This review delves into the principles of PET imaging and radiomics, emphasizing their importance in detecting, staging, and monitoring various cancers. It highlights the clinical applications of PET radiomics in oncology, showcasing its impact on personalized cancer care. Additionally, the review addresses challenges such as standardizing PET radiomics, integrating multiomics data, and ethical concerns in clinical decision-making. Future directions are also discussed, including broader applications of PET radiomics in clinical trials, artificial intelligence integration for automated analysis, and incorporating multiomics data for a comprehensive understanding of tumor biology
Key points
- •
PET imaging provides functional information on tumor metabolism and offers profound insights into tumor biology.
- •
The integration of PET imaging and radiomics enhances cancer diagnosis, prognosis, radiation treatment planning, and outcome prediction.
- •
Challenges include standardizing PET radiomics, integrating multiomics data, and addressing ethical concerns in using PET radiomics to aid clinical decision-making.
- •
PET radiomics can be applied in clinical trials, integrated with artificial intelligence for automated analysis, and combined with multiomics data to provide a comprehensive view of tumor biology.
Introduction
Cancer remains a significant global health burden, with millions of new cases and deaths annually. In the United States, over 2 million new noncutaneous cancer cases and 600,000 cancer deaths are projected to occur in 2024. Optimizing personalized treatment and monitoring of each patient’s disease and risk status are essential to achieve best outcomes for cancer treatment. The complexity of cancer biology, coupled with tumor heterogeneity, presents challenges for diagnosis, treatment, and monitoring. Advanced imaging technologies promise to help address these challenges, aiming to improve the precision and effectiveness of cancer management. Recent advances in medical imaging and quantitative imaging data analytics, particularly radiomics, empower major breakthroughs in managing cancer and bring new hope of “cure” for more cancer patients.
Among the imaging modalities, PET has emerged as a critical tool in oncology, offering functional insights by visualizing metabolic and molecular processes within the body and complementing traditional imaging techniques such as computed tomography (CT) and MR imaging that primarily provide anatomic information. This capability allows PET to detect early-stage tumors, assess tumor aggressiveness, and monitor treatment response with greater accuracy.
Radiomics is a relatively new imaging data analytical technique that enhances the value of medical imaging in oncology research and patient care. By integrating quantitative image features derived from medical images with statistical data analysis and machine learning (ML) techniques, radiomics is capable of uncovering patterns of imaging data for improving cancer diagnosis, staging, and prognosis, offering a noninvasive means of tumor characterization. Recent advances in radiomics have enabled the prediction of clinical outcomes based on radiologic imaging data. ,
The synergy between PET imaging and radiomics enhances their complementary strengths, presenting unique opportunities to advance cancer research and patient care. , , Specifically, PET provides detailed information on tumor metabolism, whereas radiomics converts this information into a set of high-dimensional features that can be analyzed and integrated in radiomic models for aiding cancer diagnosis and prognosis and predicting clinical outcomes. This integration has the potential to improve diagnostic accuracy and enable more personalized treatment. Emerging trends in the field include the integration of multiomics data, the use of artificial intelligence (AI) and ML to analyze high-dimensional features, and the development of standardized protocols to ensure reproducibility. These advancements have the potential to transform cancer care, making it more precise, personalized, and effective.
To provide a review of the roles of PET imaging and radiomics in radiation oncology, we explore their individual contributions and the advantages of their integration, highlighting current applications, challenges, and future directions. This will enhance the understanding of the potential and impact that PET radiomics has on personalized oncology and the broader field of cancer research.
PET imaging in radiation oncology
PET Imaging and Its Role in Cancer Detection and Staging
PET is a nuclear imaging technique widely utilized in radiation oncology for visualizing metabolic processes in the body by detecting the distribution of radiotracers. , Radiotracers are molecules tagged with positron-emitting isotopes to show the distribution of the radiotracer within the body. The strength of PET lies in its ability to provide functional information. 18 F-fluorodeoxyglucose (FDG), a glucose analog, is the most commonly used radiotracer in cancer imaging to visualize high glucose metabolism of tumors. , This allows PET to detect tumors that may not be visible on anatomic imaging, assess tumor activity, and evaluate treatment response. PET imaging is typically combined with CT or MR imaging to provide both functional and anatomic information in a single scan. PET/CT has become a standard tool, and PET/MR imaging has also seen wide applications in oncology, offering comprehensive insights into tumor biology and improving diagnostic accuracy.
PET imaging plays a crucial role in the detection and staging of cancer. Early detection is vital for improving outcomes, and PET’s ability to identify metabolic changes at the cellular level makes it particularly valuable in this context. For example, in lung cancer, FDG-PET is used to detect primary tumors and assess the extent of disease, helping to determine whether surgery is a viable option. , PET is also used to detect metastatic disease, guiding treatment decisions and improving prognosis. In lymphoma, PET imaging is a standard tool for staging and assessing treatment response. It provides information on the metabolic activity of the tumor, which can be more predictive of treatment response than anatomic tumor size alone.
