ABSTRACT
Objective
Non-invasive surgical approaches, including boiling histotripsy (BH), are currently being developed for the treatment of brain disorders aiming to avoid craniotomy and exposure of intervening tissues, and, thus, minimize associated complications. This work aimed to demonstrate the feasibility of BH for mechanical fractionation of human brain tissues ex vivo under B-mode guidance, with preliminary measurements of tissue stiffness via shear wave elastography.
Methods
Young’s moduli of 25 human autopsy brain samples obtained from de-identified patients of 51–91 y old (median 77 y old) were measured via shear wave elastography prior to BH sonication. Seventeen volumetric BH lesions (1–4 layers of 5 × 5 points with a 1- mm step) were produced near brain surface ( n = 10), in white matter ( n = 3), in thalamus ( n = 2), and globus pallidus ( n = 2) using 12 element 1.5 MHz sector transducer under B-mode guidance with 10 ms or 2 ms pulses delivered 10 or 15 times per sonication point with 1% duty cycle. After treatment, the lesions were evaluated grossly through bisection, histologically with hematoxylin and eosin staining, and ultrastructurally via scanning and transmission electron microscopy.
Results
Young’s moduli of autopsy brain samples were lower in older patients (from 32.9 ± 6.6 kPa in 51 y olds to 10 ± 2 kPa in 91 y olds) and at higher temperature (6%–50% lower at 37°С vs 23°С), and were within the range observed clinically. All tested BH treatments performed near the brain surface (i.e., mostly in gray matter) resulted in formation of well-demarcated rectangular lesions with homogenized content and sharp boundaries, with majority of residual fragments below 100 microns. The use of shorter pulses (2 ms vs 10 ms) accelerated the treatment at least threefold, and the highest liquefaction rate was 568 mm 3 /min. White matter was more resistant to BH vs gray matter: at least 15 pulses of 2 ms duration were required per each sonication point, and the liquefaction rate was three times lower. The ability of BH to produce lesions in thalamus and globus pallidus was also confirmed.
Conclusion
This work presents the first demonstration of BH proof-of-concept in human brain tissues ex vivo under B-mode guidance with clinically relevant treatment rates.
Introduction
Standard protocols for radical management of brain diseases currently include chemotherapy and surgical resection of the target area, which involves craniotomy and incision made through healthy tissues on the way to the target region. Thus, resection of most brain tumors is associated with a high risk of infection, bleeding and other complications, and the surgery outcome highly depends on the tumor size and location in the brain. Minimally invasive procedures, such as endoscopic surgery [ ], cryoablation [ ] and radiofrequency ablation [ ] allow to reduce the craniotomy size and shorten the post-operative recovery period; however, they still require removal of the skull bone and exposure of the intervening tissues to reach the target area. Radiation therapy can allow completely non-invasive ablation of the target tissue transcranially; however, it uses ionizing radiation that affects healthy intervening tissue on the way to the target area and is known to have a cumulative effect on the entire body [ ].
Focused ultrasound examination has been suggested as a non-invasive approach to treat brain disorders, for example, to enhance targeted drug delivery through sonoporation and, thus, reduce the required drug doses or use ultrasound-released drugs [ ]. Furthermore, high-intensity focused ultrasound (HIFU) modalities provide a non-invasive approach to treat brain disorders by heating the target tissue up to the temperatures of thermal necrosis under magnetic resonance imaging guidance [ ]. Thermal HIFU technology is already approved by the U.S. Food and Drug Administration to treat essential tremor and Parkinson’s disease by ablating a region in thalamus [ ] or globus pallidus [ ], both of which are located in the central region of the brain, and is currently undergoing clinical trials for the treatment of other neurological disorders and brain tumors [ ]. Such heating-based HIFU modality, however, is known to have certain limitations, including heat sinking and heat diffusion beyond the focal spot that reduce the ablation precision, as well as a reliance on rather costly magnetic resonance imaging guidance. Moreover, the semispherical shape of the current clinical system (ExAblate, Insightec, Inc., Tirat Carmel, Israel) and significant attenuation and distortion of the ultrasound beam on the way through skull bones limit the available area of ablation to the central region of the brain and can result in overheating of the skull and low treatment rate of volumetric targets [ ].
