Alzheimer’s disease (AD) involves a complex pathophysiology of neurodegeneration that leads to severe cognitive deficiencies. Understanding the molecular alterations that underlie this disease is fundamental to clinical management and therapeutic innovation. Functional imaging with positron emission tomography (PET) enables a visualization of these impaired pathways, such as cerebral hypometabolism, amyloid and tau accumulation, and neurotransmitter dysfunction. This review discusses the clinical applications of PET in AD and mild cognitive impairment, focusing on relevant original research studies for disease diagnosis, progression, and treatment response.
Key points
- •
FDG PET, a marker of cerebral metabolic activity, reveals a specific pattern of temporoparietal hypometabolism in patients with Alzheimer’s disease (AD) that may extend to the frontal cortices with advanced disease.
- •
While amyloid PET may have high sensitivity in distinguishing AD from cognitively unimpaired elderly subjects, its specificity is low due to the presence of amyloid accumulation in natural aging and other neurodegenerative diseases.
- •
PET imaging of neurotransmitters is a promising field of study that has demonstrated impairment in the cholinergic, dopaminergic, and serotoninergic systems in AD.
- •
In combination with neurophysiological and cognitive testing, PET is instrumental to the assessment of therapeutic response for patients with AD; anti-amyloid therapies have yet to demonstrate clinically relevant correlates between PET findings and cognitive improvement.
Introduction
Alzheimer’s disease (AD) is a neurodegenerative disorder and the most common cause of dementia, affecting 6.9 million Americans aged 65 and older. AD characteristically arises in the elderly (ie, late-onset AD or LOAD) but occurs before the age of 65 in about 5% of patients (ie, early-onset AD or EOAD). It is the seventh leading cause of mortality in the United States and poses a significant financial burden on the health care system, with health care expenses amounting to $305 billion in 2020 and projected to increase substantially owing to a growing geriatric population. ,
The initial diagnostic criteria for AD were established by the 1984 Working Group of the National Institute of Neurologic and Communicative Disorders and Stroke and the Alzheimer’s Disease and Related Disorders Association (NINCDS-ADRDA). These clinical criteria outline the characteristically progressive cognitive decline observed in middle-aged or elderly patients without any clear, alternative underlying cause. Other factors which may contribute to cognitive impairment, such as thyroid disease, vitamin deficiencies (ie, B12, folate), renal or liver dysfunction, medication side effects, depression, or infectious and inflammatory conditions, are ruled out prior to attributing clinical symptoms to AD. While accurately diagnosing severe cases of dementia or reaching a diagnosis after observing patients clinically for 6 to 12 months are feasible, differentiating various etiologies of dementia in the early stages remains challenging. , Recognizing these diagnostic challenges, the National Institute on Aging and the Alzheimer’s Association (NIA-AA) revised the AD definition in 2011. Current clinical guidelines depict AD as a continuum of pathologies, ranging from mild cognitive decline to severe manifestations such as full-onset dementia.
The clinical evaluation of AD continues to evolve as the advancements in neuroimaging and genetics have identified biomarkers that may facilitate disease diagnosis and prognostication. , Among neuroimaging techniques, positron emission tomography (PET) has demonstrated the greatest potential for identifying the underlying pathophysiology of AD. Indeed, the revised NIA-AA guidelines acknowledge the unique role of biomarker evidence from PET in the delineation of AD and mild cognitive impairment (MCI), the latter indicating intermediate cognitive deficits that do not meet dementia criteria. , While this biomarker-based definition of AD serves primarily as a research framework, it underscores the significance of integrating biomarker data from PET neuroimaging into our understanding of MCI and AD.
The purpose of this review is to provide an overview of the clinical applications of PET imaging in AD, discussing major radiotracers used to investigate the molecular pathophysiology of this disease for both diagnostic and therapeutic purposes.
Metabolic imaging with FDG PET
The brain is a metabolically demanding organ, consuming nearly 20% of the body’s energy stores despite comprising just 2% of body weight. Cerebral metabolism is chiefly dependent on a continuous influx of glucose and oxygen to support the proper functioning of neurons. As such, severe reductions in the supply of these nutrients can lead to significant cognitive and motor dysfunction. The PET radiotracer [18F] fluorodeoxyglucose (FDG) acts as a glucose analog to map the patterns of metabolic activity in the brain. Most PET studies focusing on AD have utilized FDG to identify areas of significantly reduced cerebral glucose metabolism throughout the brain. Particularly notable is the hypometabolism observed in the bilateral temporoparietal regions, often described as the prototypical AD pattern ( Fig. 1 ). This pattern tends to be more pronounced in patients under 65 years of age or those with advanced symptoms.
