While MR imaging is the main imaging modality to assess brain tumors, PET imaging has a specific role. Among the many tracers that have been proposed and are still being developed, 2-[ 18 F]fluoro-2-deoxy- d -glucose ([ 18 F]FDG) and O-(2-[ 18 F]-fluoroethyl)- l -tyrosine ([ 18 F]FET) PET remain the most solidly established in the clinics. In particular, [ 18 F]FET has gained increased acceptance due to its higher sensitivity. In this paper, we present an overview of the current clinical status of brain tumor imaging, with emphasis on PET imaging.
Key points
- •
2-[ 18 F]fluoro-2-deoxy- d -glucose ([ 18 F]FDG) PET/computed tomography scan is a reliable and accessible tool for characterizing suspicious MR imaging lesions, guiding biopsy, and identifying recurrence/persistence of high-grade gliomas, but it has limitations.
- •
Amino acid PET imaging is recommended in conjunction with MR imaging for primary malignant brain tumors and metastases assessment.
- •
Amino acid radiotracers are better than [ 18 F]FDG for low-grade gliomas, and [ 18 F]FET is the most used one.
- •
New radiotracers are continuously being evaluated in the diagnosis and follow-up of brain tumors. Radiotracers based of fibroblast activation protein inhibitor are one of the most studied by now.
Introduction
Cancer is one of the leading causes of death. According to the World Health Organization (WHO) in 2019, it is the first or the second leading cause of death before the age of 70 years in 112 out of 183 countries. Malignant brain tumors can be primary brain tumors or metastases. In adults with malignant brain tumors, the most common are metastases while gliomas are the second one and account for 81% of primary brain tumors. Brain metastases are 10 times more frequent than primary malignant brain tumors.
In 2021, the fifth edition of the WHO classification of tumors of the central nervous system (WHO CNS5) was published. It introduces major changes that advance the role of molecular diagnostic markers to histology and immunohistochemistry in the central nervous system (CNS) tumor classification.
In this new update, adult-type diffuse gliomas are divided into 3 main groups :
- •
Astrocytoma, isocitrate dehydrogenase (IDH)-mutant;
- •
Oligodendroglioma, IDH-mutant, and 1p/19q-codeleted; and
- •
Glioblastoma, IDH-wild type.
Defining tumor genetics correctly is crucial as the prognosis is affected by it. In the present review, we use the terms “WHO grade 2 (and 1)” and “WHO grade 3 and 4” gliomas for “low grade” and “high grade” respectively, unless otherwise stated.
MR imaging is the main imaging modality used in the diagnosis and follow-up of brain tumors, due to its high sensitivity and specificity, as well as spatial resolution and relative availability (compared to PET imaging). It is a multiparametric exploration, using multiple sequences, each with a specific purpose, with excellent tissular contrast. It allows the differential diagnosis between intracranial tumor and other mimics, such as brain abscesses, inflammatory pseudotumors, and vascular lesions. It has some limitations in the post-treatment follow-up, mainly to identify early progression versus post-therapeutic effects, and its specificity can be increased by some more advanced MR imaging techniques and with the use of metabolic nuclear imaging.
Nuclear medicine imaging includes single photon emission computed tomography (SPECT) and PET. The great variety of used radiotracers provides physiologic and functional information and allows in vivo evaluation of metabolic and molecular processes (eg, glucose metabolism, nucleoside and amino acid [AA] transporter expression). , Spatial resolution is limited for SPECT and PET imaging, thus it needs the support of high-resolution anatomic information that can be provided by computed tomography (CT) scan or by MR imaging. , PET/CT scan or PET/MR imaging can help for the diagnosis and the follow-up of brain tumors. The most common PET tracer in daily-practice is the 2-[ 18 F]fluoro-2-deoxy- d -glucose ([ 18 F]FDG). Other tracers are used like O-(2-[ 18 F]-fluoroethyl)- l -tyrosine ([ 18 F]FET), [ 11 C]-methylmethionine ([ 11 C]MET), 3,4-dihydroxy-6-[ 18 F]-fluoro- l -phenylalanine ([ 18 F]FDOPA), and many others. , ,
Treatment of brain tumors is multimodal. Surgery is the standard of care and the whole treatment is based on tumor histology and preoperative considerations. Radiation therapy such as stereotactic radiosurgery (SRS) or conventional radiation therapy is chosen depending on tumor type and number of lesions. Over the years, SRS has become a standard of care for many patients with brain metastases. Additional therapies are often used (eg, steroids, intravenous chemotherapies, and intrathecal chemotherapy agents) and new treatment agents (chemotherapy or immunotherapy agents) are continuously developed. All these therapies can induce post-treatment modifications of the tumor that need to be taken into account in neuroimaging assessment. , , ,
The present paper aims to summarize the current knowledge about brain tumor imaging, especially using PET imaging.