A newer HIFU-based modality, termed histotripsy [ , ], is currently being developed for neurosurgical purposes as an alternative to thermal HIFU methods. Instead of heating the tissue with continuous ultrasound exposure, histotripsy uses short (microseconds- to milliseconds-long) ultrasound pulses of much higher amplitude containing shock fronts at the focus to fractionate the target tissue mechanically into non-viable suspension. The underlying mechanisms involve gas and vapor bubble activity in the treated area that are highly reflective of ultrasound, which allows the use of conventional B-mode imaging to monitor the treatment.
Intrinsic threshold histotripsy relies on initiation of cavitation within the focal area in tissue by focusing microseconds-long pulses with a greater than 26 MPa peak negative pressure at the focus [ ]. The method is being investigated currently for non-invasive neurosurgery on in vivo rat and pig models [ ], as well as for endoscopic procedures [ ]. First in vivo studies have shown that histotripsy brain treatment results in minor to no bleeding which resolves within 1 wk after treatment. However, this type of histotripsy requires significant negative pressures to be reached at the focus, and therefore, relies on the use of rather large strongly focused transducers. Although the use of multi-element arrays allows expansion of the effective area of treatment via electronic steering of the focus [ ], the large form factor of intrinsic threshold histotripsy transducers still limits the range of focus positions within the brain.
Boiling histotripsy (BH) uses longer (milliseconds-long) ultrasound pulses to initiate rapid and highly localized tissue boiling within each pulse. BH is feasible with lower acoustic pressures produced at the focus and, therefore, can be realized using more compact transducers than that required for intrinsic threshold histotripsy. This allows the use of less focused arrays for BH that have higher degree of freedom in terms of their positioning relative to the patient’s head, thus increasing the available treatment envelope in the brain [ ].
Moreover, BH individual lesions are typically larger than those produced via cavitation-based histotripsy; therefore, BH treatment rates are typically higher [ , ]. Therefore, although intrinsic threshold histotripsy that produces more compact lesions can be favorable for the treatment of smaller targets, BH may provide a more suitable option for non-invasive resection of large target volumes and accelerate their treatment.
Although it has been shown numerically that the shock amplitudes required for BH brain treatment are achievable when focusing through a human skull [ ], the feasibility of BH for human brain ablation has not been investigated experimentally yet. Thus, this study was aimed at the first experimental demonstration of BH feasibility in human brain tissues ex vivo . The study consisted of two parts. In the first part, the stiffness (i.e., Young’s modulus) of autopsy brain samples was measured via a shear wave elastography (SWE) method to confirm their clinical relevance in terms of the stiffness, which is currently considered as the main metric of tissue resistance to BH [ ]. In the second part, we aimed to show the ability of BH to generate lesions in tissues located in different parts of the brain: near the brain surface where gray and white matter merge, specifically within the white matter, as well as in thalamus or globus pallidus, which are currently ablated clinically via thermal HIFU technique to treat essential tremor and Parkinson’s disease [ , ].
Methods
Autopsy brain tissue samples
De-identified brain tissue specimens were obtained via rapid autopsy (<24 after death, IRB exempt) from 14 anonymous patients 51–91 y olds (median, 77 y old): 7 males and 7 females. The specimens were then dissected into smaller samples ( Fig. 1a – h ) and divided into three groups: 13 samples were used, first, for SWE measurements and then sonicated with BH, 12 samples were only used for SWE measurements, and 4 samples were only used for BH. All brain samples were used within 24 h after autopsy.