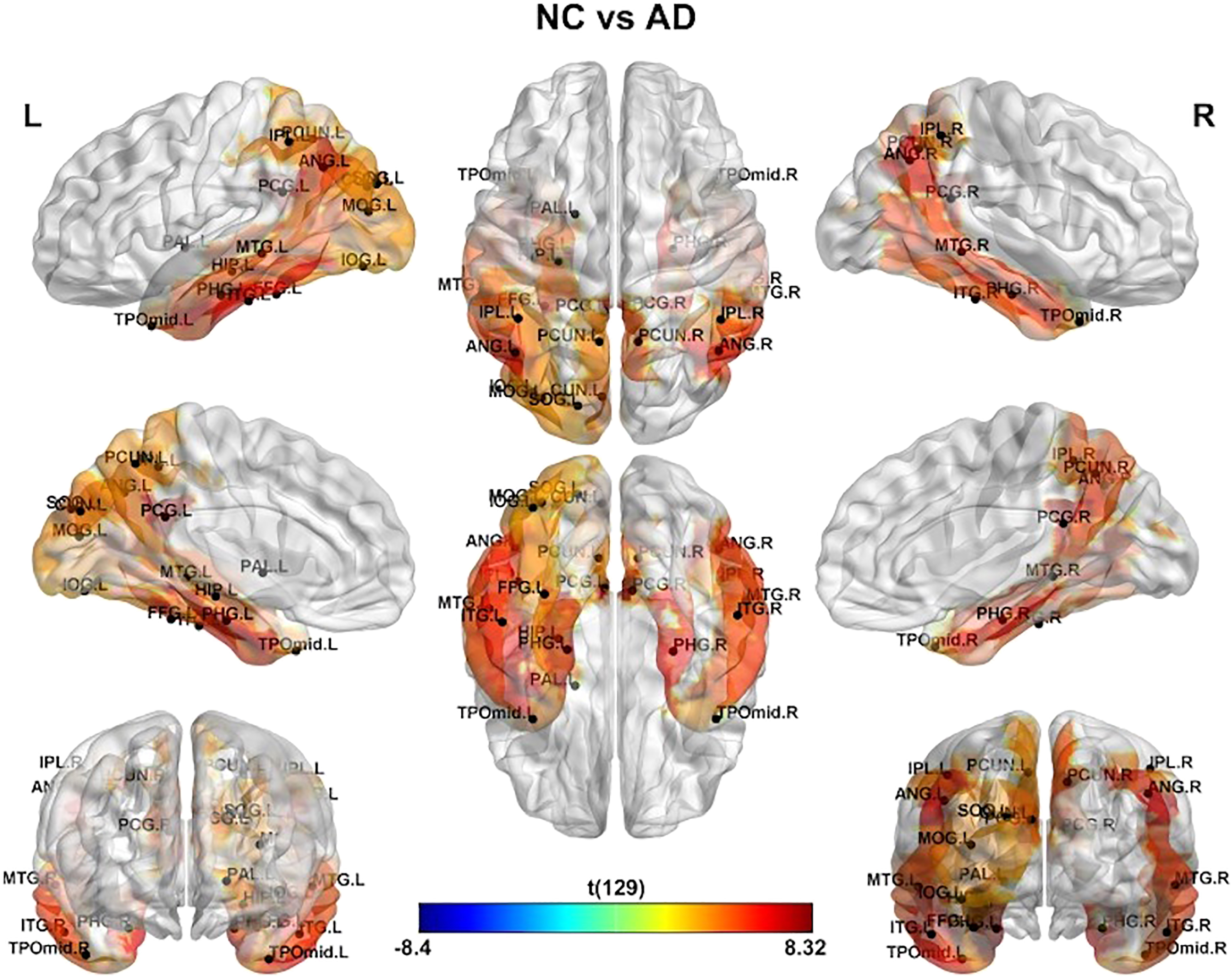
While FDG PET predominantly points to temporoparietal hypometabolism as a key indicator of AD status, reduced glucose utilization has also been observed in other brain regions, including the posterior cingulate and precuneus. Notably, there is a relative sparing of metabolic activity in the primary sensorimotor and visual cortices, basal ganglia, pons, thalamus, and cerebellum. As AD progresses, the frontal cortices also demonstrate decreased uptake of FDG. ,
The pattern of hypometabolism seen on FDG PET can aid in the differentiation of AD from normal aging as well as other forms of dementia. In a meta-analysis of 27 studies, FDG PET demonstrated an approximate sensitivity of 92% and specificity of 89% in distinguishing patients with AD from controls. The performance of FDG PET exceeded that of both computed tomography (CT) and magnetic resonance imaging (MRI), of which the latter is the most routinely used neuroimaging procedure in AD evaluation. A separate meta-analysis of 14 articles by Na and colleagues found that FDG PET could differentiate AD from frontotemporal dementia (FTD) with a sensitivity of 96% and specificity of 84%, as well as differentiate AD from dementia with Lewy bodies (DLB) with a sensitivity of 93% and specificity of 92%.
Longitudinal studies have consistently shown that the pattern of hypometabolism observed on FDG PET scans in AD is both persistent and progressive. For instance, Ossenkoppele and colleagues observed hypometabolism in the temporoparietal cortices and posterior cingulate in 8 patients with AD. Over a period of 2.5 years, further reductions in FDG uptake were identified in the frontal, parietal, and lateral temporal lobes. A separate study by Ishibashi and colleagues performed FDG PET on 2 patients with AD over 9 to 12 years, encompassing the preclinical to symptomatic stages of their disease. Whereas controls showed FDG reductions in AD-associated voxels at a rate of 2.2% per decade, the 2 patients exhibited decreases of over 9%.