Imaging techniques
Computed Tomography
CT holds a limited role in the overall imaging of brain tumors. Its sensitivity and specificity are lower than those of MR imaging, and it is thus mainly used as a first-line modality of differential diagnosis of acute neurologic symptoms, before and after treatment.
MR Imaging
MR imaging is used in the initial diagnosis of the tumor, surgical planning, and treatment response follow-up. Imaging protocol will differ between those specific situations. The sequences being routinely used are 3 dimensional (3D) T1-weighted imaging before and after contrast media administration, T2 and fluid-attenuated inversion recovery (FLAIR) pondered sequences, diffusion-weighted imaging (DWI), MR spectroscopy (MRS), perfusion-weighted imaging (PWI – T2 ∗ ), and susceptibility-weighted imaging (SWI). Of those, T1 imaging, before and after gadolinium-based contrast media injection, shows the extravasation and/or concentration of gadolinium in pathologic areas. Tumor growth is associated with anarchic neoangiogenesis, creating neovessels with incompetent blood–brain barrier (BBB), which will concentrate and leak gadolinium in the pericellular space. Spin-echo-based T1 acquisition has greater sensitivity than gradient-echo T1 and should be performed whenever available, although at a greater cost in time.
T2 and FLAIR acquisitions are very sensitive for the detection of vasogenic edema and tumor infiltration (both appearing as a hyperintensity). T2/FLAIR mismatch is a very specific sign for 1p/19q-codeletion, and thus for oligodendroglioma diagnosis.
DWI shows restriction of the diffusion of water molecules, which is seen in the hypercellular parts of the lesion, or in those where tumor growth has surpassed its capacity to obtain enough metabolic support via neoangiogenesis, and its suffering from cytotoxic edema. Although highly specific, this feature has a low sensitivity.
PWI (with dynamic susceptibility contrast) has become an integral component of the classic neuro-oncologic MR imaging protocol. It makes possible the semiquantitative measurement of regional cerebral blood volume (CBV) and estimates the diffusivity of contrast through BBB. CBV is higher in tumors with important neoangiogenesis, such as high-grade gliomas (HGG), but also some brain metastasis (BM), meningiomas and hemangiomas. PWI can be used in the initial diagnosis of an intra-axial tumor, to differentiate HGG from BM, and in the oncologic follow-up, to help differentiate early progression from post-therapeutic effects (radionecrosis and pseudoprogression).
MRS is able to analyze directly the spectral curve of brain metabolites in a region of interest, such as N-acetyl-aspartate of acetylcholine. As those metabolites ratios are altered in pathologic states, MRS can be useful to differentiate tumoral tissue from nontumoral tissue, and to some extent to help characterize tumoral entities. It suffers from low spatial resolution and field of view and is heavily influenced by the surrounding environment, limiting its accuracy for lesions located near bone, air, or calcified tissues. Future sequences, allowing 3D MRS imaging of the brain, might solve some of those limitations.
SWI , uses the paramagnetic artifacts of paramagnetic, diamagnetic, and ferromagnetic components of brain tissue, to highlight blood degradation products (consequences of hemorrhage) and calcifications in the tumoral tissues, precising its differential diagnosis.
Main goals of the initial MR imaging exploration of a suspected brain tumor include the precise determination of the lesion’s location and extension, its proximity to key cerebral structures (highly functional areas, ventricular system, and cerebral vasculature), evaluating its repercussion on the adjacent brain parenchyma (invasion, mass effect, and engagement), and if possible, to suggest the most probable diagnosis(es). It has a very important role in the confirmation of the probable tumoral origin of the lesion and excluding other etiologies.
Preoperative tumor exploration often uses diffusion tensor imaging (DTI) and is blood oxygenation level dependent to image white fiber tracts and functional areas in the brain near the lesion.