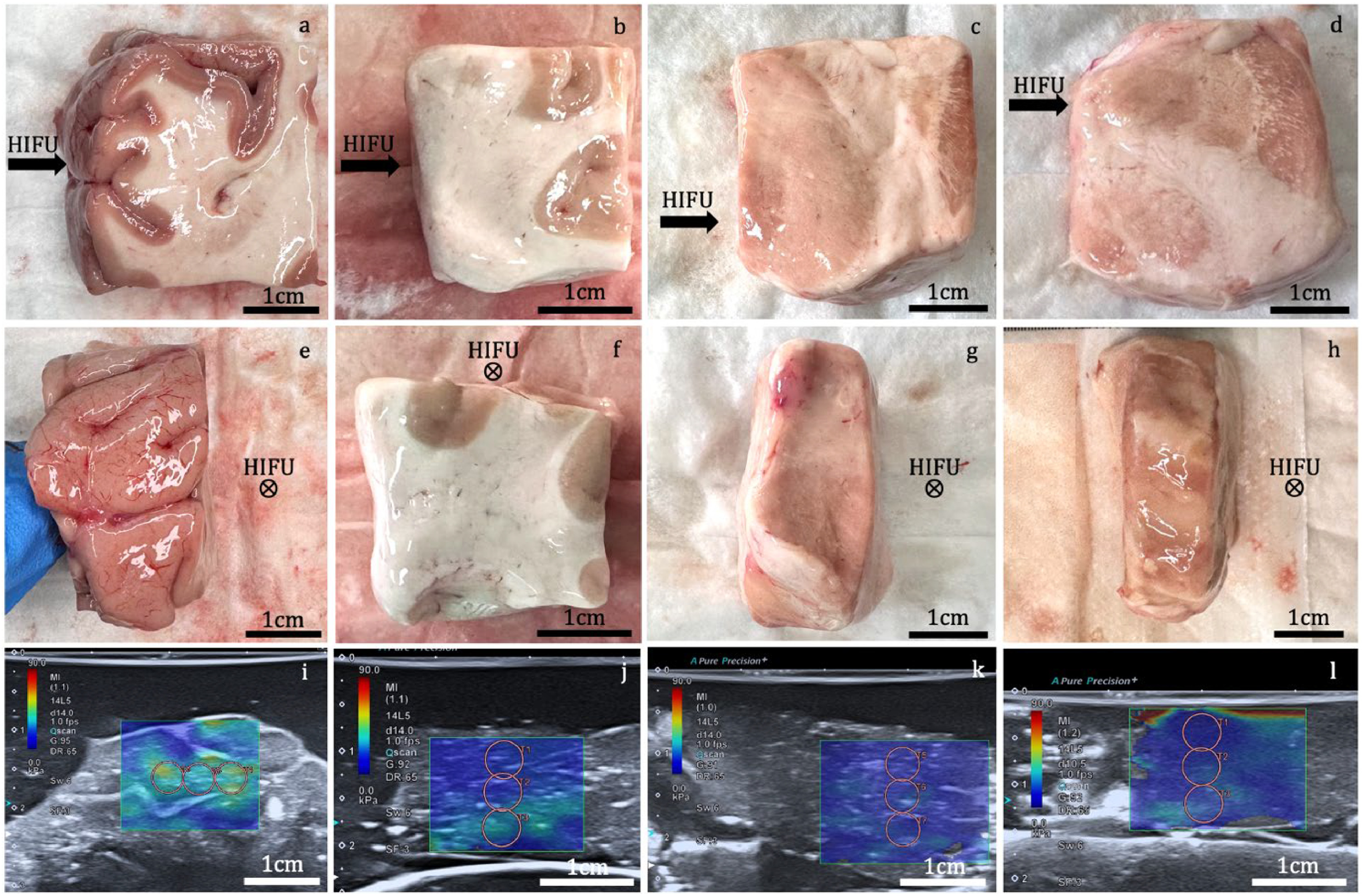
SWE measurements
Young’s moduli of 25 brain tissue samples were measured on Aplio i800 system (Canon, Tokyo, Japan) using linear 14L5 probe in Thyroid preset. The measurements were performed in a plastic container filled with phosphate-buffered saline on a 4 cm high silicone absorbing layer (ToolDecor 25, BMP Technology, Moscow, Russia). At least three measurements were performed in each sample in 4 mm circle areas: 13 samples were measured near brain surface at 1 cm depth ( Fig. 1i ) at 23°С, 8 samples were used to measure white matter stiffness ( Fig. 1j ) at 23°С vs 37°С, and stiffness of thalamus and globus pallidus were measured in 2 samples each ( Fig. 1k , 1 l ).
The measured values of Young’s moduli were compared across different patients with regard to their age and across varied temperatures for each sample individually via two-tailed two-sample homoscedastic Student’s t -test. The significance threshold was set at p = 0.05.
