Abstract
Objective
To investigate the effects of ultrasound treatment on the healing of hip bone fractures using frequencies of 0.5 MHz and 1.5 MHz with constant intensity (30 mW/cm 2 ) at the fractured site.
Methods
For the ex vivo experiments, acoustic attenuations of 0.5 MHz and 1.5 MHz ultrasound were measured and compared using different thicknesses of human cadaver and porcine tissues in a hydrophone system. For the in vivo experiments, 20 hip-fractured rabbits were divided into four groups, namely: control, 1.5 MHz with unchangeable intensity (positive control), 0.5 MHz with changeable intensity, and 1.5 MHz with changeable intensity. For the 0.5 and 1.5 MHz groups with changeable intensity, a constant intensity of 30 mW/cm 2 at the fracture site was achieved using a compensation method for power transmission with reference to the acoustic attenuation.
Results
The effective intensity measured using a hydrophone was substantially reduced to 6.16 mW/cm 2 from 30 mW/cm 2 in the positive control device after propagating soft tissues with a thickness of 5.0 cm, with an attenuation of approximately 6.0 dB. Meanwhile, for the 0.5 and 1.5 MHz groups, the ultrasound intensity was consistently controlled at 30 mW/cm 2 after passing through tissues with different thicknesses using the compensation method. In the in vivo study using a newly established hip fracture rabbit model, the best results in bone histomorphometry, mechanical properties, and histological evaluation were consistently found in the 0.5 MHz group, while the 1.5 MHz group exhibited relatively better bone healing than the positive control group.
Conclusion
The results suggest a LIPUS frequency of 0.5 MHz together with the consistent intensity of 30 mW/cm 2 at the fracture site for more effective treatment of hip bone fractures.
Introduction
Hip fractures are life-threatening injuries for older people and are treated as public health concerns in many countries [ ]. The annual global incidence of hip bone fracture is currently about 1.6 million [ ], which is estimated to increase to 6.26 million by 2050 [ ]. Hip fractures are usually the result of impact from falling, while factors such as smoking, ethnicity, age, and medical conditions such as osteoporosis, may weaken the bones, increasing their susceptibility to fracture on impacts. The healing of hip fractures generally takes a long time whilst incurring high medical costs [ ], and there is an increased risk of fracture-related complications due to the complex morphology of the hip bones. LIPUS has been reported to be a safe and effective approach to accelerate the bone healing process as it has been found to enhance bone healing at all stages, specifically, the inflammatory, reparative, and remodeling stages [ ]. An FDA-approved, commercially available LIPUS device has been used in previous studies on fracture healing. However, there have been no investigation into the benefits of LIPUS for hip fractures. The effectiveness of LIPUS in cases with deeper fracture sites (femur and humerus) was found to be inferior compared with fractures in superficial bones (tibia, fibula, radius, ulna) [ ]. Fracture sites in the hip or acetabulum are painful and take longer time to heal and can become a life-long burden in elderly people. Since most studies have investigated superficial bone fractures using LIPUS, the demonstration of whether it is less efficacious in deeper fracture sites and how to make it more effective requires more investigation [ ].
Commercial LIPUS devices use a fixed set of parameters with an ultrasound frequency of 1.5 MHz, 20% duty cycle of burst wave pattern (200 µs on and 800 µs off) with a pulse repetition frequency of 1 kHz, and spatial average temporal average (I SATA ) intensity of 30 mW/cm 2 10 . During the 1930s–1950s, the usage of ultrasound intensity ranging from 5000 to 25000 mW/cm 2 for bone fracture healing caused several complications in the soft tissues, which led to the use of decreased ultrasound intensity (200 mW/cm 2 ) [ ]. Subsequently, numerous human and animal studies reported that an intensity of 30 mW/cm 2 accelerated both bone formation and reduction of healing time in fresh fractures, delayed unions, and nonunions [ , ]. However, very few studies have investigated its use for deeper bone fractures such as the hip bone. Hence, we selected this intensity in the present study. Ultrasound is an acoustic wave that can be attenuated with some energy absorbed by soft tissues [ , ], determined by the attenuation coefficient α with unit dB/cm/MHz which is associated by the type of soft tissue and ultrasound frequency [ ]. Given this factor, it seems not to be reasonable to use the same parameter setting of the commercial device for deeper fractures where various soft tissues with different thicknesses covering the bone.
