Abstract
This chapter covers CNS applications of nuclear medicine. The various radiopharmaceuticals used for perfusion, metabolism, and receptor imaging are discussed, as well as issues about the imaging procedures and protocols.
Specific clinical applications, including evaluation of brain death, cerebrovascular disease, neoplasms, radiation necrosis, epilepsy, and dementia, are presented. Also included is evaluation of CSF abnormalities.
Keywords
brain perfusion, metabolism imaging, cerebrovascular disease, neoplasms, neuroreceptor imaging, dementia, brain death, epilepsy, radiation necrosis, CSF
Radionuclide Brain Imaging
In specific clinical settings, radionuclide planar, single-photon emission computed tomography (SPECT), or positron emission tomography (PET) brain imaging can provide valuable functional and perfusion information about suspected cerebral abnormalities or cerebrospinal fluid (CSF) dynamics that is not obtained through anatomic imaging. In the normal cerebrum, passage of most substances from the cerebral capillaries into the extravascular space is severely restricted, constituting what is referred to as the blood-brain barrier . The degree of permeability of this barrier varies with the nature of the material attempting to pass and with the numerous complex carrier mechanisms used to facilitate or hinder passage through the cell membranes involved.
The most common nuclear medicine imaging procedures of the brain can be divided into three different approaches relative to this principle:
- •
Planar brain imaging, which generally uses radiopharmaceuticals that are perfusion agents and do not routinely cross an intact blood-brain barrier. Planar imaging is usually performed for brain death studies only.
- •
SPECT brain perfusion imaging, which uses lipophilic radiopharmaceuticals that routinely cross the blood-brain barrier to localize in normal brain tissue and pathologic processes in proportion to regional cerebral blood flow.
- •
PET metabolic and receptor brain imaging, which uses functional positron-emitting radiopharmaceuticals, such as radiolabeled fluorodeoxyglucose (a glucose analog that reflects regional glucose metabolism) and neuroreceptor agents.
Planar Brain Perfusion Imaging
Technique
Planar radionuclide cerebral imaging generally consists of two phases: (1) a dynamic or angiographic study composed of rapid sequential images of the arrival of the radioactive bolus in the cerebral hemispheres, which essentially constitutes a qualitative measure of regional brain perfusion; and (2) delayed static images. The most common application of planar technique is in the setting of suspected brain death. Most brain scans are performed with either a transient perfusion agent (technetium-99m [ 99m Tc]–diethylenetriamine pentaacetic acid [DTPA], 99m Tc-pertechnetate) or a lipophilic perfusion agent that is extracted by the brain on the first pass ( 99m Tc–hexamethylpropyleneamine oxime [HMPAO] and 99m Tc–ethylene l-cysteinate dimer [ECD]). A sample protocol giving details of the technique and associated radiation doses are given in Appendix E .
Normal Planar Brain Scan
Normally, there is prompt symmetric perfusion to the brain that in the anterior projection looks similar to a trident. The middle cerebral arteries are seen to the right and left, and the anterior cerebral arteries are seen as a single midline vertical line of activity. Perfusion should extend to the calvarial convexities bilaterally ( Fig. 3.1 ). Although symmetry is the hallmark of the arterial-capillary phase of a normal perfusion scan, asymmetry in the venous phase is common because of variations in venous anatomy. Care should be taken not to overinterpret lack of symmetry in the venous phase in the absence of an arterial abnormality.