BH setup and protocols
Before sonication, 15 brain samples were subjected to 2.5 cycles of alternating degassing (30 min each) and compression (15 min each), and embedded into a 1.5% agarose gel (UltraPure Agarose, Invitrogen, Carlsbad, CA, USA) for the ease of sample positioning during the BH treatment ( Fig. 2a ). Ten samples were sonicated through gyri near the brain surface at 1 cm depth where typically gray and white matter merged ( Fig. 1a , 1 e , 1 i ), 3 samples were used to treat solely white matter without gyri on the beam path ( Fig. 1b , 1 f , 1 j ), and 2 lesions were produced per thalamus ( Fig. 1c , 1 g , 1 k ) and globus pallidus ( Fig. 1d , 1 h , 1 l ). Overall, 17 volumetric BH lesions were produced.
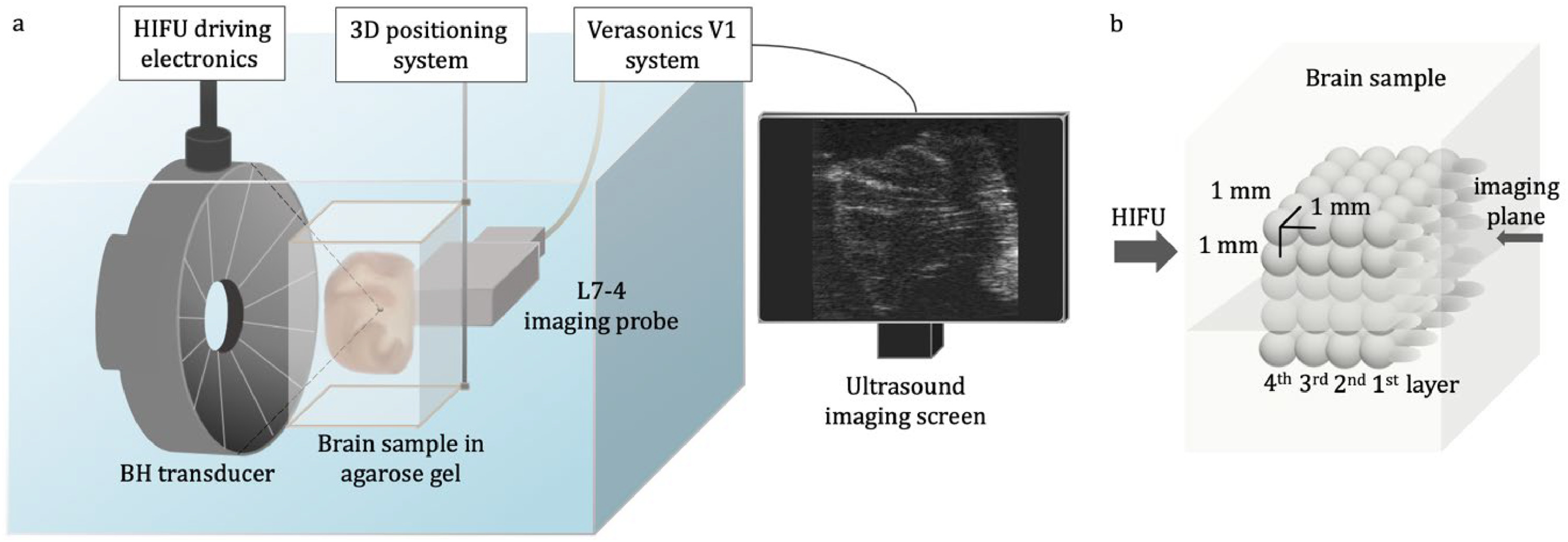
The BH treatments were performed in 34°С water degassed for 3 h with PA WTS (water treatment system, Precision Acoustics, Dorchester, UK) using a 12 element 1.5 MHz sector transducer described in [ ], with a 6 cm focal length, an 8 cm outer diameter and a 2 cm central opening diameter ( Fig. 2a ). The B-mode guidance of the treatment was performed using an L7-4 ultrasound probe (ATL, Philips, Cambridge, MA, USA) positioned on the side of the sample and aligned with the treatment plane ( Fig. 2a ).