Clinical Applications of PET in Radiation Oncology
PET imaging has become integral to the management of several cancer types, including lung, prostate, lymphoma, head and neck, and breast cancers. Its ability to provide functional and metabolic information that complements anatomic imaging is crucial for guiding treatment planning, including decisions on localized or systemic therapy and the use of additional treatment or consolidation strategies.
- •
Lung Cancer: PET-CT is the standard imaging modality for staging non-small cell lung cancer (NSCLC). , It helps identify the extent of disease, particularly the presence of metastatic lesions that may not be visible on CT or MR imaging.
- •
Prostate Cancer: PSMA-targeted PET imaging has transformed prostate cancer management. It allows for the detection of recurrent disease even at low prostate-specific antigen levels and provides precise localization of metastatic lesions.
- •
Lymphoma: PET imaging has been a standard tool in the management of lymphoma. It is used for initial staging, evaluating treatment response, particularly in assessing complete metabolic response, and detecting relapse.
- •
Head and Neck Cancer: In head and neck cancer, PET imaging is used for staging, radiation therapy planning, and assessing treatment response.
- •
Breast Cancer: While PET is not routinely used for early-stage breast cancer, it plays a crucial role in assessing metastatic disease and monitoring response to therapy as FDG-PET can help identify metastatic lesions in cases where conventional imaging is inconclusive, influencing treatment decisions.
The wide application of PET is facilitated by radiotracers that can target specific cancer types or biological processes. These radiotracers offer unique insights into tumor biology, allowing for more personalized cancer management. The choice of radiotracer depends on the type of cancer being investigated and the commonly used radiotracers include:
- •
18 F-FDG: FDG is the most commonly used radiotracer in cancer imaging, , allowing PET to detect tumors that may not be visible on anatomic imaging, assess tumor activity, and evaluate treatment response.
- •
18 F-fluorothymidine (FLT): FLT is a radiotracer used to measure cell proliferation. It targets thymidine kinase, an enzyme involved in DNA synthesis, making it useful for assessing tumor aggressiveness and monitoring response to therapy.
- •
68 Ga-prostate-specific membrane antigen (PSMA): PSMA is highly expressed in prostate cancer cells, making this radiotracer particularly effective for detecting primary and metastatic prostate cancer. PSMA-targeted PET imaging has revolutionized prostate cancer management by enabling earlier detection and more precise localization of disease.
- •
64 Cu-DOTA-TATE: This radiotracer targets somatostatin receptors, which are commonly expressed in neuroendocrine tumors.
- •
18 F-fluoromisonidazole (FMISO): FMISO is used to image hypoxia within tumors, facilitating tailored radiation therapy to target treatment-resistant areas more effectively.
Advantages and Limitations of PET Imaging
PET imaging has its unique advantages and limitations when applied to cancer research and patient care. , Its advantages include (1) providing insights into metabolic and molecular processes that are not available through anatomic imaging alone, making it highly effective in detecting cancer and assessing treatment response; (2) allowing for the measurement of metabolic activity, which can be used to assess tumor aggressiveness and predict outcomes; (3) enabling whole-body imaging to facilitate the detection of both primary tumors and metastatic lesions in a single scan; and (4) offering versatility with various radiotracers tailored to different types of cancer and biological processes. Additionally, specifically in radiation oncology, PET imaging better informs target delineation and treatment planning relative to conventional anatomic imaging. , However, the wide application of PET imaging is impeded by its high cost, relative limited accessibility, and radiation exposure. PET imaging is expensive and not as widely available as other imaging modalities such as CT and MR imaging. The effectiveness of PET imaging is dependent on the availability and accuracy of radiotracers. The high cost of the equipment and radiotracers can limit access to this technology, particularly in low-resource settings. PET imaging also involves exposure to ionizing radiation, which raises concerns about cumulative radiation doses, particularly in patients who require multiple scans. Moreover, despite providing improved functional information, the spatial resolution of PET imaging is lower than that of CT or MR imaging. Such low spatial resolution can make it challenging to precisely localize small lesions or differentiate between closely situated structures.
PET radiomics
Radiomics
Radiomics is a quantitative imaging data analysis method that gleans quantitative information from medical images as a large number of image features, often referred to as radiomic features, , and builds classification or predictive models on those imaging features for cancer diagnosis, prognosis, and prediction of treatment response. , , The radiomic features can also be correlated and integrated with genomic information, biological markers, clinical measures, and outcomes using statistical analysis and ML methods so that medical imaging data can be used to uncover imaging patterns that can improve the diagnosis, prognosis, and treatment of cancer. In cancer research, radiomics provides a noninvasive means for characterizing tumor heterogeneity, assessing treatment response, and predicting outcomes based on imaging data, complementing biopsies, and genomic tests. This makes radiomics a powerful tool in personalized oncology, facilitating personalized treatment tailored to the individual characteristics of each patient’s tumor.