The main challenge encountered with MR imaging in the follow-up of the patients after treatment is the distinction between tumor progression and post-therapeutic effects, which are frequent after radiation therapy and immuno-therapy. Classic MR imaging scans have an imperfect specificity for this crucial distinction, and advanced techniques or metabolic imaging are necessary to increase confidence in imaging results and choose the best course of action for the patient.
To address the challenges met with conventional MR imaging, multiple sequences have been and are currently evaluated. One of the main issues encountered with those sequences are the difficulty of implementing them in clinical routine, as they often have high scan duration, high postprocessing time, or need dedicated postprocessing software. Of those future techniques, MR imaging-based metabolic imaging is the one closer to clinical implementation, with chemical exchange shift transfer (CEST). CEST will indirectly measure the concentration of a specific target, like proteins with amide-proton-transfer CEST, its more mature version. In this case, proteins will act like endogen contrast media, and their concentration can be quantified. As protein concentration increases in tumoral tissue, it could be very useful to differentiate tumor progression from post-therapeutic effects.
Relaxometry is a technique, which can theoretically allow quantitative measurement of T1 and T2 values of a specific tissue, independently of magnetic field strength or constructor parameters. It could allow more precise delimitation of tumor invasion than T1-weighted sequences and detect more precociously tumor progression.
Many more advanced MR imaging techniques are under scrutiny, such as arterial spin labeling, sodium (Na) imaging, variants of DTI (diffusion spectrum [DSI], q-space imagining [QSI], and neurite orientation dispersion and density imaging [NODDI]), or hyperpolarized MR imaging, whose precise description exceeds the scope of this review.
PET/Computed Tomography
2-[ 18 F]fluoro-2-deoxy- d -glucose PET imaging
Aerobic glucose metabolism is the main brain energy source. , Glucose is transported by glucose transporters (GLUT), mainly GLUT1 and GLUT3, across the BBB and its accumulation into brain tumors does not depend on the BBB disruption. Intracellular accumulation of [ 18 F]FDG is proportional to glucose metabolism and increased concentration shows high cellular metabolism. , For brain imaging, physiologic background uptake of the brain is a significant limitation, especially for accurate delineation of the tumor. , , [ 18 F]FDG uptake is not specific to tumoral processes, the inflammatory or infectious conditions also have a high [ 18 F]FDG uptake. , [ 18 F]FDG uptake is not always high in tumors or tumor environment (eg, necrosis can be find in high-grade tumors leading to decreased [ 18 F]FDG uptake). As [ 18 F]FDG PET evaluates brain glucose metabolism, it requires a minimum of 4 to 6 hours of fasting before examination. If fasting has not been performed or in case of hyperglycemia or diabetes for example, examination sensitivity can be diminished.
The WHO grade 3 and 4 gliomas tend to have high [ 18 F]FDG uptake, similar to or higher than that of gray matter. In contrast, lower grade gliomas tend to have similar uptake than white matter, making them more challenging to distinguish from normal cortical metabolism. The tumor to contralateral normal brain standardized uptake value (SUV) ratios (TBRs) can be used to differentiate WHO grade 3 and 4 tumors from WHO grade 1 and 2. Delbeke and colleagues , proposed a TBR cutoff value of 0.6 for tumor-to-cortex maximum SUV (SUV max ) ratio, which yields a positive predictive value (PPV) of 77% and a negative predictive value of 100% for gliomas; for all brain tumors, these values are 83% and 91%, respectively. Another tumor to contralateral normal cortex ratio of 0.9 was proposed as a threshold to distinguish high from low-grade gliomas with a diagnostic accuracy of 89%. Indeed, Takahashi and colleagues demonstrated that tumors with high [ 18 F]FDG uptake have a high PPV of 93% for high-grade tumor with the cutoff value for the tumor-to-normal ratio of 0.9 or greater. Nevertheless, this TBR value can be high in some WHO 1 and 2 grade tumors, which limits the accuracy of [ 18 F]FDG PET/CT scans in predicting the glioma grade.