FDG PET studies have also revealed unique patterns of metabolic activity in patients with EOAD. Iaccarino and colleagues assessed FDG PET in 134 patients with sporadic EOAD and 89 patients with dominantly inherited EOAD, observing greater global FDG reductions in sporadic EOAD. Moreover, temporoparietal hypometabolism was relatively greater in sporadic EOAD while hypometabolism in frontal white matter and pericentral regions was relatively greater in dominantly inherited EOAD. In a separate analysis of 27 patients with EOAD and 65 patients with LOAD, Mosconi and colleagues reported reduced FDG uptake in the orbitofrontal, parietal, and inferior temporal cortices in EOAD relative to LOAD. In contrast, LOAD patients exhibited lower FDG uptake in the anterior cingulate and medial temporal cortices.
Evidence suggests that the degree of hypometabolism correlates with the severity of dementia symptoms in AD patients. Reductions in hippocampal and posterior cingulate FDG uptake have been associated with dementia severity as assessed by the Global Deterioration Scale. Additionally, Khosravi and colleagues identified direct associations between regional FDG uptake, particularly in the temporal and parietal regions, and performance on the Mini-Mental Status Examination (MMSE).
Individuals with MCI are at an increased risk of developing AD, and FDG PET may assist in predicting this conversion. Mosconi and colleagues reported significant hypometabolism in the inferior parietal cortex of 8 patients with MCI who converted to AD relative to nonconverters within 1 year. An analysis by Ottoy and colleagues found that FDG PET was the single strongest determinant of the short-term conversion of MCI to AD, achieving an accuracy of 83% that surpassed the performance of amyloid, cerebrospinal fluid (CSF), and MRI biomarkers. Teng and colleagues developed a support vector machine combining longitudinal PET and cognitive features (eg, MMSE) to obtain an accuracy of 93% in predicting progression to AD.
A significant interpretive challenge with PET scans lies in their spatial resolution, making it difficult to distinguish metabolically active brain tissue from inactive ventricular and sulcal CSF spaces. Correction for brain atrophy is thus recommended for accurate PET-based glucose metabolism measurements. MRI scans are preferred over CT scans for volumetric corrections because of their superior accuracy in determining brain atrophy. Given the greater atrophy observed in AD patients compared with controls, volumetric correction becomes crucial to differentiate disease-related hypometabolism from tissue loss-related hypometabolism. However, debates persist regarding the necessity of atrophy correction for the cerebral metabolic rate of glucose (CMRGlu) values in AD patients. Early studies showed decreased whole-brain CMRGlu in AD patients compared with controls before atrophy correction, but no differences postcorrection. However, other studies have reported significantly lower global CMRGlu in AD patients both before and after atrophy correction, indicating genuine metabolic reductions in AD. , Beyond volumetric correction, employing more quantitative approaches to FDG PET data analysis could enhance the determination of AD course and severity. ,
Amyloid and tau PET
While PET continues to be utilized for the measurement of cerebral metabolic activity, there is an increasing emphasis on its use to identify new therapeutic targets and monitor treatment outcomes for AD. A major focus has been placed on the accumulation of amyloid-β (Aβ) proteins in the brain, now recognized as a key pathologic feature of AD. Consequently, PET research has honed in on imaging these Aβ plaques in the human brain, leading to the development of imaging agents that bind to Aβ plaques, such as [11C]- and [18F]-labeled tracers, for example, 6-OH-BTA-1 ([ N -methyl-]2-(4′-methylaminophenyl)-6-hydroxybenzothiazole), SB-13 (4- N -methylamino-4′-hydroxystilbene), and FDDNP (2-(1-{6-[(2-[F-18]fluoroethyl) (methyl)amino]2-naphthyl} ethylidene) malononitrile).
Aβ PET reveals positive amyloid accumulation in approximately 70% to 90% of patients with AD, , with plaques predominantly found in cortical gray matter regions. Studies have demonstrated increased [11C] PiB PET uptake in areas such as the frontal and temporoparietal cortices of patients with AD relative to healthy controls. , Elevated cortical [11C] PiB binding has been also shown in patients with MCI compared with control, with greater amyloid retention serving as a potential predictive marker of conversion from MCI to AD. ,
The short half-life of [11C] has limited its broader clinical use, prompting the development of second-generation [18F] labeled radiotracers such as florbetapir and florbetaben. , PET imaging studies using these second-generation tracers have shown similar patterns of Aβ aggregation as revealed by [11C] PiB.
While the use of Aβ PET in AD and MCI continues to expand, a number of substantial limitations must be considered. Aβ PET has high sensitivity in determining AD, yet 20% to 30% of cognitively unimpaired elderly subjects also exhibit significant Aβ retention. , Additionally, distinguishing between MCI and AD populations using Aβ PET can be challenging. One meta-analysis revealed that all 3 [18F]-labeled amyloid tracers had similar diagnostic accuracy, with high pooled sensitivity and specificity values. However, their performance was effective in distinguishing AD patients from healthy controls rather than differentiating between AD and MCI patients. Further limiting the utility of Aβ PET is the significant presence of off-target binding, as current radiotracers may inadvertently target regions of inflammation, white matter hyperintensities, and myelin damage.