The integration of PET imaging with radiomics brings together the strengths of both approaches, offering a powerful tool for cancer research and clinical practice. One of the key advantages of PET imaging is its ability to capture metabolic activity, which is often closely correlated to tumor aggressiveness. By incorporating PET data into radiomic analysis, radiomic features can be computed to reflect both the anatomic and functional characteristics of the tumor, providing a more detailed characterization of the tumor and potentially leading to more accurate cancer diagnosis, prognosis, and prediction of treatment response.
Radiomics Workflow: From Imaging Data to Radiomic Models
A typical radiomic study workflow consists of image acquisition, image preprocessing, tumor segmentation, radiomic feature extraction, and classification or predictive modeling, as well as model validation and applications, as illustrated in Fig. 1 .
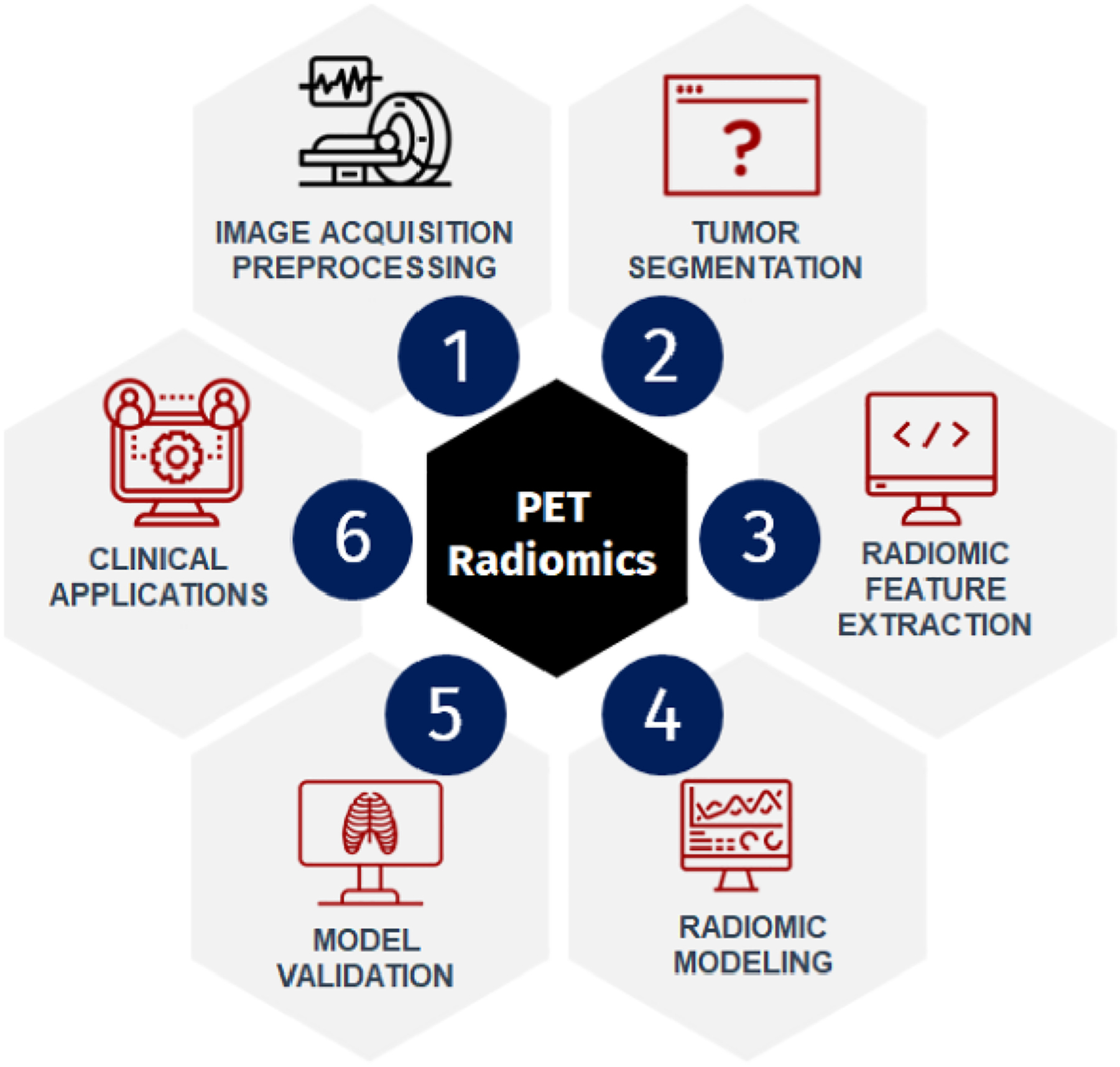
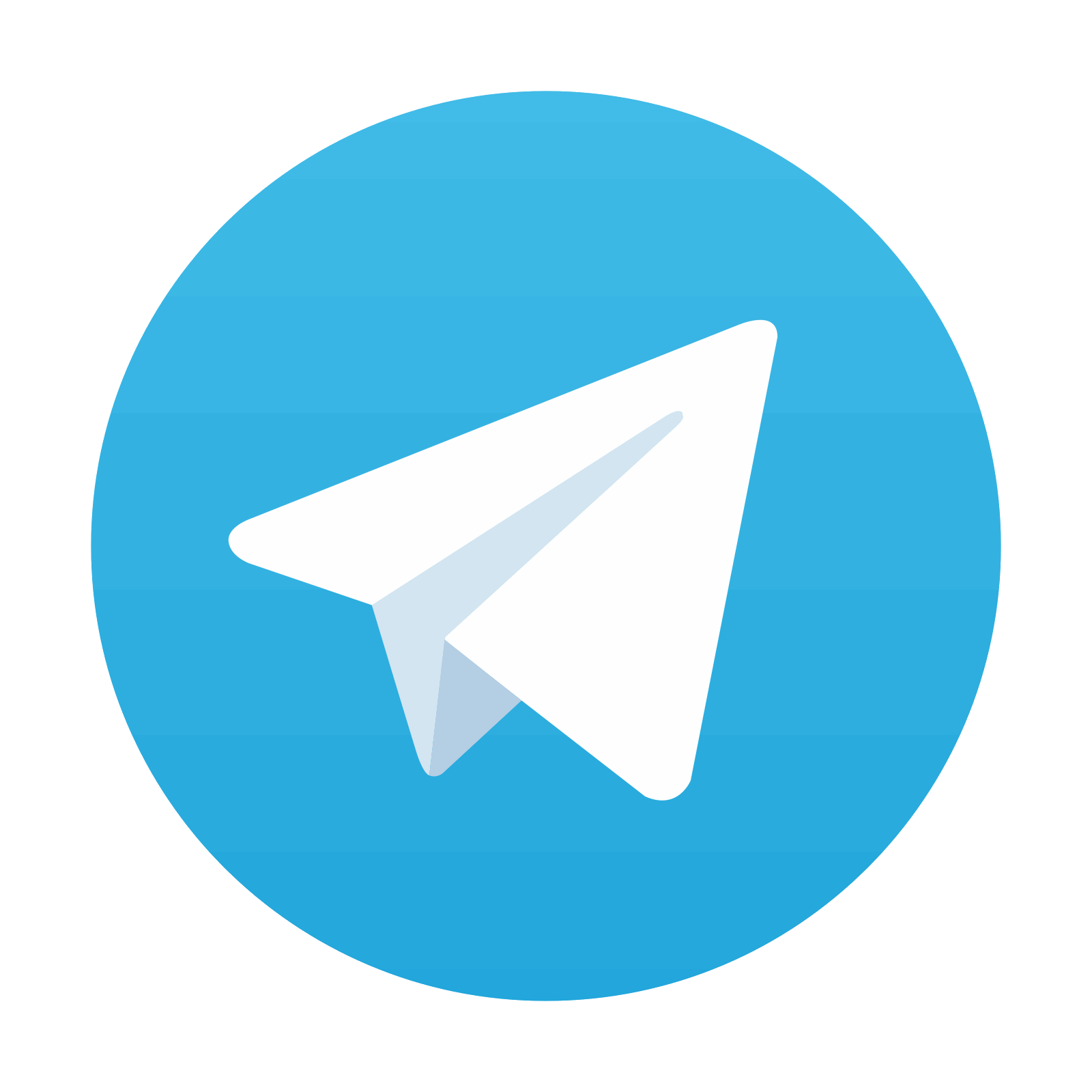
Stay updated, free articles. Join our Telegram channel

Full access? Get Clinical Tree
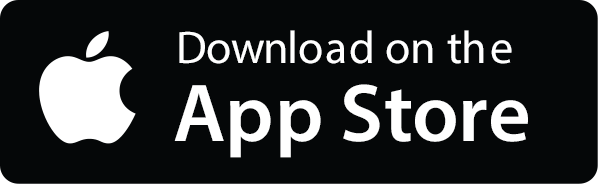
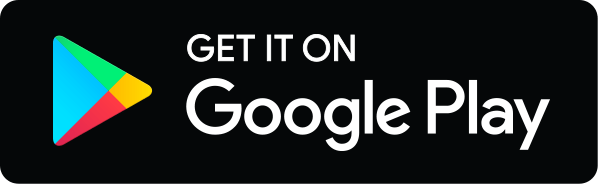