In this study, a customized compensation method was applied to maintain an acoustic intensity of 30 mW/cm 2 at the fractured site by referring to other uses of deeply targeted ultrasound stimulation, which makes this as the first study to demonstrate the effect of ultrasound on hip fractures in an animal model . The objective was not to identify the best parameter but to demonstrate that LIPUS can be more effective by compensating for the attenuation caused by the soft tissues covering the fracture location, as well as by lowering the frequency from the conventionally used 1.5 MHz to 0.5 MHz. In this study, the thickness of the soft tissue was hypothesized to be the key factor affecting fracture healing using LIPUS, and the effectiveness of fracture healing may be dependent on the effective intensity (30 mW/cm 2 ) of the LIPUS reaching the fracture site. Hence, different thicknesses of human cadaver and porcine tissues were first used to assess the attenuation of the ultrasound after propagation through the tissues. Soft tissue immediately anterior to the superior iliac spine and acetabular rim was collected from a human cadaver, while and porcine tissues were collected from the thigh region of the pig . The use of tissue samples from a human cadaver can help to recognize actual changes in acoustic intensity in human tissues, while the porcine tissues were used to evaluate changes in attenuation in different tissue thicknesses. According to previous studies, porcine tissues have very similar properties to human tissues and have been widely utilized in studies of musculoskeletal biomechanics [ ]. In the present study, a portable customized ultrasound stimulator was developed to allow adjustments of ultrasound frequency and output intensity. The output intensity of the customized stimulator could be increased to ensure an intensity of 30 mW/cm 2 after passing through a layer of soft tissue, thus compensating for ultrasound attenuation caused by the soft tissue layer. Moreover, an ultrasound frequency of 0.5 MHz was hypothesized to be more effective for healing fractures of the hip bone, as ultrasound with a lower frequency can more easily penetrate deeper into the fracture site due to its lower attenuation [ ]. There is currently a lack of experimental data about the penetration characteristics of LIPUS for hip fracture healing with frequencies lower than 1.5 MHz. The results of this study on the use of frequencies of 0.5 and 1.5 MHz together with the controlled intensity at the fracture surface may provide a direct reference for the future investigation of LIPUS for the healing of deep bone fractures.
Materials and methods
LIPUS devices and stimulation pattern
Two LIPUS stimulators were used in this study; one was a commercial LIPUS stimulator (EXOGEN 4000+, Bioventus LLC, USA), while the other was our customized stimulator, as shown in Figure 1 (a–b). The customized stimulator is a portable device that includes a signal generator, a 50 W power amplifier, and removable unfocused ultrasound transducers with resonance frequencies of 0.5 and 1.5 MHz. Parameters such as frequency, duty cycle, and pulse repetition frequency (PRF) could be adjusted by controlling the signal generator. An external power of 16 V was used to drive the customized device, and the voltage was boosted to around 50 V to supply the power amplifier unit. For the stimulation pattern, 20% of the duty cycle (pulse duration of 200 µs) and PRF of 1 kHz were fixed for both devices, as shown in Figure 1 c. The LIPUS signal amplitude was fixed for the EXOGEN device, while it was adjustable for the customized LIPUS device.