Moreover, there can be false-negative findings in [ 18 F]FDG PET/CT scans, such as in WHO grade 2 gliomas or metastases, as well as false-positive findings, such as in inflammatory lesions. Multiphase [ 18 F]FDG PET/CT scans can aid in evaluating gliomas and metastases because normal brain [ 18 F]FDG activity decreases over time, whereas malignant tumor activity remains steady or increases. , , In case of suspicion of brain lymphoma, [ 18 F]FDG PET/CT scan of the whole body is recommended for disease staging. , Moreover, [ 18 F]FDG uptake is an independent prognostic factor in primary CNS lymphoma and in WHO grade 4 gliomas at diagnosis and after treatment. , , [ 18 F]FDG PET/CT scans can help to guide biopsy to the areas where the [ 18 F]FDG uptake is the highest that are more alike to correspond to WHO grade 3 and 4 gliomas.
PET imaging lacks spatial resolution and, therefore, requires the support of high-resolution anatomic information, which can be provided by CT scan or MR imaging. , It appears that [ 18 F]FDG PET combined with MR imaging is superior to [ 18 F]FDG PET/CT for diagnosis purpose. Additionally, [ 18 F]FDG PET combined with MR imaging outperforms [ 18 F]FDG PET and MR imaging alone. , , The PET and MR imaging scans performed separately can be accurately and quickly coregistered with commercially available software.
[ 18 F]FDG PET/CT scan can be helpful to distinguish tumor recurrence after treatment from either pseudoprogression or radionecrosis. In the literature, the sensitivity and specificity of [ 18 F]FDG PET imaging to differentiate radionecrosis from WHO grade 3 and 4 progression range from 65% to 84% and 40% to 94%, respectively. , Previous PET examinations should always be compared for interpretation. Indeed, tumor uptake is expected to increase between 2 PET scans without treatment, while lesion uptake is expected to decrease after treatment.
Pseudoprogression and pseudoresponse can occur after treatment and present potential challenges in postradiation imaging.
- •
Pseudoprogression typically occurs within 3 months, but it can also occur during the first 6 months after therapy, and it is seen in 20% to 40% of cases. , , , It is more frequent in tumors with 6-O-methylguanine-DNA methyltransferase promoter methylation. Pseudoprogression was defined according to the Response Assessment in Neuro-Oncology (RANO) criteria as the appearance of new lesion or an increase in contrast enhancing areas, but these changes gradually faded or stabilized without any change of the treatment. According to the immunotherapy RANO criteria, the time window for pseudoprogression after immunotherapy is 6 months. Therefore, it is recommended for patients without significant clinical symptoms and evidence of early imaging progression within 6 months after immunotherapy to continue the immunotherapy before follow-up imaging confirms tumor progression. Pseudoprogression corresponds to an improved prognosis and survival. ,
- •
Pseudoresponse is characterized by a significantly decreased in tumoral enhancement posttreatment and is most frequently observed with antiangiogenic drugs such as bevacizumab (an anti-vascular endothelial growth factor [VEGF] antibody drug). Prognosis remains unchanged in pseudoresponse.
Radionecrosis can occur 6 months after radiation treatment and up to several years after. It occurs in approximatively 5% to 25% of all treated patients. The risk of radionecrosis increases with total radiation dose, irradiated volume, and additional chemotherapy. False-positive findings may occur due to necrotic reactions related to the treatment, which can exhibit increased glucose metabolism, leading to elevated [ 18 F]FDG uptake.
Radiolabeled amino acid PET imaging
Cells use AAs for protein synthesis. Neoplastic cells, due to their higher proliferative rate compared to normal cells, require more proteins for proliferation and thus exhibit increased AA transport. Radiolabeled AA PET imaging utilizes this facilitated transport and the subsequent accumulation, which is proportional to the cell proliferation rate. , AA PET imaging is recommended in conjunction with MR imaging for primary malignant brain tumors and metastases assessment. It can be used to differentiate tumor from nontumor processes, to delineate tumor extent, to guide biopsies (to target zones with the highest radiotracer uptake in low-grade gliomas and HGG), for prognosis, for baseline imaging before treatment, for postresection assessment, for radiation therapy planning, and to diagnose treatment-related changes versus progression or recurrence. , , Some studies outline that AA PET imaging could help identifying treatment responses better than MR imaging after radiotherapy and chemotherapy or bevacizumab in gliomas. AA PET imaging has a prognostic value in all-grade gliomas, especially with dynamic acquisition. , AA radiotracers are better than [ 18 F]FDG for low-grade gliomas since [ 18 F]FDG typically does not accumulate sufficiently in low-grade gliomas. Nevertheless, some gliomas (more often low grade) may be negative with AA PET.
