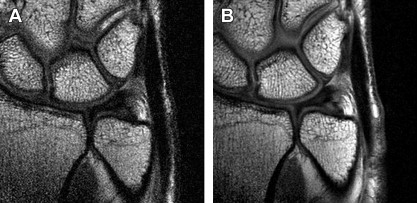
Although one would assume that doubling the field strength from 1.5 to 3.0 T should result in double the intrinsic SNR, it actually results in slightly less than a 2-fold increase because of changes in T1 relaxation times and complexities of coils at higher field strengths. Research measuring changes in relaxation times have shown that T1 relaxation times must be increased by 14% to 20% when moving from 1.5 to 3.0 T. Increased off-resonance effects may result in higher receiver bandwidth for some sequences, which in turn, reduces SNR.
There are several technical considerations that must be addressed to take full advantage of 3.0 T and higher field imaging systems. The most prominent issues include chemical shift, fat saturation, and radiofrequency (RF) power deposition. Chemical shift displacement artifact doubles in the frequency encoding direction when moving from 1.5 to 3.0 T. Doubling the receiver bandwidth is one way to resolve this issue. Doubling the bandwidth not only corrects the chemical shift artifact, but may also allow for an increase in the number of slices acquired, decrease metal artifacts, shorten echo times, and reduce echo spacing. On the other hand, doubling the bandwidth decreases the SNR by a factor of √2, because the overall readout window length is shorter.
The doubled chemical shift difference between the fat and water resonance at 3.0 and 1.5 T makes fat saturation much easier. The peaks are twice as far apart with a chemical shift of 440 Hz, meaning that the lengths of the fat saturation pulses can be shortened from about 16 to 8 msec. An advantage of this is the ability to acquire more slices at a given repetition time, bandwidth, and slice thickness.
RF power deposition is a third technical issue, especially in fast or turbo spin-echo sequences used in musculoskeletal imaging. RF power is proportional to the square of the field strength; therefore, it will quadruple when field strength is doubled from 1.5 to 3.0 T. Although the overall deposition depends on the number of RF pulses and amplitude, using rapid imaging sequences with lower flip angles may minimize the deposition. When examining small volumes and using transmit receive coils, this complication should be diminished, because the RF power that is deposited is a function of the tissue volume excited. However, many dedicated wrist and surface coils use the body coil to transmit RF energy and can result in high specific absorption rate (SAR).
It is more advantageous to use a localized transmit/receive RF coil than a body coil transmit; however, if a body coil transmit is used, shortening the examination time or lowering the refocusing pulses would help to limit SAR. The US Food and Drug Administration limitation for the whole body over a 15-minute period in all patients is 4 W/kg and for extremities over a period of 5 minutes the local SAR limit is 12 W/kg.
The use of 7.0 T to image the musculoskeletal system is still in the early stages, and there are many technical problems, including SAR, chemical shift, and B1 homogeneity. Routine imaging at 7.0 T could provide higher SNR, higher resolution, or more rapid imaging. Multichannel coils with parallel transmission capability are under development, which promise to improve image quality and lower SAR.
Phased array coils/parallel imaging
Higher field strength MR imaging systems are being developed to increase SNR and improve resolution. However, tissue/field interactions often develop owing to a higher precession frequency and shorter wavelength, making it difficult to acquire high-quality images. Phased array coil technology was developed originally to improve the signal intensity uniformity of MR images obtained using surface coils and high field imaging, while preserving the inherent SNR gain. New methods for encoding the MR imaging signal are being adopted that fall under the generic name of “parallel imaging.” Parallel imaging methods exploit the spatially varying sensitivity profiles of the surface coil elements within the array to extend the imaging field of view without adding additional scan time. This strategy allows a net reduction in the amount of time required to obtain the MR image up to a factor related to the number of independent coil channels within the array. Multiple channels are required to process the data independently, and in principle, an 8-channel coil would be able to image 8 times as fast, assuming an ideal geometry. However, practical considerations limit image acceleration to values below the theoretic maximum.
The clinical impact of parallel imaging is considerable for the higher field imaging of the wrist and elbow at 3.0 T. The use of parallel imaging technology can not only reduce scan time and the number of RF pulses required to form an image, but can also be used to shorten the echo time, which proves to be a significant improvement for musculoskeletal imaging. This will be important in limiting the total RF power to regulatory guidelines, particularly for wrist and elbow imaging with body coil transmit at 3.0 T. When parallel imaging is used, image uniformity and SNR are both compromised because scan times are reduced. Innovative phased array coil designs with up to 32 or more channels have been developed to accommodate parallel imaging methods at higher magnetic fields. The newest MR imaging systems are being offered with receiver system capacity for a highly scalable number of individual RF channels. The maximum number of RF channels that can practically be incorporated into the design of clinical MR imaging systems is controversial.