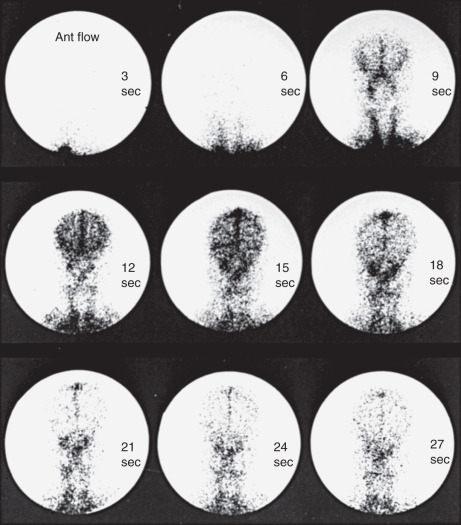
On the static images of a 99m Tc-DTPA or 99m Tc-pertechnetate scan, radioactivity does not normally lie within the brain itself because of the integrity of the blood-brain barrier, but rather is located in the overlying scalp soft tissues, calvarium, and subarachnoid spaces that outline the cerebral hemispheres. Activity is also seen in the larger blood pool accumulations, such as the sagittal and transverse sinuses. Thus, the normal static brain images include a number of consistent landmarks ( Fig. 3.2 ). On the posterior view, the transverse sinuses are generally symmetric, although it is not uncommon for the right sinus to be dominant. On the lateral views, activity in the suprasellar and sylvian regions is noted, although it is less constant and less well defined than activity in the venous sinuses.
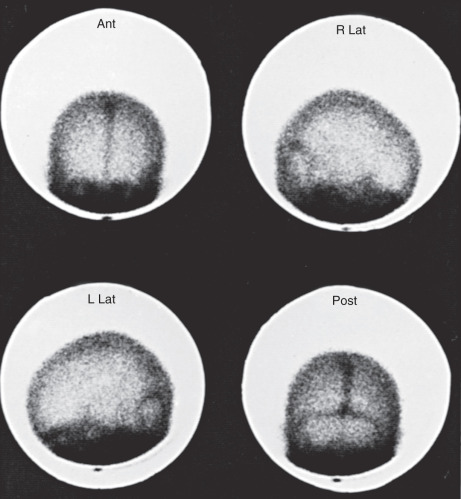
In contrast to 99m Tc-DTPA or 99m Tc-pertechnetate imaging, normal static planar images obtained with a first-pass extraction perfusion agent ( 99m Tc-HMPAO, 99m Tc-ECD) will demonstrate activity in the brain substance (primarily gray matter) ( Fig. 3.3 ).
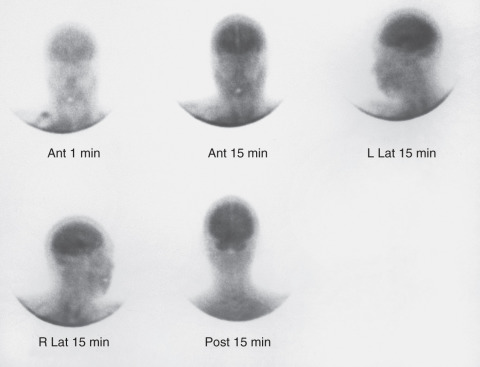
SPECT and PET Brain Imaging
Radiopharmaceuticals
Although planar brain perfusion imaging is usually limited to compounds that enter the brain substance only when there is disruption of the normal blood-brain barrier, SPECT brain perfusion imaging uses several lipophilic radiopharmaceuticals. These radiopharmaceuticals cross the intact blood-brain barrier and are retained by the brain tissue in proportion to regional cerebral blood flow (rCBF). They thus map the distribution of brain perfusion in both normal and pathologic brain tissue. These agents include 99m Tc-HMPAO (exametazime) and 99m Tc-ECD (bicisate).
99m Tc-HMPAO ( 99m Tc exametazime or Ceretec) is a lipophilic agent that crosses the blood-brain barrier with rapid first-pass uptake. Once in the brain substance, HMPAO is metabolized to a hydrophilic form that cannot diffuse out of the brain. Uptake in the brain peaks several minutes after injection. About 5% of the injected activity localizes in the brain, with no significant late redistribution. Activity of 99m Tc-HMPAO is highest in gray matter and is proportional to rCBF. Because it may be unstable in vitro, 99m Tc-HMPAO should be injected within 30 minutes after its preparation, although a stabilized form is available that can be used up to 4 to 6 hours after preparation.