A meta-analysis from Schlürmann and colleagues studied the utility of AA PET in the differential diagnosis of recurrent or progressive BM and treatment-related changes and found a pooled sensitivity and specificity of 82% and 84%, respectively.
[ 11 C]MET is the most studied AA agent, but it has a short half-life of 20 minutes making production only possible for centers with onsite cyclotron. , , [ 11 C]MET PET imaging has a sensitivity of 76% to 100% and a specificity of 75% to 100% for the diagnosis of suspected glioma. There are false-negative findings as some WHO grade 2 gliomas show no [ 11 C]MET uptake. There also are false-positive findings due to uptake in inflammatory or infectious processes. Because of the low background activity, [ 11 C]MET PET scan is preferred to [ 18 F]FDG PET scan to guide biopsy and delineate the tumor. Various TBR cutoff values have been utilized to predict tumor grade, ranging from 1.3 to 2.05. Due to the wide range of TBR values, the accuracy is limited as the TBR values overlap among WHO grade 2, 3, and 4 gliomas.
AA PET imaging tends to favor the use of [ 18 F]FET with its longer half-life of 110 minutes and by its additional well-characterized properties for both static and dynamic tumor imaging. , [ 18 F]FDOPA has received regulatory approval by the Food and Drug Administration for use in certain clinical settings, facilitating its use in clinical practice in the United States.
De Zwart and colleagues showed in their meta-analysis that [ 11 C]MET and [ 18 F]FET should be preferred, when available, over [ 18 F]FDG because of their higher sensitivity.
AA PET imaging using [ 18 F]FET or [ 11 C]MET is also a promising tool for pituitary neuroendocrine tumors assessment. [ 11 C]MET is the most studied agent, but [ 18 F]FET seems to have similar accuracy detecting functional pituitary neuroendocrine tumors. AA PET imaging value has been mainly investigated in Cushing’s disease. In the other functional pituitary adenoma, such as prolactinoma, acromegaly, and (TSH)-secreting pituitary adenomas only small case series are available, requiring further investigations in these entities.
Interpretation should be performed using visual assessment (higher uptake in pituitary lesion than normal pituitary tissue) after proper PET alignment and fusion with MR imaging, as currently no cutoff value for semiquantitative analysis has been determined.
Emphasis on O-(2-[ 18 F]-fluoroethyl)- l -tyrosine
FET is derived from tyrosine and is passively taken into cells by system L transporter (LAT) in exchange of leucine. , , LAT1 and LAT2 are overexpressed on the tumor cell membrane to have enough AAs for protein manufacturing. [ 18 F]FET uptake is nondependent of BBB disruption. The retention time in neoplastic tumor tissue is higher for FET than for other AA agents. [ 18 F]FET PET imaging is a useful tool for tumor extent assessment, tumor grading, identification of tumor progression versus pseudoprogression or radiation injury. , [ 18 F]FET uptake is correlated to prognosis. [ 18 F]FET has a lower uptake in inflammatory cells compared to [ 11 C]MET, but there are also false-positive findings due to inflammatory/infectious processes. Moreover, [ 18 F]FET uptake can be seen in vascular malformations. There are also false-negative findings for [ 18 F]FET as about 30% of WHO grade 2 gliomas can have a negative [ 18 F]FET PET scan. [ 18 F]FET PET imaging has a higher sensitivity (79%–98%) compared with [ 18 F]FDG (27%–50%) for the diagnosis of brain tumor versus nontumoral lesions when the specificity is comparable (37%–99% for [ 18 F]FET and 31%–99% for [ 18 F]FDG). ,
Unterrainer and colleagues studied the uptake characteristics in patients with newly diagnosed and untreated brain metastases using [ 18 F]FET PET imaging. They showed [ 18 F]FET uptake in most of the brain metastases, 40 of 45 metastases (89%) had a TBR max greater than 1.6 and were classified as [ 18 F]FET positive. Only a third of metastases inferior to 1.0 cm was [ 18 F]FET negative, most likely because of the scanner resolution and partial-volume effects. In metastases superior to 1.0 cm, [ 18 F]FET uptake intensity showed a high variability independent of tumor size.