Positioning and fat suppression
Ideally, the body part of interest is placed at the isocenter of the scanner, where magnetic field homogeneity and gradient uniformity are best. However, this can be challenging for wrist and elbow imaging. Placing the wrist or elbow at isocenter usually requires prone positioning with the arm of interest over the subjects head, the so-called “superman” position. This places the anatomy of interest in the best location for imaging, but can be uncomfortable and difficult to maintain for long periods of time, and therefore prone to motion artifact ( Fig. 3 A ). Motion artifact can be improved by adding motion-insensitive sequences, such as propeller (see Fig. 3 B), to the protocol.
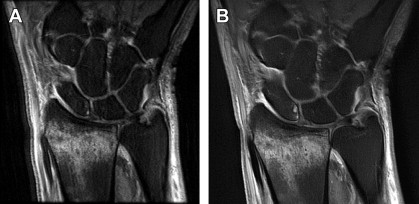
Modern phased-array multichannel coils allow placement of the wrist or elbow by the side of the patient in a supine position, which is more comfortable and less prone to motion. However, this places the anatomy far from the isocenter, and achieving high-quality imaging with fat suppression can be difficult ( Fig. 4 A ). Use of manual shimming and manual prescan to set the center frequency can often correct this problem (see Fig. 4 B). Use of short Tau inversion recovery for fat suppression or methods of fat/water separation such as Iterative Decomposition of water and fat with Echo Asymmetry and Least-squares estimation (IDEAL) can also be helpful to obtain quality fat suppressed or fat–water separated images in areas of poor magnetic field homogeneiety.
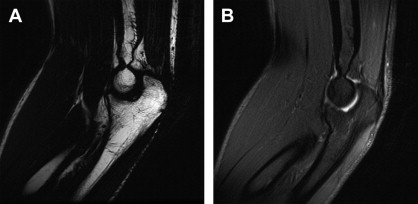
Direct and indirect magnetic resonance arthrography
MR arthrography is an important alternative to conventional MR imaging of the wrist and elbow, particularly in cases where detailed evaluation of cartilage or ligaments is vital. Direct MR arthrography distends the joint compartment, allowing for better delineation and visualization between tissues. It also allows for detection of abnormal communication between joint compartments and between extraarticular soft tissues and joint compartments. The drawbacks of direct MR arthrography are that it is invasive, thereby introducing the potential for infection, and exposes the patient to x-rays for procedure guidance.
Indirect MR arthrography is useful in joints that have less capacity for distension, and is less invasive than direct MR arthrography. A disadvantage of indirect MR arthrography is that contrast will be present in all components of the joint, preventing visualization of abnormal communication between joint compartments. In addition, the level of joint enhancement depends on joint volume, intraarticular pressure, blood concentration of contrast, inflammation and permeability, synovial area, and time delay after contrast injection. Despite the drawbacks of both direct and indirect MR arthrography, these techniques have proven useful in the evaluation of most joints including the elbow and wrist.
3.0 T MR Imaging protocols
Example 3T imaging protocols are included for the wrist ( Fig. 5 , Table 1 ) and the elbow ( Fig. 6 , Table 2 ). These protocols are relatively long in total scan time by modern standards, but include good coverage of the anatomy and high SNR and resolution. The important principle is to cover the joint in multiple planes and with multiple different tissue contrasts. Coronal images of the wrist are obtained by acquiring oblique slices oriented parallel to the ulnar and radial styloids ( Fig. 7 ). Coronal images of the elbow are prescribed in plane with a line drawn along the anterior margin of the humerus at the level of the medial and lateral condyles ( Fig. 8 ).