99m Tc-ECD (bicisate or Neurolite) has uptake and redistribution properties similar to HMPAO. 99m Tc-ECD is rapidly localized in a normal brain in proportion to rCBF, with slow clearance. It is retained in the brain tissue by rapid deesterification to a polar metabolite that does not recross the blood-brain barrier and therefore maintains residence within the brain tissue. Thus there is no intracerebral redistribution. A high ratio of gray to white matter that persists over time is identified. Intracerebral activity peaks several minutes after administration, with about 6% of the dose localizing within the brain. Although similar to 99m Tc-HMPAO, 99m Tc-ECD demonstrates more rapid clearance from the blood pool, thus reducing background activity and increasing target to background. It also demonstrates better chemical stability with a longer post-preparation shelf life of 6 hours.
99m Tc-ECD and 99m Tc-HMPAO are injected intravenously using 10 to 20 mCi (370 to 740 MBq). SPECT images are obtained 15 to 20 minutes after injection. External sensory stimuli, such as pain, noise, and light, as well as patient motion, affect rCBF. Therefore these, along with cognitive functions such as reading, should be minimized at the time of injection and localization to prevent interfering increased activity in the corresponding sensory cortex. For a similar reason, the intravenous access should be placed 5 minutes before the radiopharmaceutical is administered.
Thallium-201 chloride is used for SPECT imaging in the differential diagnosis of recurrent tumors versus radiation necrosis. Very little thallium is concentrated in normal or necrotic brain tissue, and an increase in thallium indicates the presence of viable tumor.
More recently, the SPECT molecular imaging agent 123 I-Ioflupane (DaTscan) has become available to demonstrate the loss or dysfunction of presynaptic dopaminergic neurons. This can be used to better differentiate patients with essential tremor from those with presynaptic Parkinsonism syndromes.
The most commonly employed radiopharmaceutical for PET imaging of the brain in the United States is 18 F-fluorodeoxyglucose (FDG). Uptake is reflective of regional glucose metabolism and not regional blood flow. Areas of the brain stimulated by activity during 18 F-FDG injection and uptake show relatively increased metabolism. These include the visual (occipital) or auditory cortical areas in visually or auditorally stimulated patients, language centers in talking patients, and the motor cortex in moving patients. Thus injection and uptake of 18 F-FDG are best accomplished in silent, motionless patients in a quiet, darkened room. However, it is important that the patient remain awake with eyes open because uptake of FDG with the eyes closed may result in less than baseline stimulation of the visual cortex and present as an area of hypometabolism in the occipital lobe, as may be seen in dementia with Lewy bodies (DLB). Certain drugs may alter global and/or relative regional brain metabolism, including sedatives, antiepileptic and neuroleptic drugs, and barbiturates. Of course, various disease states can also cause either a decrease or increase in FDG accumulation in the brain. Other PET agents include specific amyloid plaque radiopharmaceuticals for assessment of dementias. Details of suggested techniques and radiation doses are shown in Appendix E .
Normal SPECT Perfusion Brain Scan
The normal distribution of lipophilic brain perfusion agents is proportional to regional blood flow, with significantly greater activity seen in the cortical gray matter. This is consistent with the fourfold greater blood flow in the gray matter than in the white matter. Thus activity is symmetric and greatest in the strip of cortex along the convexity of the frontal, parietal, temporal, and occipital lobes. Activity is also high in the regions corresponding to subcortical gray matter, including the basal ganglia and the thalamus. The cortical white matter has substantially less activity, and the border between white matter and ventricles may be indistinct. Although high-resolution images obtained with dedicated multidetector cameras display greater anatomic detail, the primary purpose of SPECT imaging is to evaluate relative rCBF rather than structural detail.
SPECT Brain Perfusion Image Interpretation
The cerebral perfusion images should be inspected for symmetry of radiopharmaceutical distribution and for continuity of perfusion in the rim of cortical gray matter. In general, local perfusion is measured as increased, similar, or decreased, relative to the perfusion in the identical area in the contralateral cerebral hemisphere. Pathologic processes that alter local brain perfusion produce areas of increased or decreased activity, depending on the changes in blood flow relative to the normal adjacent brain tissue. Because the anatomic detail of the images is limited, precise localization of an abnormality is greatly improved using SPECT-computed tomography (CT) with coregistration of images leading to more confident image interpretation.