Ouyang and colleagues’ meta-analysis shows that [ 18 F]FET PET imaging has a high accuracy for differentiating pseudoprogression from true progression, with a summary sensitivity of 80% and specificity of 81%. Higher sensitivity and specificity are found in the literature up to 100% and 91%, respectively. [ 18 F]FET PET imaging is highly performant in tumor recurrence identification versus radiation injury with an optimal cutoff value of TBR max greater than 2.55 and TBR mean greater than 1.95 (sensitivity = 83% and 86%, respectively; specificity = 85% and 88%, respectively).
Meta-analysis from Singnurkar and colleagues shows that [ 18 F]FET PET imaging seems to identify areas of tumor disease outside of conventional contrast-enhanced MR imaging with impact on radiation planning in 39% of cases. In glioma recurrence assessment, multiparametric [ 18 F]FET PET/MR imaging with dynamic FET parameters has the highest diagnosis accuracy of any individual modality.
TROG (Trans Tasman Radiation Oncology Group) 18.06 study currently ongoing evaluates the potential of [ 18 F]FET PET to impact adjuvant radiotherapy planning and distinguish between treatment-induced pseudoprogression versus tumor progression as well as prognostication.
Other tracers
FAPI
Fibroblast activation protein (FAP) is a type 2 membrane bound glycoprotein belonging to the dipeptidyl peptidase 4 family, and it is highly expressed in cancer-associated fibroblasts (CAFs) of several epithelial carcinomas. , The CAFs are different from normal fibroblasts as they have relative specific expression of FAP. Thus, targeting the FAP for imaging seems to be a promising strategy for the visualization of tumor stroma. FAP expression is found in more than 90% of epithelial tumors. FAP expression is reported to be associated with tumor cell migration, invasion, and angiogenesis and then with a worse prognosis. , FAP low expression in quiescent fibroblasts or in healthy adult tissues explain the low background signal in FAP imaging. Many fibroblast activation protein inhibitors (FAPI) labeled with gallium-68 or fluor-18 have been developed, for example, [ 68 Ga]Ga-FAPI-46 and aluminium-[ 18 F]fluoride (Al 18 F)-labelled 1,4,7‑triazacyclononane-N,N’,N″-triacetic acid (NOTA)-conjugated FAPI-74. FAPI tracers have gained attention for their selective binding, rapid and fast clearance from circulation, making them favorable for imaging purposes. These features contribute to their potential utility in various types of tumor imaging, including brain tumors. Brain tissue demonstrates minimal radiolabeled-FAPI uptake, with a pooled SUV mean of 0.05 ± 0.05, attributable to the restricted passage of FAPI across the BBB. This restriction may impede the detection and characterization of brain tumors in cases where the BBB remains intact.
In 2019, Röhrich and colleagues showed the elevated FAPI uptake and TBR in IDH-wild type glioblastomas and grade 3 or 4, while observing no significant uptake in grade 2, IDH-mutant gliomas. They concluded that FAPI PET imaging allows noninvasive distinction between low-grade IDH-mutant and HGG. Windisch and colleagues found that FAPI PET imaging for target volume delineation resulted in gross tumor volumes containing volume not covered by MR imaging. FAPI PET may yield false positives, as seen in progressive multifocal leukoencephalopathy, which can exhibit multifocal FAPI uptake (SUV max : 2.2–4.2) and tuberculosis meningitis, where FAPI accumulation may mimic BM. Various ongoing clinical trials are currently investigating FAPI PET imaging and the theragnostic possibilities.