One volumetric lesion per sample was produced by sonication of a 3-D grid of points at an average depth of 1 cm ( Fig. 2b ). The grid consisted of 1–4 layers, 5 × 5 points each, with a 1 mm increment in all directions as was successfully performed in human prostate and leiomyoma tissues [ , ]. Relative movement of the BH focus and tissue sample was performed by mechanical movement of the agarose-embedded sample attached to a 3-D positioning system (UMS3, Precision Acoustics, UK). Each point of the grid was sonicated with 10-ms or 2-ms pulses with 10 or 15 pulses per point ( ppp ). In this pilot study, the treatments were performed at the maximum achievable output voltage driving the transducer ( U = 240 V) to ensure successful fractionation of brain tissue. Boiling of the tissue at the selected output voltage was confirmed experimentally by the appearance of a hyperechoic spot on the B-mode during BH treatment, indicating formation of boiling bubbles in the focal area in tissue.
Estimation of shock wave parameters in situ
Experimental peak acoustic pressures and the amplitude of the shock front in brain tissue at the selected voltage were evaluated via numerical simulations in the HIFU beam software [ ] as described in [ ] in a “water–brain” flat-layered medium with the focus positioned 1 cm under the tissue surface. Acoustic properties of brain tissue required for modeling were taken from the literature [ ]: density ρ = 1046 kg/m 3 , speed of sound c = 1546.3 m/s, nonlinearity coefficient β = 4.36, diffusivity coefficient δ = 4.33 mm 2 /s, absorption coefficient α = 0.106 Np/cm at a 1.5 MHz frequency, with power law ν = 1.1. The calculated peak positive pressure field around the focus and the focal pressure waveform are shown in Figure 3 . The axial and lateral dimensions of the focal spot at the selected voltage (i.e., in non-linear regime) were 4.7 mm × 0.42 mm at −3 dB peak positive pressure, and 12.2 mm × 1.7 mm at zero pressure ( Fig. 3a ). The maximum achievable shock wave parameters at the focus were as follows: peak positive and negative pressures were 138 and −24 MPa, respectively, and the shock amplitude was 155 MPa ( Fig. 3b ). The time to reach tissue boiling, given the heat capacity per unit volume of brain tissue c v = 3.8 MJ/(m 3 ·K) [ ], was evaluated according to the standard procedure based on the energy absorption at the shock fronts [ , ]: t b = Δ T c V 6 ρ 2 c 4 / ( β f 0 A s 3 ) , where Δ T is the temperature change from the tissue temperature to the boiling temperature, f 0 is the ultrasound frequency, and A s is the shock amplitude at the focus. Thus, the time to reach tissue boiling was shown to be 0.44 ms, that is, within each BH pulse, as required for BH. However, the simulations did not account for any inhomogeneities on the beam path, and numerically predicted shock wave parameters could be overestimated and boiling time—underestimated. Therefore, the tissue boiling within the intended BH pulse was confirmed experimentally on the B-mode as described elsewhere in this article.