PET/CT Image Interpretation
18 F-FDG is the most commonly employed radiopharmaceutical for PET/CT of the brain and permits the noninvasive in vivo quantification of local cerebral metabolism and, unlike CT or magnetic resonance imaging (MRI), provides a physiologic test that may illustrate pathologic conditions before morphologic manifestations are discernible. PET metabolic imaging has significant usefulness in certain discrete clinical settings and can be used to evaluate refractory seizure disorders, dementia, and recurrent brain tumors.
The normal distribution of 18 F-FDG in the brain is of highest intensity in the basal ganglia (especially the putamen and caudate nucleus) and thalami ( Fig. 3.4 ), followed closely by uptake in the cortical gray matter. Normal white matter shows very low uptake. This pattern changes with aging, and significant variations in cortical uptake have been noted. Relatively decreased frontal lobe metabolism with normal aging is not uncommon. Metabolism in the thalami, basal ganglia, cerebellum, and visual cortex is generally unchanged with normal aging.
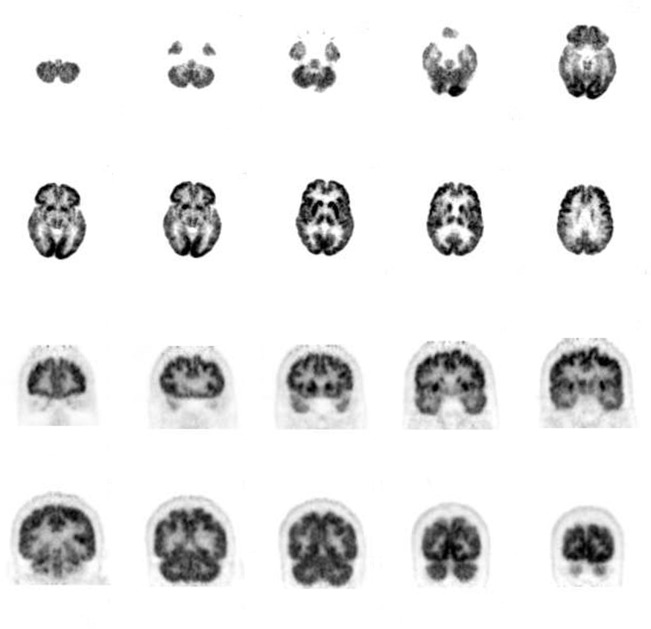
Certain areas of the cerebral cortex can normally be focally hypermetabolic compared with the remainder of the cortex. These include the posterior cingulate cortex (anterior and superior to the occipital cortex), a focus in the posterior superior temporal lobe (Wernicke region), the frontal eye fields (anterior to the primary motor cortex and may be asymmetric), and a symmetric area of increased activity in the posterior parietal lobes. The degree of uptake in the cerebellar gray matter is less on an FDG PET study than on a SPECT perfusion scan.
Although primary visual analysis of PET/CT images remains a mainstay in interpretation, the use of computer algorithms employing ROI and voxel-based approaches, which are more quantitative and less operator-dependent, to measure subtle differences in metabolic or other functional changes is becoming more common. This is often crucial in the application of PET imaging for the early diagnosis of and differentiation among complex neurodegenerative diseases, such as the dementias with their often subtle findings.
Clinical Applications
Brain Death
Radiopharmaceuticals used for evaluation of suspected brain death may be suitable either for planar or SPECT imaging ( Table 3.1 ). The planar radionuclide angiogram using ≤30 mCi (1110 MBq) of 99m Tc-DTPA or 99m Tc-pertechnetate is a simple, noninvasive method of determining the presence or absence of intracerebral perfusion and thereby offering confirmatory evidence of a clinical impression of brain death. To prevent mistaking scalp perfusion for intracerebral blood flow, an elastic band can be placed around the head just above the orbits to diminish blood flow to the overlying superficial scalp vessels. Imaging should begin before the appearance of the radiopharmaceutical in the common carotid arteries and end after the venous phase is complete. Patients should not have recently received high levels of barbiturates, as this may decrease cerebral blood flow.