A longitudinal study of [11C] PiB PET imaging indicated a 41.3% prevalence of positive amyloid burden among cognitively normal elderly individuals aged 80 to 89, compared with 2.7% between 50 and 59. These results demonstrate that Aβ plaques alone do not specify cognitive impairment among older populations, especially considering the limited association between brain amyloid load and dementia severity in AD patients. , Moreover, a comparison between FDG and [18F] florbetapir PET showed that global quantitative analysis of FDG uptake was found to more strongly correlate with MMSE in AD-associated brain regions, including both supratentorial and tentorial regions, than florbetapir. Notably, the pattern of amyloid deposition in florbetapir PET scans did not coincide with temporoparietal glucose hypometabolism shown in FDG PET scans ( Fig. 2 ). , It is the variety of findings and concerns regarding the diagnostic utility of amyloid tracers that raises questions about the value of amyloid PET in AD and other forms of dementia. ,
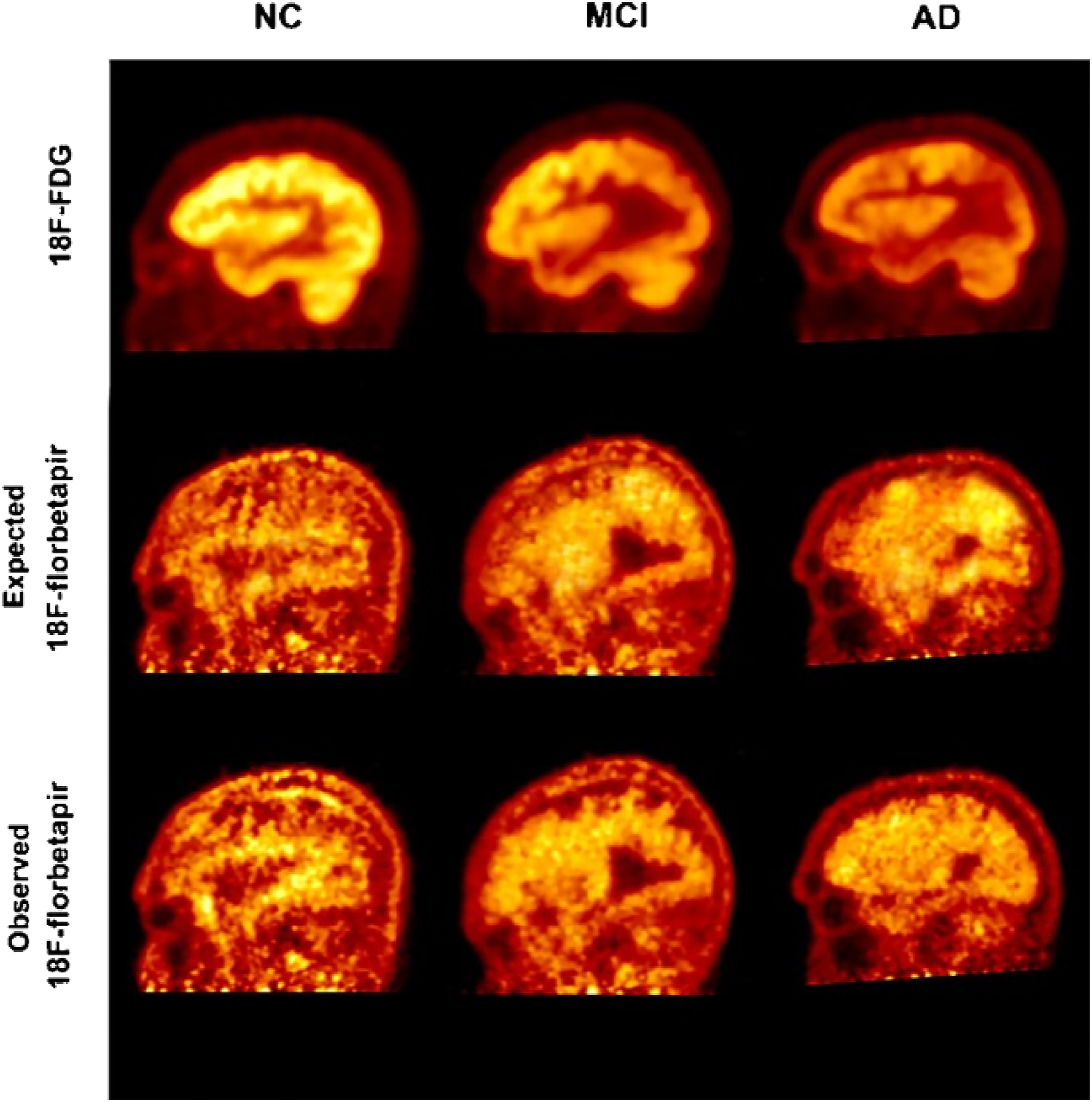
The accumulation of tau protein, forming neurofibrillary tangles, is closely associated with neurodegeneration and cognitive decline, similar to Aβ plaques. In vivo detection of tau protein deposits offers a valuable technique for diagnosing tauopathies and predicting disease progression. Recently developed tau PET tracers, including PBB3, T807, and THK-5117, have shown promise in imaging neurofibrillary pathology. Moreover, [18F] THK-523, [18F] THK-5105, and [18F] THK-5117 have demonstrated high tau selectivity over Aβ in the AD brain. Initial PET studies of [18F] T807 in humans revealed significant tracer retention in areas with paired helical filaments of tau, associated with increased disease severity in patients with MCI and AD. Retention of [18F] THK-5105 has been correlated with dementia severity and brain atrophy, with AD patients showing stronger correlations between cortical atrophy and tau deposition in specific brain regions than controls. This led to the hypothesis that interactions between amyloid and tau deposition might drive atrophy in certain brain regions throughout AD progression.