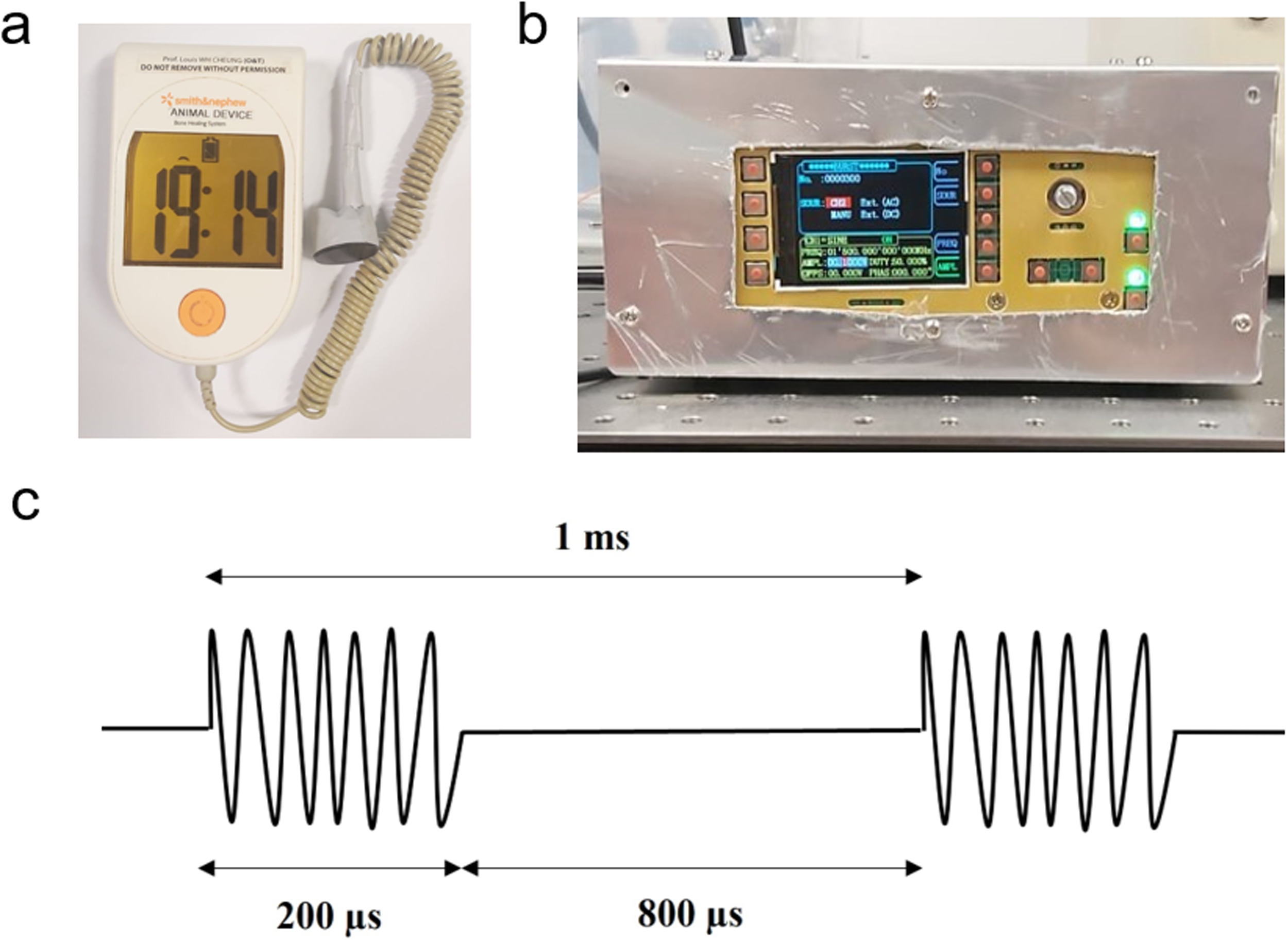
Acoustic intensity measurement in human cadaver and porcine tissues
The aim of this measurement was to investigate the relationship between acoustic intensity and tissue thickness, as well as the intensity patterns at different distances from the transducer surface. In this study, acoustic intensity, i.e., I SATA was measured in human cadaver and porcine tissues of different thicknesses (ranging from 1 cm to 5 cm). This range was chosen because the average trochanteric soft tissue thickness in hip fracture cases is 2.9 ± 1.1 cm in men [ ] and 4.0 ± 1.6 cm in women [ ]. The ethical approval was granted to use the human cadaveric tissue and was not required by the Joint CUHK-NTEC CREC (The Chinese University of Hong Kong-New Territories East Cluster Clinical Research Ethics Committee) for this study. A schematic diagram of the experimental setup is shown in Figure 2 . A water bath (length, 60 cm; width, 35 cm; height, 30 cm) was filled with degassed water to a height of 25 cm. A 25 mm diameter unfocused ultrasound transducer with an operating frequency of 0.5 MHz and 1.5 MHz was used. The diameter of the commercial LIPUS device was also 25 mm. The tissue sample was placed on top of the transducer, and a rugged needle-type hydrophone (HNR-1000, Onda Corporation, USA) was mounted immediately above the tissue. The distance between the transducer surface and the hydrophone was approximately 5.3 cm and was kept constant for all the measurements. During the measurements, the transducer was connected to the output of the laboratory-constructed and commercial LIPUS devices. The output of the hydrophone was connected to the data acquisition card (NI 5112, National Instruments, USA). The movement of the hydrophone was controlled by an XYZ motor controller to enable scanning of the whole tissue; this was operated using a custom-made program designed in LabVIEW (LabVIEW 2015 SP1, National Instruments, USA). The scanning range was set to 0–25 mm with a step size of 0.5 mm that generated a color map of a 50*50 matrix. The voltage recorded by the hydrophone was converted into acoustic intensity using the standard calibration factor V 2 cm 2 /W provided by the manufacturer of the hydrophone. The I SATA values were then calculated by drawing a circle with a diameter of 22 mm (to avoid data points in the low-intensity region at the edge of the transducer based on acoustic distribution pattern) using the custom script code in MATLAB r2016b (Math Works Inc., Natick, MA, USA). The color map generated by MATLAB shows the distribution pattern of the acoustic field, and the circle denotes the effective region of the transducer during ultrasound stimulation.