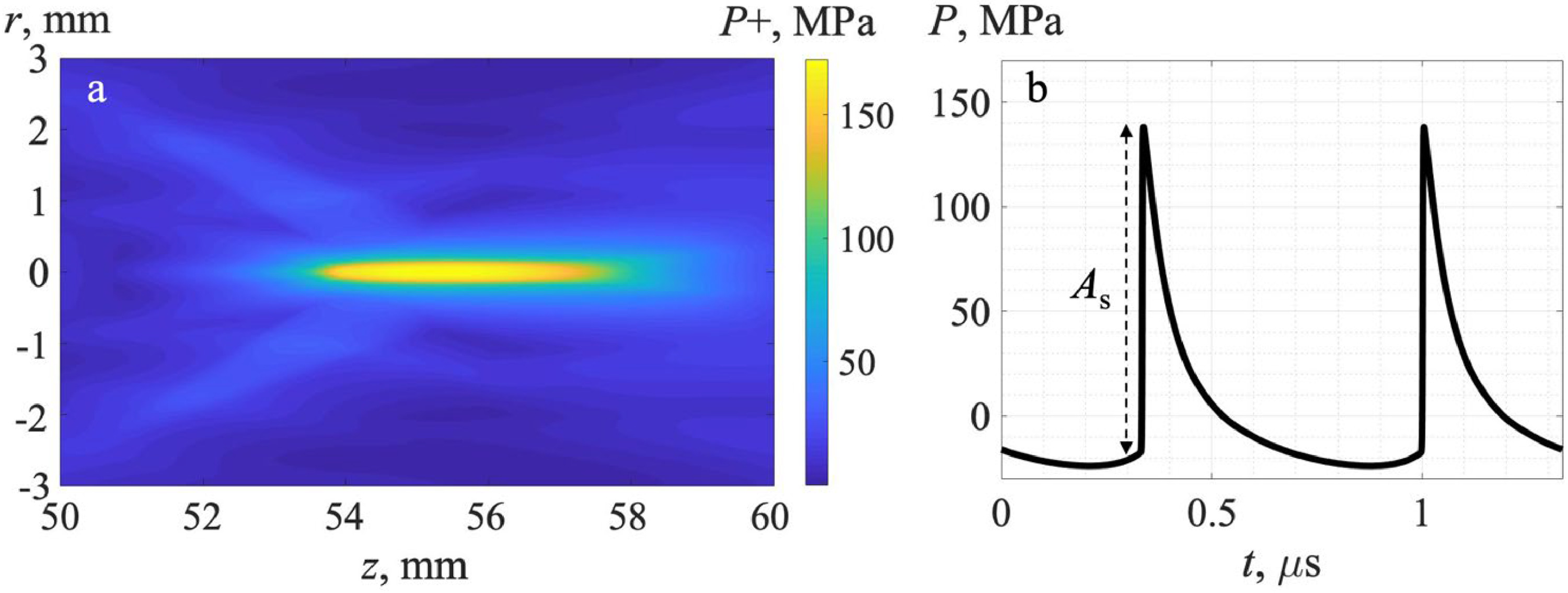
Microscopic analysis of BH outcome
After treatment, the obtained volumetric lesions were investigated via B-mode imaging, then 11 lesions were bisected along the imaging plane for gross analysis, and the other 6 lesions were marked with histological inks (HistoSafe, Moscow, Russia), fixed en bloc in 10% neutral buffered formalin (Labiko, St. Petersburg, Russia), cut into 2 mm thick sections parallel to the imaging plane, processed and embedded in paraffin. Then, 3 μm thick sections were taken from the resulting blocks, processed and stained with hematoxylin and eosin for histological evaluation of the BH outcome by a certified pathologist (N.D.).
Lesion volumes were estimated as a product of three dimensions measured either grossly after bisection, or in histological slides. The liquefaction rate was then evaluated as the lesion volume divided by the treatment time.
The content of 7 of 11 bisected lesions was collected with a scalpel and either placed on a carbon double-sided tape and prepared for scanning electron microscopy (SEM) as described in [ ], or diluted with distilled water to separate individual residual fragments, and processed for SEM or transmission electron microscopy (TEM) as described in [ ]. SEM specimens containing diluted lesion content were digitalized on JEOL JSM-6380LA Analytical Scanning Electron Microscope (Tokyo, Japan), and maximum Feret diameters of all residual fragments of brain tissue exceeding 20 µm were measured in ImageJ software (National Institutes of Health, Bethesda, MD, USA). A two-tailed two-sample Student’s t -test was performed to compare the measured values of fragment sizes across varied BH protocols and brain tissues. Overall, 2786 residual fragments were analyzed. Significance threshold was set at p = 0.05. TEM specimens were digitalized on JEOL JEM-1011 Transmission Electron Microscope (Tokyo, Japan).
Results
Stiffness of autopsy brain tissue samples
Figure 4a shows Young’s moduli measured via SWE near the brain surface through gyri at 1 cm depth for patients of different age and sex. The autopsy samples of younger patients were statistically stiffer, as per Student’s t -test, which agrees with the literature [ ]. SWE measurements of white matter in samples kept at 23°С vs heated up to 37°С ( Fig. 4b ) showed that brain stiffness decreased with temperature, as per Student’s t -test, which also agrees with the literature [ ]. Specifically, Young’s modulus range in autopsy white matter was 15.4–34.9 kPa at 23°С and 8.9–17.9 kPa at 37°С, being within the broad range reported in literature [ , ]. The Young’s modulus of thalamus and globus pallidus at 23°С were statistically similar: 13.8 ± 3.2 kPa and 12.4 ± 3.6 kPa, respectively. All stiffness values obtained here were within the typical range observed clinically [ , ], which confirms clinical relevance of the autopsy brain samples used here in ex vivo studies.