In a study comparing [18F] florbetaben and [18F] flortaucipir retention using PET imaging, Bullich and colleagues found a significant correlation between Aβ plaques and tau deposition. Specifically, they observed elevated tau deposition in individuals with existing amyloid pathology, particularly in the fusiform gyrus and lateral temporal cortex. Conversely, patients without significant Aβ pathology showed minimal to no tau deposition. While PET imaging tracers for amyloid and tau offer distinct insights, these studies emphasize the importance of recognizing their association in AD pathology.
The clinical utility of tau PET in characterizing AD remains limited, with notable concerns surrounding tracer specificity and the presence of off-target binding. In vivo studies have demonstrated significant off-target binding effects of various tau tracers in the basal ganglia, which are believed to result from an affinity to monoamine oxidase B (MAO-B). This has been further corroborated by a postmortem analysis of an AD patient that revealed binding of the tau PET tracer [18F] THK-5351 to MAO-B. Development of novel tracers that are highly specific to tau is critical to advancing and legitimizing the application of tau PET in AD.
Neurotransmitter PET
AD is strongly associated with neurotransmitter dysfunction, particularly of the cholinergic system. Cholinergic impairment and loss of neurons are seen in the basal forebrain, whereas striatal interneurons and innervation of the thalamus are relatively preserved. This has motivated the clinical approval of several cholinesterase inhibitors for the treatment of AD. By visualizing the function of neurons and their neurotransmitters at a molecular level, PET may offer novel insights into AD-associated neuropathy and its impact on cognitive and functional outcomes.
Several developed PET radiotracers targeting the cholinergic system, such as N-[11C] methyl-4-piperidinyl propionate ([11C] PMP) and N-[11C] methyl-4-piperidyl acetate ([11C] MP4A), are specific to the enzyme acetylcholinesterase (AChE) that functions to increase acetylcholine turnover. , Imaging with these radiotracers has revealed reductions in AChE activity in regions such as the neocortex, hippocampus, and amygdala in AD patients, with the temporal and parietal cortices being the most affected. This aligns with the pattern of temporo-parietal hypometabolism observed in FDG scans. A separate study using MP4A PET demonstrated that the patients with AD at various disease stages present with reduced AChE binding in the temporal cortex. While EOAD patients displayed global reductions in AChE, those with LOAD showed reductions mainly in specific cortical regions. Notably, EOAD patients exhibited more pronounced AChE reductions than LOAD patients, suggesting that AChE may be of therapeutic interest particularly for EOAD.
In a study of the PET ligand (+)-[18F] flubatine, which assesses nicotinic acetylcholine receptor (nAChR) activity, AD patients showed reduced binding of (+)-[18F] flubatine in several brain regions including the left hippocampus, precuneus, putamen, right anterior orbital frontal cortex, right paracentral lobule, left anterior cingulate cortex, and left triangular inferior frontal gyrus ( Fig. 3 ). The most pronounced reductions were observed in the bilateral mesial temporal cortices. Additionally, there was a negative correlation between (+)-[18F] flubatine binding in the lateral temporal cortex and Aβ deposition in cortical regions, possibly due to amyloid-induced damage to associated neuronal fibers. However, the authors noted that this explanation was specific to the lateral temporal region and did not apply to other observed cortical regions.