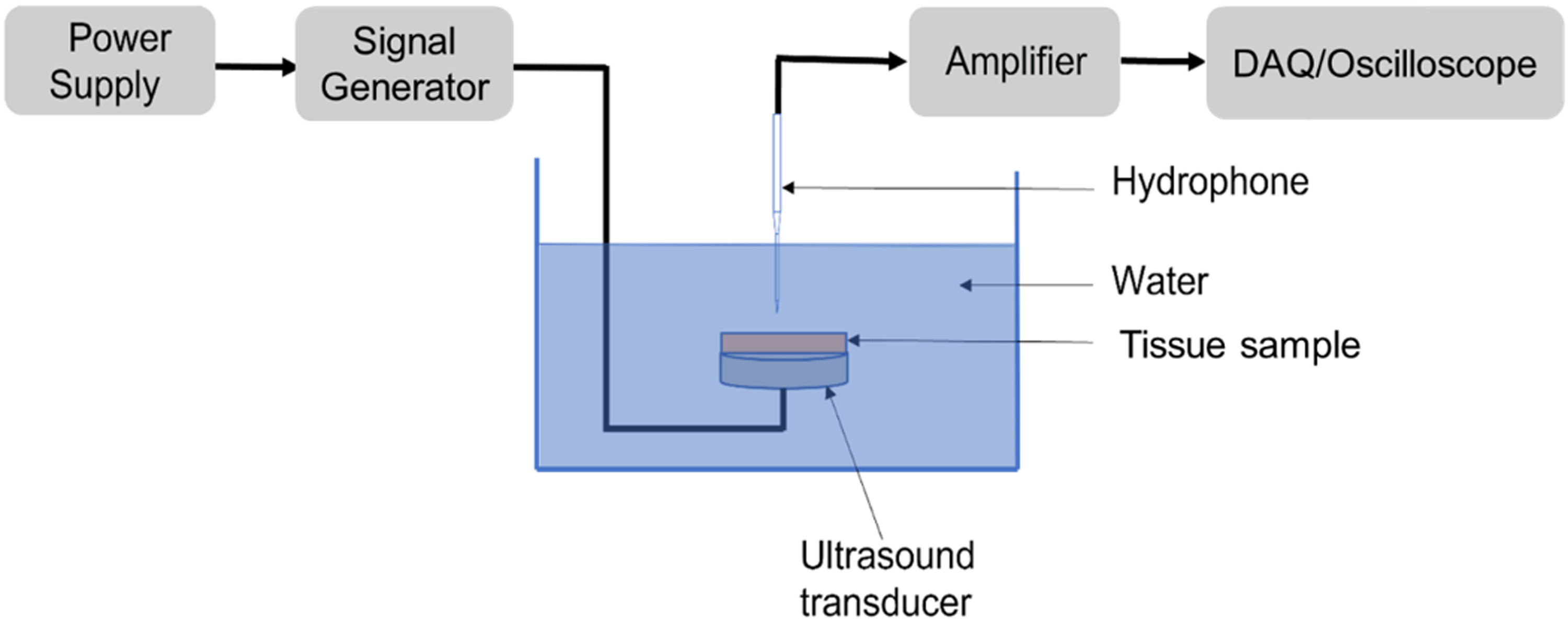
The size of the animal used in this animal study was smaller than that of human subjects, and the average soft tissue thickness covering the fracture location in the rabbit model was around 1 to 2 cm. Therefore, we also conducted additional scanning to map the acoustic intensity at distances of 1, 1.25, 1.5, 1.75, and 2 cm from the transducer surface for both 0.5 and 1.5 MHz transducers, using the scanning parameters as described above.