Radiopharmaceutical a | Dose | Flow | Static | SPECT |
---|---|---|---|---|
Nonlipophilic 99m Tc DTPA 99m Tc Pertechnetate | Adult: ≤ 30 mCi Child: 0.3 mCi/kg (~ 5 mCi minimum) | Mandatory Anterior 1s/frame × 1 min | Immediate Planar Ant, Lat | N/A |
Lipophilic 99m Tc ECD 99m Tc HMPAO | Adult: ≤ 30 mCi Child: 0.3 mCi/kg (~ 5 mCi minimum) | Recommended Anterior 1s/frame × 1 min | 20-min delay Planar Ant, both Lats | As needed |
In the presence of cerebral death, the injected activity typically proceeds through the carotid artery to the base of the skull, where the radioactive bolus stops ( Fig. 3.5 ). As with all radionuclide arteriograms, a technically adequate study is mandatory with injection of a good bolus. The absence of intracerebral flow is strong corroborative evidence of cerebral demise, but is not in itself sufficient to make the diagnosis. Generally, a single anterior or lateral cerebral view is obtained within 5 to 10 minutes of the completion of the angiographic portion of the study to determine the presence of any sagittal sinus activity. The significance of low-level sagittal sinus activity without an obvious arterial phase is somewhat controversial, as it may represent activity from scalp vessels draining into the sinus or a small amount of intracerebral flow. Such activity may be seen in as many as 50% of patients with confirmed brain death. In cases of head trauma, hyperemic blood flow to injured scalp structures may mimic brain blood flow or superior sagittal sinus activity. In any case, most patients without confirmed intracerebral perfusion on the angiographic arterial phase have a grave prognosis and the presence of slight dural sinus activity does not in itself contradict the diagnosis of brain death.
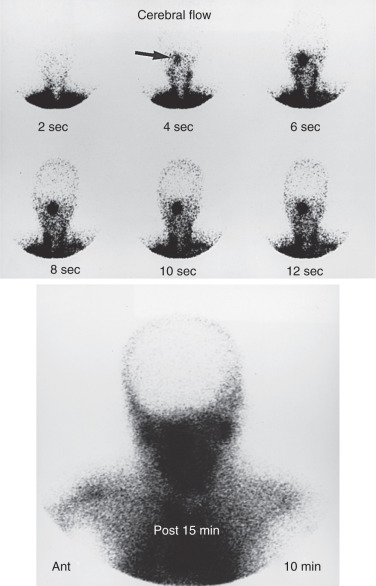
When intracranial carotid blood flow ceases in the setting of brain death, increased or collateral flow through the maxillary branch of the external carotid artery may produce markedly increased perfusion projecting over the nasal area in the anterior view, as seen on the radionuclide angiogram and subsequently on static images. This so-called “hot-nose” sign cannot be used specifically to indicate brain death, but it may be used as a secondary sign when intracerebral perfusion is absent. This sign may also occur with a generalized decrease of cerebral perfusion from various causes, including severe cerebrovascular or carotid occlusive disease or increased intracranial pressure of any cause.
If clinical evaluation of the patient suggests brain death and no cerebral perfusion is demonstrated on the radionuclide study, brain death is virtually certain. Although an actual diagnosis of brain death should not be made by using nuclear imaging techniques alone, these techniques are important supportive evidence of such a diagnosis in the proper clinical settings ( Box 3.1 ).