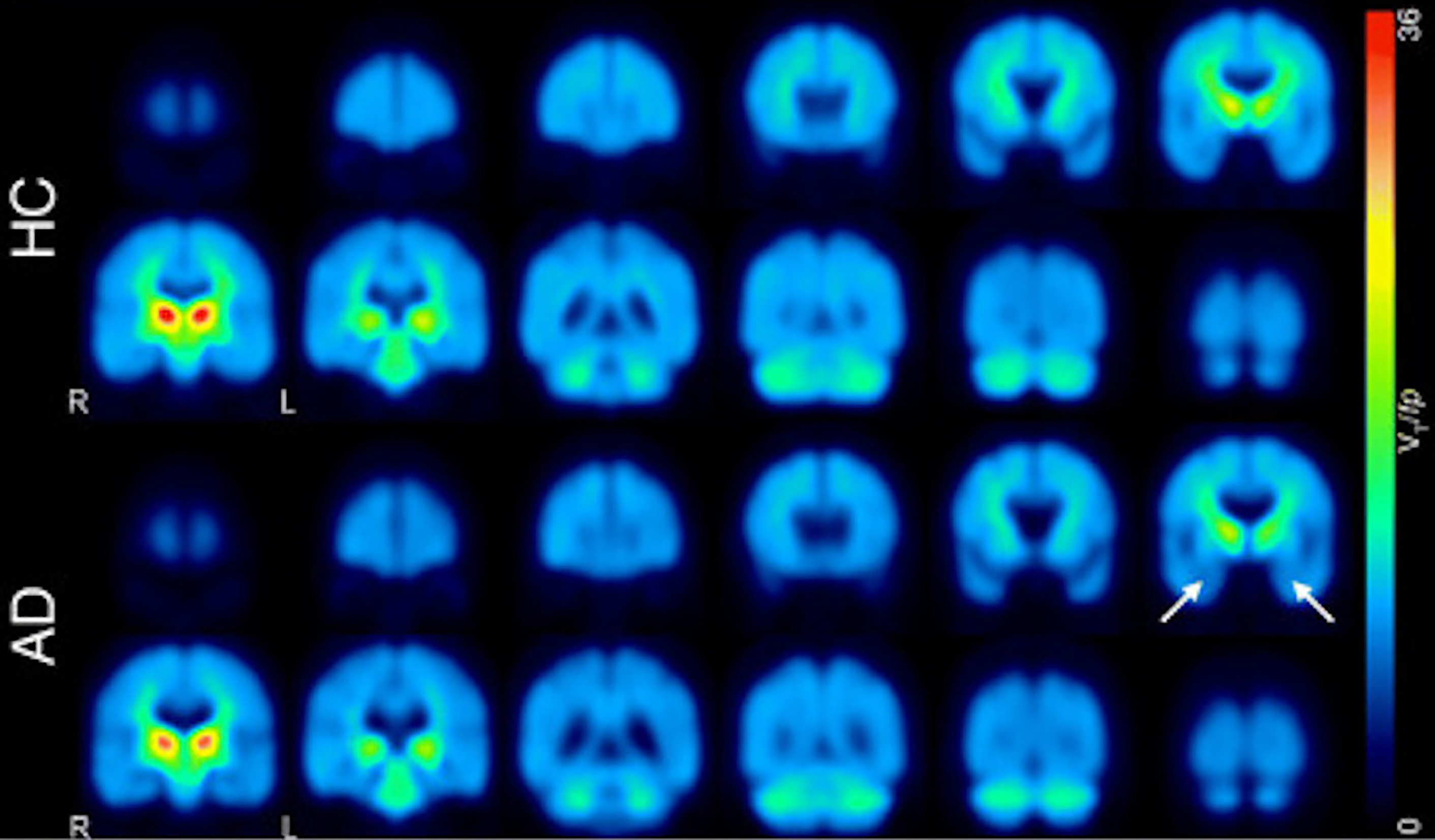
A separate study involving 9 AD patients, 8 MCI patients, and 7 age-matched healthy controls examined the binding of the PET radiopharmaceutical 2-[18F] FA-85380 BP(ND), which also measures nAChR activity. The study found reduced binding of 2-[18F] FA-85380 BP(ND) correlating with the severity of cognitive impairment. Importantly, only MCI patients who later progressed to AD showed reduced binding, suggesting that PET imaging of nAChR may not only reflect cognitive impairment severity but also predict disease progression.
Beyond the cholinergic system, PET has been applied to explore AD-associated changes in the serotonergic system, which governs fundamental behaviors including mood, sleep, and cognition. PET studies have demonstrated reductions in the cerebral serotonin transporter (5-HTT), 5-HT 1A receptor, and 5-HT 2A receptor among patients with AD. For instance, a study of [18F] setoperone (5-HT 2A receptor antagonist) showed decreased uptake in various cortical regions, including the temporoparietal and frontal areas, of 9 AD patients compared with controls. A separate analysis by Ouchi and colleagues revealed striatal 5-HTT reductions in 8 nondepressed AD patients relative to controls, indicating presynaptic cholinergic alterations before the onset of emotional or psychiatric manifestations.
During a 2-year follow-up of MCI patients, 8 out of 14 initially diagnosed subjects met probable AD criteria, but no significant changes in 5-HT 2A receptor binding were observed compared with baseline values. The authors of this study, therefore, concluded that reduced 5-HT 2A receptor binding in MCI patients occurs slowly and is not associated with progression to AD. These findings suggest that decreased cortical 5-HT 2A receptor binding may be an early feature in MCI, but additional reductions are not linked to MCI-to-AD progression. Similar to the observations with 5-HT 2A receptors, a notable reduction in the binding potential of 5-HT 1A receptors has been observed in AD patients compared with healthy controls confined to the medial temporal lobe.
In contrast, a separate study on 5-HT 1A PET in AD and MCI patients revealed significant reductions in 5-HT 1A receptor densities in the bilateral hippocampi and raphe nuclei of AD patients. The authors further reported that decreased 5-HT 1A receptor binding in the hippocampus strongly correlated with deteriorating cognition, as assessed by MMSE scores, and reduced cerebral glucose metabolism, as measured by FDG PET. A voxel-based analysis of 5-HT 1A receptor density using PET also showed decreased whole-brain binding in AD patients, while patients with amnestic MCI (aMCI) exhibited increased binding. Further regional analysis revealed that AD patients had reduced 5-HT 1A receptor binding in the hippocampus and parahippocampus, whereas aMCI patients showed increased binding in the inferior occipital gyrus. These findings suggest that PET imaging of 5-HT 1A receptor binding could potentially differentiate aMCI patients from those with mild AD.