Animal model
Twenty adult New Zealand white rabbits (1.6–2 kg) were used in this study. All the surgical and experimental procedures were approved by the Animal Experimentation Ethics Committee (AEEC, Reference 20-051-MIS) of The Chinese University of Hong Kong. The rabbits were randomly allocated to four groups, namely: the control group, the positive control (PC) group (1.5 MHz with intensity fixed at 30 mW/cm 2 ), the 0.5 MHz group, and the 1.5 MHz group.
Surgical procedures for the fracture model
All the animals received general anesthesia using a mixture of ketamine (50 mg/kg) and xylazine (10 mg/kg) via intramuscular injection. The surgical site was shaved and aseptically prepared for the procedure. An incision was made between the biceps femoris and semimembranosus muscles with a scalpel blade (surgical blade, size 23) to expose the subtrochanteric region. Then an air-powered sagittal oscillating saw (Air pen drive, DePuy Synthes, Germany) with a blade thickness of 1 mm was used to create an open osteotomy at the subtrochanteric region, as shown in Figure 3 . Five holes were drilled with a diameter of 1.5 mm to fix the 3D-printed titanium implant (U3DP, The Hong Kong Polytechnic University, Hong Kong) using five self-tapping cortical screws (Biortho Co Ltd, Jiangsu, China). The surgical site was then cleaned using sterile saline, and the incision was