- •
If components of neurologic examination cannot be performed
- •
If uncertain neurologic examination result is present
- •
If apnea test cannot be performed
- •
To shorten duration of observation period
- •
If uncertain medication effect is present
- •
If helpful for family members to better comprehend diagnosis
Radiopharmaceuticals used for SPECT brain perfusion imaging ( 99m Tc-ECD and -HMPAO) may also be used for cerebral angiography in the same manner as conventional brain imaging agents. Absence of perfusion on the angiographic phase and lack of cerebral activity on subsequent static planar or SPECT images are confirmatory of a clinical diagnosis of brain death. Advantages over conventional 99m Tc-pertechnetate or 99m Tc-DTPA imaging are conferred by the ability to perform static planar or SPECT imaging 20 minutes after injection, which renders the examination less dependent on the radionuclide angiographic phase, including bolus adequacy and the problems associated with interfering superficial scalp blood flow and sagittal sinus activity.
Brain death studies should be reported by addressing the presence or absence of observed intracerebral blood flow. If perfusion is severely decreased, but not completely absent, a repeat follow-up study may be warranted.
Cerebrovascular Disease
SPECT brain perfusion imaging has been demonstrated to be of value in the diagnosis and prognosis of cerebrovascular disease manifested by transient ischemic attacks (TIAs), acute cerebral infarction, and intracranial hemorrhage.
Cerebral Infarction
SPECT brain perfusion imaging is more sensitive than CT and MRI in detecting cerebral ischemia during the first hours of stroke. Only about 20% of CT scans are positive 8 hours after cerebral infarction, whereas 90% of SPECT brain perfusion images show deficits. By 72 hours, however, the sensitivity of the two examinations is about equal. Sensitivity of SPECT brain perfusion imaging is significantly affected by the size of the infarct. Small infarcts, particularly those in the white matter (lacunar infarcts), may not be detected with SPECT or PET. Acute infarcts are usually identified on non-contrast MRI within 4 to 6 hours. In addition, SPECT and PET brain imaging cannot distinguish between hemorrhagic and ischemic infarction, which is critical in the early stages of evaluation and treatment.
During the acute phase of stroke (first hours to 2 to 3 days after vascular insult), a reduction in blood flow to the affected area is identified ( Fig. 3.6 ). The area of decreased perfusion on SPECT imaging may be greater than that seen with CT imaging, suggesting tissue at risk (ischemic penumbra) surrounding the infarct.
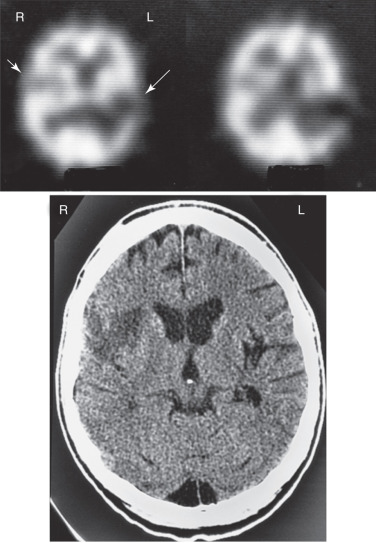
In the subacute phase of stroke (1 to 3 weeks after onset), the brain SPECT perfusion pattern is complicated by the phenomenon of increased, or “luxury,” perfusion; that is, the blood supply is greater than is metabolically required because the cells are already dead or dying ( Fig. 3.7 ). This phenomenon may decrease the sensitivity of SPECT perfusion imaging in the subacute phase of stroke.
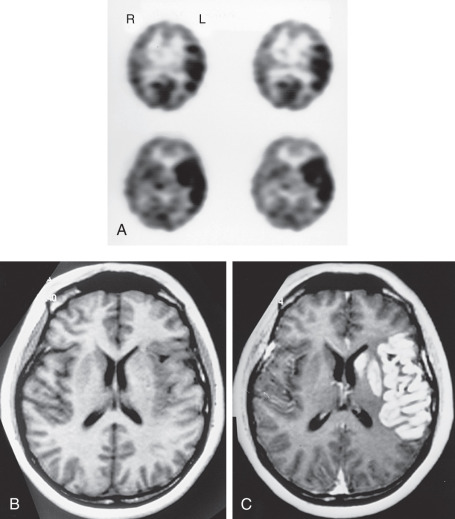
Prognostically, patients displaying improvement of perfusion during the first week after infarction display a greater chance of recovery of neurologic function than do those whose perfusion improves at a later time. Post-treatment regional hypoperfusion observed by 99m Tc-HMPAO SPECT is associated with a poor outcome, whereas hyperperfusion after treatment predicts symptom improvement but is not related to development of intracerebral hemorrhage
In the chronic phase (≥1 month after symptom onset), luxury perfusion has generally subsided, and the perfusion deficits seen on SPECT imaging stabilize. Except for monitoring improvement and serving as comparisons for future studies, SPECT brain imaging is of limited use in the chronic phase of stroke.