Given the need to differentiate AD from Parkinson’s dementia, DLB, and depression, a common finding in AD patients, PET imaging of the dopaminergic system has received increased attention. [11C] raclopride PET has been used to study dopamine D2 receptor availability, demonstrating reduced hippocampal binding in patients with AD and correlating with severity of memory impairment. However, other studies of [18F] AV-133 (florbenazine), a tracer of the dopaminergic vesicular monoamine transporter 2 (VMAT2), reported mixed or negative findings on dopaminergic degeneration in patients with AD. ,
A small study of 27 MCI patients explored the diagnostic utility of PET by comparing striatal dopamine terminal integrity measured by [11C] dihydrotetrabenazine and cerebral amyloid burden measured by [11C] PiB. The study classified 11 subjects as amnestic MCI, 7 as multidomain MCI, and 9 as nonamnestic MCI based on initial clinical diagnoses. At a mean follow-up of 3 years, cerebral amyloid deposition or nigrostriatal denervation strongly predicted MCI-to-dementia conversion. However, PET-based subtype classification only moderately agreed with clinical subtype classification.
PET in AD therapeutics
PET imaging holds significant potential for evaluating therapeutic interventions for AD. In recent years, there has been a surge in efforts to develop pharmaceutical and immunologically mediated therapies for AD. In the context of patient management, PET imaging can be employed before treatment to identify promising therapies and targets for individual patients. Additionally, longitudinal PET scans can assess a therapy’s effectiveness and mechanism of action over time. An example of the utility of PET in evaluating pharmacologic therapy is its role in understanding donepezil’s impact on AChE activity. Contrary to earlier beliefs that donepezil achieves nearly total inhibition of cerebral cortical AChE activity in patients with AD, Kuhl and colleagues showed an average AChE activity inhibition of only 27% following donepezil administration. Moreover, an amyloid PET study by Pyun and colleagues found no significant association between AChE inhibitor activity and amyloid burden or cognitive decline in AD patients. These insights highlight PET’s role in elucidating AD mechanisms, guiding clinical trial development, and ensuring appropriate pharmaceutical use.
In the domain of AD immunotherapy, PET imaging has been used to assess the efficacy of vaccines targeting immunologic responses against amyloid protein. For instance, [18F] florbetapir PET was utilized to assess amyloid burden in a phase 2 randomized control trial of an experimental vaccine for AD, known as vanutide cridificar (ACC-001) combined with Quillaja saponaria (QS-21). The study revealed that neither of the vaccine-administered groups showed significant reductions in amyloid burden or clinical improvements compared with the controls. Intriguingly, participants who received either vaccine dose experienced a faster decline in brain volume. This research challenges the utility of detecting amyloid in the brain for developing effective therapies and questions the use of amyloid burden as a clinical progression indicator.
This issue gained further attention due to the controversy surrounding the FDA’s approval of aducanumab, an anti-amyloid monoclonal antibody, as a clinical treatment for AD. Amyloid PET imaging was instrumental in evaluating aducanumab’s impact on amyloid burden in AD patients during the EMERGE and ENGAGE trial experimental arms. However, no clear clinical benefit was observed from the divergent evidence presented in these trials.
Clinical trials of lecanemab and donanemab have claimed the clinical benefit of reducing cognitive decline by 27% and 36% over 18 months of treatment, respectively. , For lecanemab, the reported effect on the primary endpoint was a difference of 0.45 on the Clinical Dementia Rating-Sum of Boxes (CDR-SB) scale. For donanemab, the reported effect was a difference of 3.25 on the integrated Alzheimer’s Disease Rating Scale (iADRS), a difference of 0.67 in CDR-SB, and a difference of 0.48 to 0.57 points on the MMSE. These results, however, may not have true clinical significance for patients, as a separate study estimated a change of 1 to 2 points in the CDR-SB as a minimal clinically important difference. Moreover, greater differences in the CDR-SB and MMSE have been achieved with conventional AD therapy.
A recent study used [18F] florbetaben PET to assess changes in Aβ plaque deposition of 3 patients with mild AD participating in focused ultrasound blood–brain barrier opening, a novel technique to optimize delivery of adacanumab over a 6-month period. While the protocol achieved significant reductions in Aβ deposition, there were no significant changes in cognitive or behavioral outcomes. Nonetheless, this study presents a promising proof-of-concept of drug delivery as assessed by PET imaging and its potential implications for AD.
The controversial performance of anti-amyloid therapeutics underscores the need to contextualize PET imaging, especially when many amyloid radiotracers remain prone to nonspecific binding. Further research is required to determine the optimal imaging techniques for assessing AD progression and identifying the most effective therapeutic targets for AD treatment.
Summary
PET imaging has been instrumental in investigating and evaluating the complete disease process of AD. Recent innovations in tracer development, artificial intelligence techniques, and -omics models have further elevated the value of PET in AD. Expanding on this role, PET techniques are likely to remain crucial in uncovering AD’s pathophysiology, managing the disease clinically, and designing and assessing therapeutic strategies targeting AD pathology.