1-amino-3- 18 F-fluorocyclobutane-1-carboxylic acid
Synthetic nonmetabolized leucine-derivate anti-1-amino-3- 18 F-fluorocyclobutane-1-carboxylic acid ( 18 F-Fluciclovine, [ 18 F]FACBC) accumulates in prostate cancer tumor cells, but also in other cell types. Beyond prostate cancer, [ 18 F]FACBC has also demonstrated to be highly accumulated in other solid tumors, including high-grade gliomas. [ 18 F]FACBC is transported into glial cells by both l -AA transporters (especially LAT1) and by alanine–serine-cysteine transporters (especially ASCT2). They are up regulated and activated in glioma cells but are less expressed in healthy brain cells and inflammatory cells. [ 18 F]FACBC PET/CT or PET/MR imaging showed a good safety profile and a good diagnostic accuracy for high-grade gliomas detection before and after treatment, according to Castello and colleagues meta-analysis. It is reported that normal brain parenchyma had no significant uptake of [ 18 F]FACBC, resulting in good image contrast for high-grade gliomas detection. Moreover, [ 18 F]FACBC uptake increased with tumor grade and proliferative activity in gliomas, allowing for differentiation between high-grade and low-grade gliomas. For high-grade glioma identification, the sensitivity of [ 18 F]FACBC PET imaging ranged between 85.7% and 100% (pooled estimate of 92.9%), while the specificity ranged from 50% to 100% (pooled estimate of 70.7%). There are false positives as focal increased uptake can be seen in pituitary adenoma, meningioma, inflammatory, and infectious processes.
Prostate-specific membrane antigen labeled with gallium-68
Prostate-specific membrane antigen (PSMA) labeled with gallium-68 has shown promising uptake in glioblastoma, particularly when comparing uptake in lesions labeled as radiation necrosis, which typically show no PSMA uptake. , A prospective study by Kumar and colleagues, which included 30 patients with recurrent grade 3 and 4 gliomas and a total of 49 lesions detected by MR imaging, along with 3 treated patients with MR imaging-proven radionecrosis as negative controls, increased [ 68 Ga]PSMA-11 uptake was observed in all the 49 lesions. Semiquantitative assessment of uptake in the recurrent lesions revealed a median SUV max and SUV mean values of 6.2 and 3.3, respectively. Ratios of both SUV max and SUV mean of lesion to background uptake were high allowing an excellent detection rate. The 3 patients with radiation necrosis on MR imaging did not have significant PSMA uptake. This shows the potential of [ 68 Ga]PSMA PET imaging to differentiate tumor recurrence from radiation necrosis in the post-therapy setting.
[ 68 Ga]Ga-PSMA-617 has a lower nonspecific affinity to the kidneys compared to [ 68 Ga]Ga-PSMA-11, and preliminary clinical results show high uptake into high-grade gliomas. These characteristics make PSMA-617 one of the best PSMA-targeting ligand candidates for theragnostic applications. The prospective study by Brighi and colleagues, which included 10 patients with recurrent gliomas for the comparison of [ 68 Ga]Ga-PSMA-617 and [ 18 F]FET PET imaging showed that [ 68 Ga]Ga-PSMA-617 accumulates in large parts of the tumor that extend beyond the [ 18 F]FET-avid margins. That suggests that [ 68 Ga]Ga-PSMA-617 might accumulate in regions of early neoangiogenesis. Validating this hypothesis in a larger cohort of patients through histologic analysis would support that [ 68 Ga]Ga-PSMA-617 might be a better imaging biomarker for delineation of progressing tumor volume.
The theragnostic concept is now well known in nuclear medicine. Theragnostic is the use of a radiotracer for diagnosis purpose and for lesion expression assessment and then combining the same tracer with a high-dose radioactive agent to destroy tumor cells, which is named radioligand therapy. It is now routinely used in prostatic cancer with [ 177 Lu]Lu-PSMA-ligands. With this concept, Kumar and colleagues , treated one of their patients with recurrent glioma with [ 177 Lu]PSMA-617 therapy. It showed good partial response and decrease in the volume of the tumor. Further studies are required to evaluate PSMA-targeted therapy efficacy in gliomas.
The advantages and limitations of those radiotracers are listed in Table 1 .