Sequence | FOV (cm) | TR | TE | Slice Thickness (mm) a | Matrix |
---|---|---|---|---|---|
Cor T2 FS | 8 | 5500 | 55 | 2.0 | 416 × 224 |
Ax T2 FS | 8 | 5000 | 55 | 2.8 | 416 × 224 |
Cor PD FS | 8 | 4000 | 35 | 2.0 | 384 × 192 |
Cor T1 | 8 | 750 | 20 | 2.0 | 416 × 192 |
Ax T1 | 8 | 750 | 20 | 2.8 | 416 × 192 |
Sag PD FS | 8 | 4000 | 40 | 2.5 | 320 × 224 |
Cor CUBE Flex | 12 | 2000 | 25 | 2 | 224 × 224 |
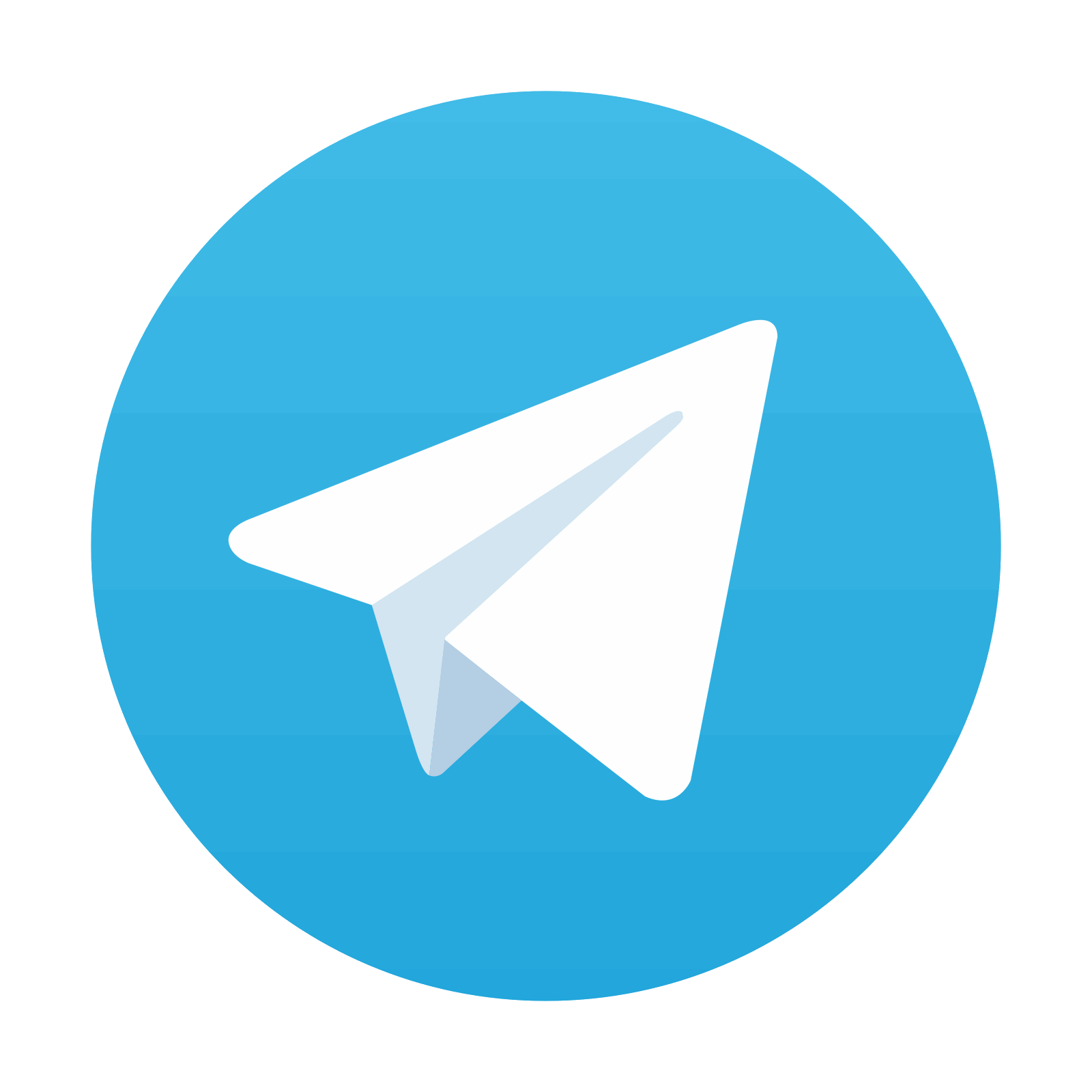
Stay updated, free articles. Join our Telegram channel

Full access? Get Clinical Tree
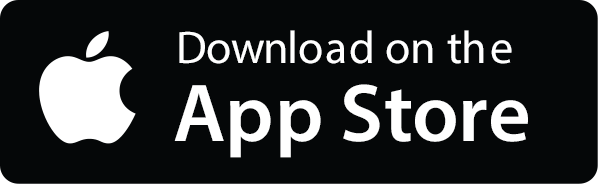
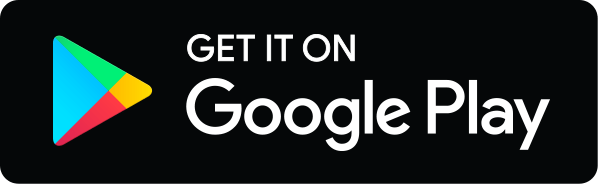