Clinics care points
- •
FDG PET reveals a characteristic pattern of temporoparietal hypometabolism in AD that can distinguish this disease from other dementias and normal aging.
- •
The utility of amyloid PET for patients with AD remains contentious due to limitations of existing radiotracers and an uncertain clinical benefit of anti-amyloid therapeutics.
- •
Emerging PET radiotracers of neurotransmitter pathways are an active area of scientific research that may elucidate the clinical relevance of these systems in AD pathophysiology.
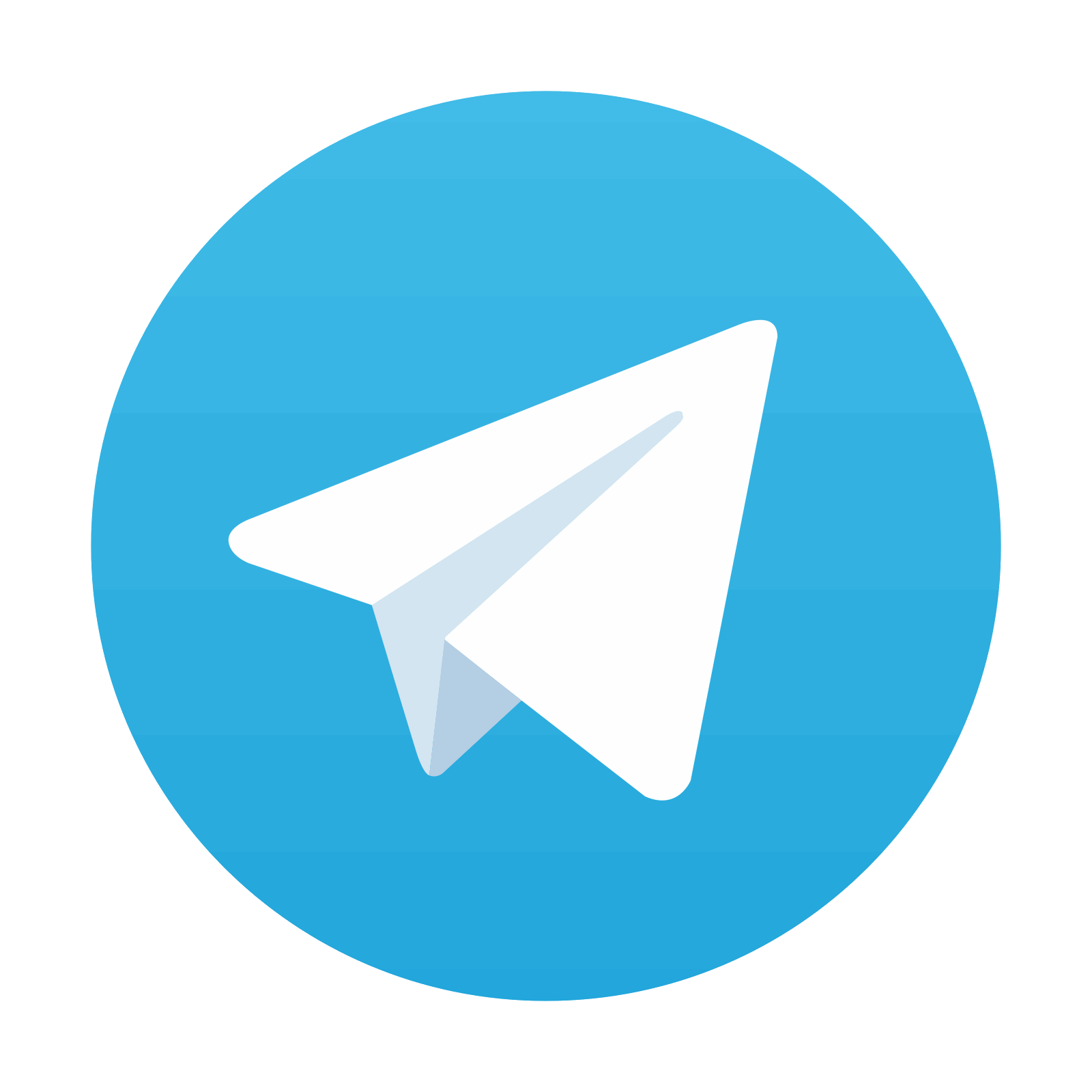
Stay updated, free articles. Join our Telegram channel

Full access? Get Clinical Tree
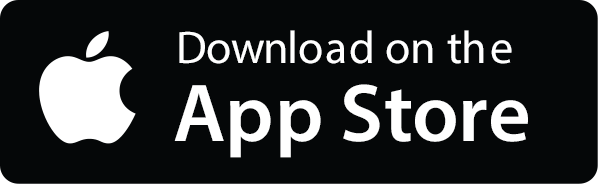
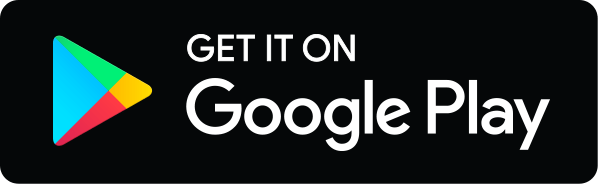