Radiotracers | Advantages | Limitations |
---|---|---|
[ 18 F]FDG |
|
|
Amino acid analogs |
|
|
FAPI |
|
|
[ 18 F]FACBC |
|
|
PSMA ligands |
|
|
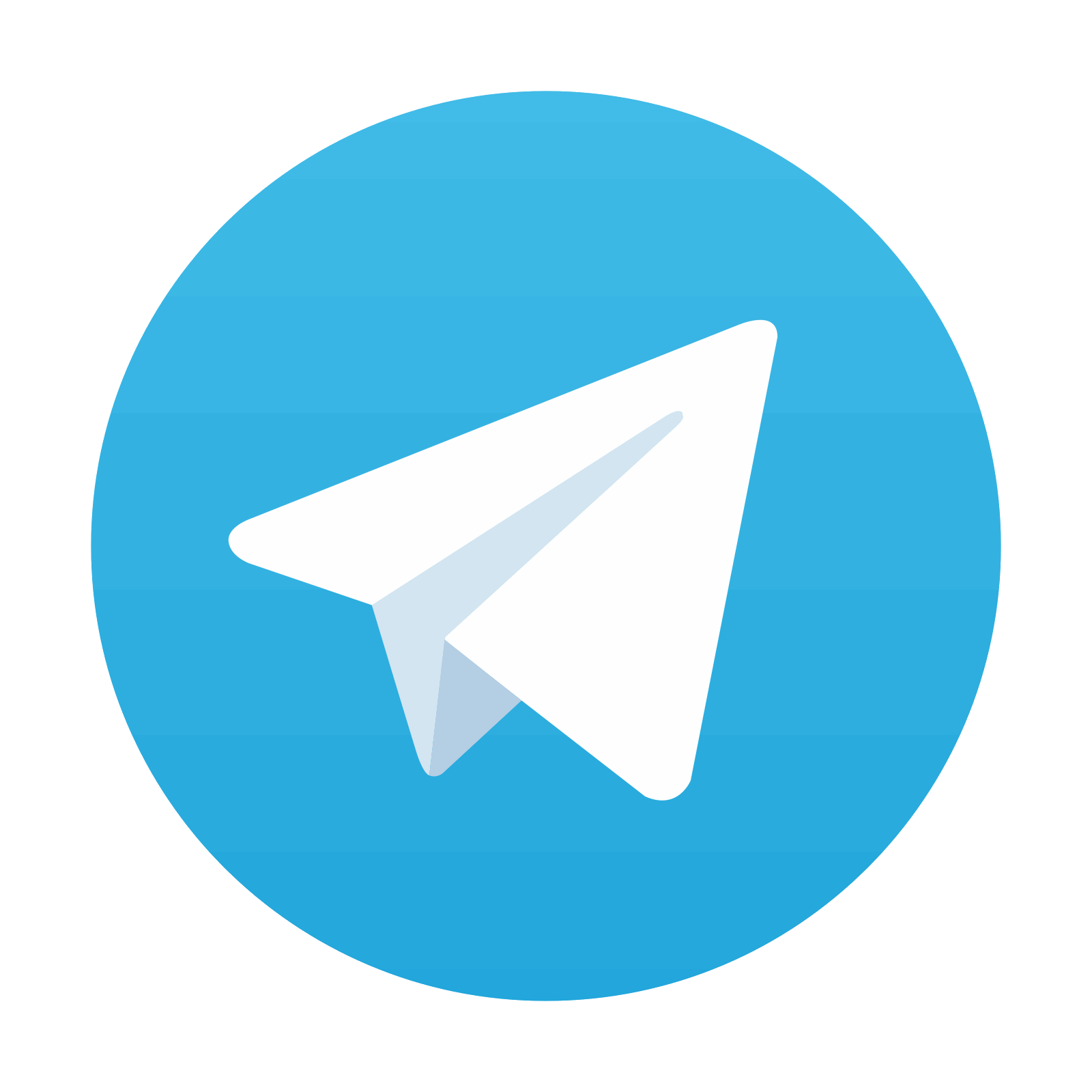
Stay updated, free articles. Join our Telegram channel

Full access? Get Clinical Tree
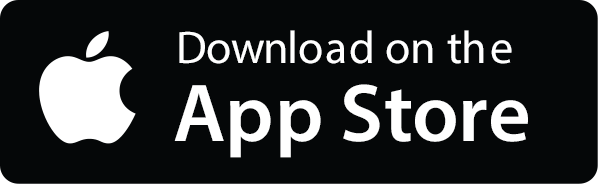
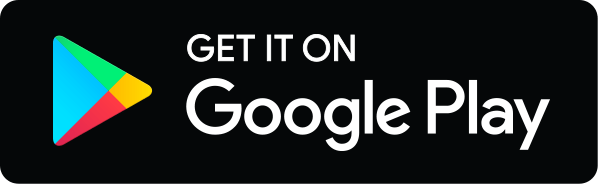