During the acute and subacute phases of stroke, crossed-cerebellar diaschisis (seen primarily with cortical strokes) is a common phenomenon and should not be confused with primary cerebellar ischemia or other pathology (see following).
Transient Ischemic Attack
The sensitivity for detecting localized cerebral ischemia associated with TIA is time sensitive, but most can be differentiated from ischemic strokes by SPECT within 6 hours of symptom onset. Sixty percent of these perfusion deficits are detected in the first 24 hours, but less than 40% are detected 1 week after the insult. In addition, hypoperfusion duration is variable and may persist even after symptoms have resolved. Early SPECT findings correlate well with the severity of neurologic deficits and their clinical outcome, with early severe hypoperfusion predicting a poor outcome. PET performed within 5 to 8 hours of stroke onset has shown to be even more reliable for predicting outcome and may indicate critical ischemia that may be responsive to therapy
Carotid Stenosis
Most patients with TIAs or carotid stenoses do not display cortical perfusion defects without pharmacologic intervention. A simple method for evaluating the adequacy of cerebrovascular reserve is to assess brain perfusion response to pharmacologic cerebrovascular vasodilatation using acetazolamide (Diamox), a carbonic anhydrase inhibitor, in conjunction with SPECT brain perfusion imaging. In normal patients, cerebral blood flow increases threefold to fourfold with use of Diamox. In areas in which regional perfusion reserve is diminished because autoregulatory vasodilatation distal to a stenosis is already maximal, a relative Diamox-induced regional perfusion defect is identified on SPECT brain perfusion images compared with the surrounding normal regions, which increase in perfusion (and thus activity) compared with baseline images obtained without Diamox intervention ( Fig. 3.8 ).
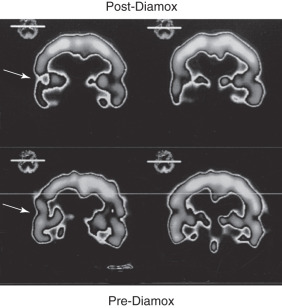
Brain Tumors
Both primary and metastatic brain lesions present on SPECT brain perfusion imaging as localized defects that correspond to the mass lesions. This technique alone is of limited value in the primary diagnosis or evaluation of intracranial mass lesions. In conjunction with thallium-201 ( 201 Tl), however, SPECT brain perfusion imaging may be valuable in distinguishing between radiation necrosis and tumor recurrence in patients with malignant gliomas treated with high-dose radiation. The study may also localize suspected recurrences for biopsy.
In the differentiation of recurrent malignant glioma from radiation necrosis, 99m Tc-HMPAO images generally show a focal defect in the region of abnormality, whether containing necrotic tissue, recurrent tumor, or both. 201 Tl activity, however, is a marker of viability, localizing in living tumor cells but not in nonviable tumor cells or necrotic tissue. 201 Tl activity may be graded as low (less than scalp activity), moderate (equal or up to twice scalp activity), or high (greater than twice scalp activity) ( Fig. 3.9 ). A high degree of increased thallium activity in the region of a 99m Tc-HMPAO defect is indicative of tumor recurrence, whereas a low degree is consistent with postradiation necrosis. Careful attention to study acquisition and processing is needed to compare identical areas between the two SPECT studies and with correlative CT or MRI scans.