sutured layer by layer (Mersilk, W580, Ethicon, USA). The animals were given an intramuscular injection of 0.1 ml of analgesic at the fracture site twice daily for three consecutive days after the surgery. A postoperative radiograph was taken immediately after the surgery to confirm the success of the fracture and followed up by weekly monitoring of the healing process (Xpert-80, Kubtec, Stratford, USA).
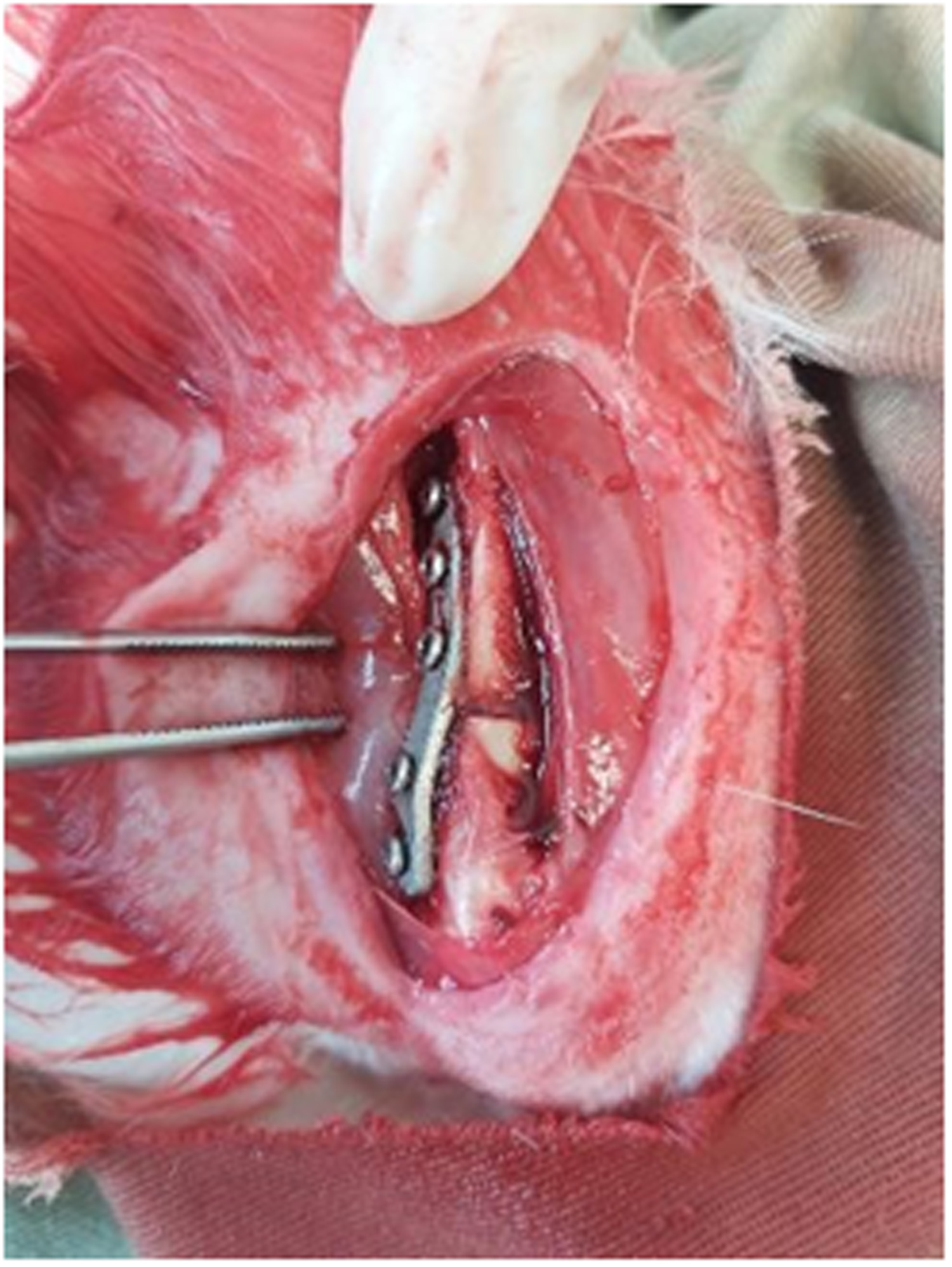
LIPUS treatment strategy
LIPUS treatments were given to the animals on the day following surgery. These rabbits received general anaesthesia during treatment and were placed in a supine position with the ultrasound treatment probe positioned directly above the fracture site ( Figure 4 ). The position of the fracture site was marked on the rabbit’s skin surface for accurate placement of the LIPUS probe to ensure exact delivery of the ultrasound signal over the surgical site. Treatment was given for 20 mins/day for a total of six weeks. Based on the measurement obtained during the acoustic intensity assessments after passing through different depths of porcine tissue, the input voltage was adjusted to deliver a consistent acoustic intensity of 30 mW/cm 2 for the 0.5 MHz and 1.5 MHz groups at the fracture surface. A portable ultrasonography device was used to measure the thickness of the soft tissue. During the measurement, the portable ultrasonography device was placed in the same area as that used for the LIPUS treatment, as shown in Figure 5 a. The thickness of the soft tissue was determined according to the ultrasound image, as shown in Figure 5 b. The thickness was measured weekly, and the output of the customized LIPUS device was adjusted to ensure a sufficient and consistent intensity for treatment.
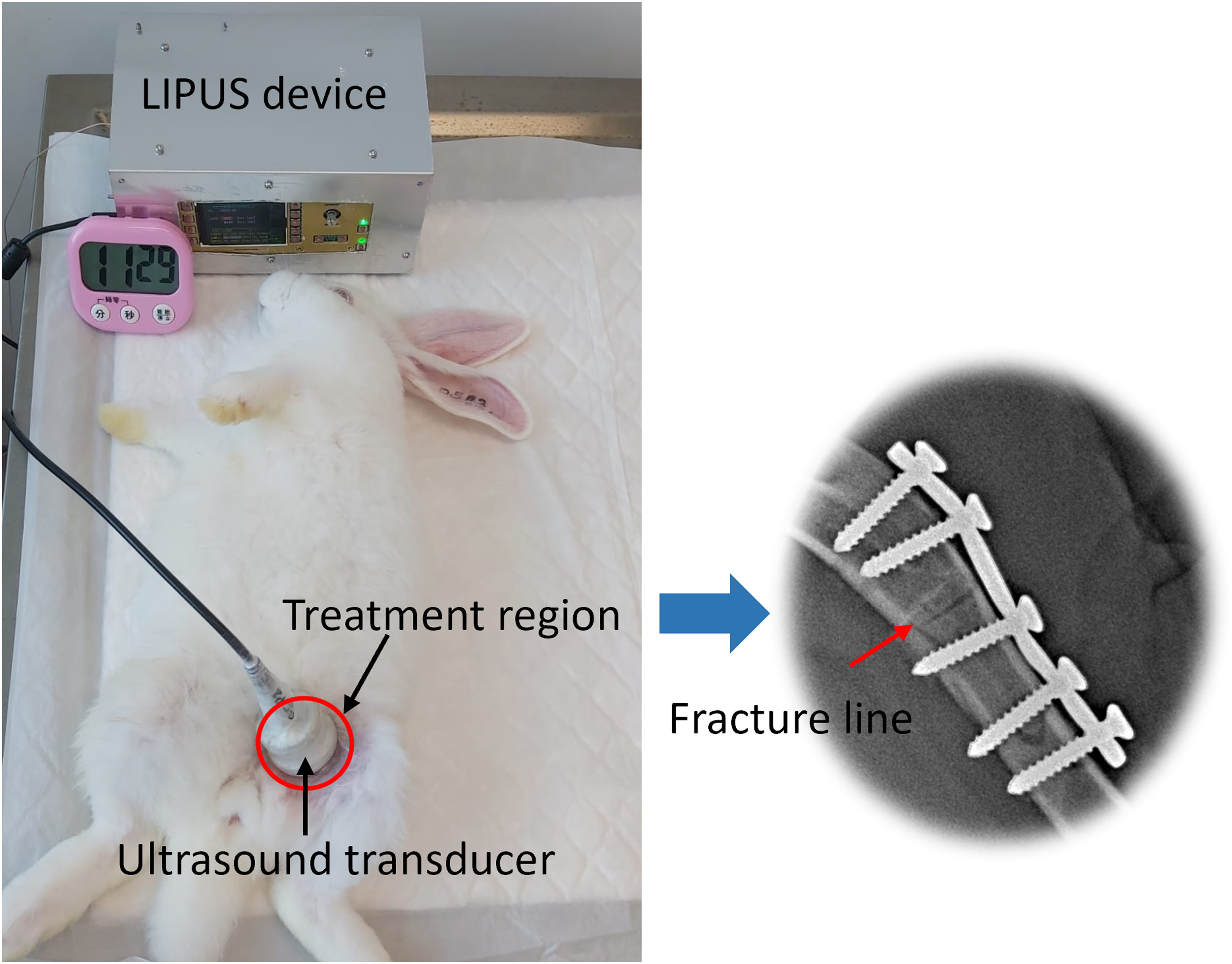
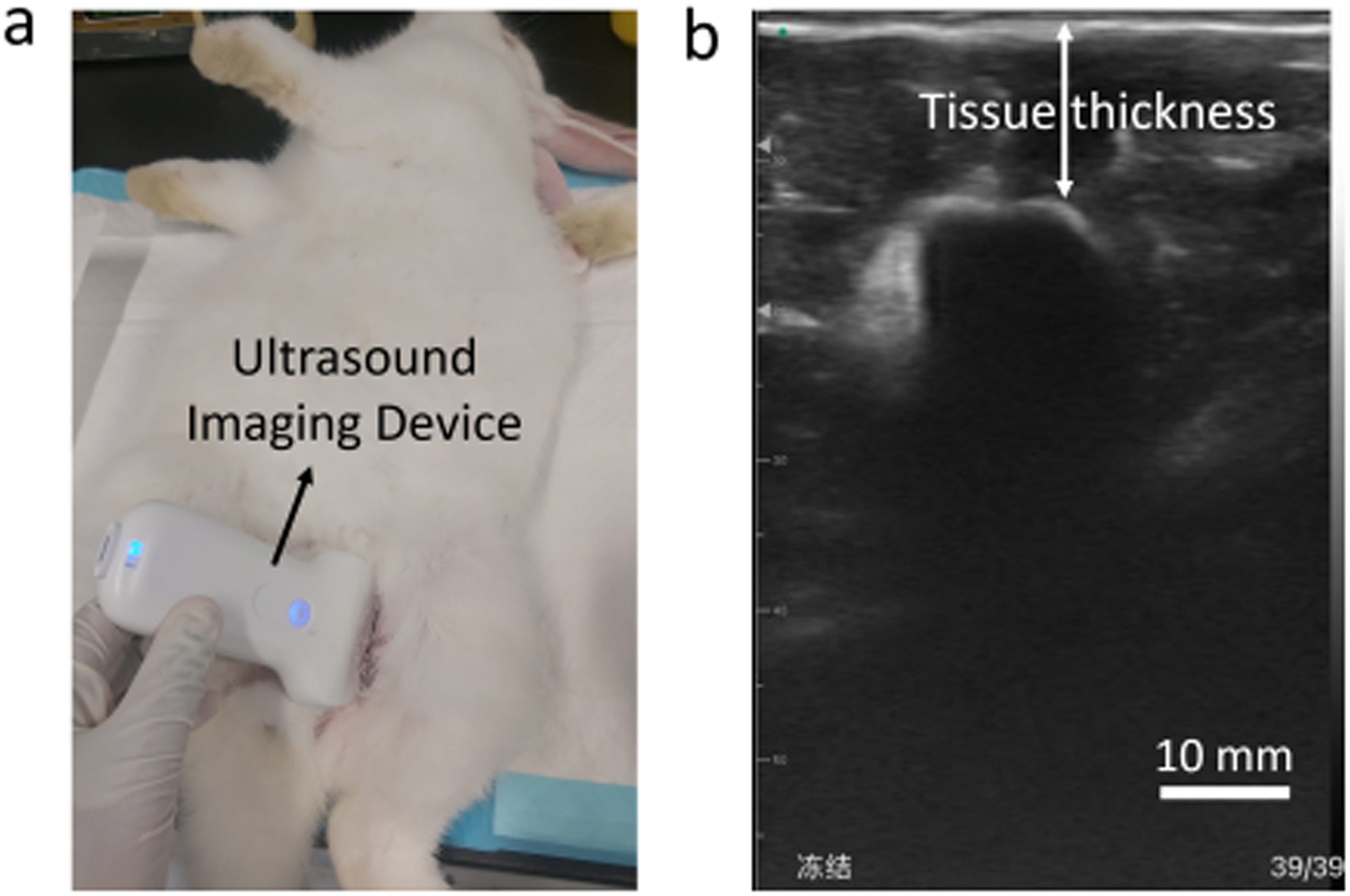
Radiographic examination
A plain radiograph was taken immediately after surgery and every week thereafter using a KUBTEC X-ray machine (KUBTEC XPERT-80, Stratford, USA) to monitor the stability of the bone fixation and to observe the fracture healing. The radiographs were taken under the same conditions using a standardized protocol. The initial osteotomy site was noted for identification of the newly formed bone.
Micro-CT analysis
After six weeks of treatment, all the animals were euthanized, and the femoral bones were harvested. The soft tissues surrounding the harvested femora and the metal implants were first removed after thawing in 0.9% saline at room temperature for 1 hour. Micro-CT scanning was performed using a multi-slice high-resolution peripheral quantitative computed tomography (HR-pQCT, XtremeCT, Scanco Medical, Bruttisellen, Switzerland) operating at 60 kV, according to the protocol for animal studies [ ]. The bones were fixed into a carbon-fiber sample holder. The subtrochanteric fracture site was scanned, resulting in 1316 slices, followed by the selection of the region of interest (ROI) and segmentation using Scanco analytical software v5.08b (Scanco Medical AG). Following the three-dimensional evaluation, the volumetric bone mineral density (BMD), bone volume (BV, mm 3 ), tissue volume (TV, mm 3 ), bone volume fraction (BV/TV, %), trabecular number (Tb.N), trabecular thickness (Tb.Th, mm), and trabecular spacing (Tb.Sp, mm) were generated for comparison. These microarchitectural parameters, such as Tb.N, Tb.Th, and Tb.Sp, help to provide information about the mechanical properties of the bone.
Mechanical testing
A standard four-point bending test was used to evaluate the mechanical properties of the fractured femora [ ]. Samples were stored in saline at -80°C and were thawed at room temperature for one hour. In the four-point bending test, the femur was placed perpendicular to the blades, after which the fractured region was located in the middle of the blades ( Figure 6 ), with lowering at a constant displacement rate of 5 mm/min (H25KS, Hounsfield Test Equipment Ltd, Redhill, Surrey, UK). Using the load–displacement curve, the ultimate load (N), stiffness (N/mm), and energy to failure (J) were evaluated using the built-in software.
