The proximal origin of the rectus femoris from the anterior superior iliac spine, anterior inferior iliac spine and the supraacetabular sulcus comprises the proximal tendinous complex. A practical MR imaging-guided approach adapted to the anatomy of the proximal tendinous complex and the myoconnective transitions along the rectus femoris is critical in accurately evaluating and following rectus femoris injuries. This review presents various conditions, including avulsions, tendon tears, and myoconnecive lesions along the muscle that can result in loss of function and pain. Furthermore, different pathologies or “abnormal states” that can present with symptoms similar to myoconnective lesions are demonstrated.
Key points
- •
Accurate MR imaging assessment of the rectus femoris relies on a comprehensive anatomic knowledge and specific acquisition protocols, both of which are essential for accurately diagnosing rectus femoris proximal tendinous complex issues associated with groin or hip pain.
- •
A diverse range of conditions, from overuse, delayed onset muscle soreness, or myoconnective injuries, can affect athletes at all levels and can be differentiated by specific imaging features discernible through MR imaging.
- •
Implementing a practical MR imaging–guided approach facilitates communication and the customization of therapeutic options, thereby ensuring personalized treatment plans tailored to the specific clinical context of each patient.
- •
MR imaging serves as a vital tool for monitoring post-injury healing, offering valuable insights into treatment effectiveness, and identifying potential residual complications, such as pseudotumors, subspine impingement, heterotopic ossifications or reinjuries, thus optimizing patient care and outcomes.
Introduction
A muscle injury in adults is a myoconnective injury that typically occurs during sports and affects the athlete’s functional capacity and ability to continue with the activity. , Muscle injuries are the most common injuries in sports, accounting for more than 30% of injuries in elite male and 24% in elite female football players. Furthermore, they constitute approximately a third of total time loss from injuries. ,
The quadriceps is the second most injured group in both male and female cohort football players, second only to hamstring injuries and accounting for approximately 4.6% of all football injuries in males and 11% in females. Quadriceps injuries have a reinjury incidence of approximately 17%. Among the quadriceps muscles, the rectus femoris (RF) is the most commonly injured. Although published data are scant in male footballers, RF injuries represent over two-thirds of all quadriceps injuries in females. , ,
The RF forms the anterior layer of the quadriceps and is the sole biarticular muscle of this group. It is mainly responsible for knee extension and, to a lesser extent, hip flexion. It is integrated into a functional system of muscles, joints, and osseous structures, extending from the spine to the foot, which stabilizes and orients the knee in various complex movements and stabilizes the pelvis in the standing position. When the knee is fully extended and the hip is flexed, the RF shortens. As a consequence, hip extension is limited, resulting in active insufficiency of the RF. On the other hand, when the knee is fully flexed and the hip is extended, the RF is at its greatest length and hip flexion is limited, which results in passive insufficiency.
RF injuries in elite athletes may present either acutely or with insidious symptoms owing to the complex anatomy and biomechanics of the RF. This article will describe the imaging strategies, risk factors, and the role of MR imaging in determining prognosis and appropriate rehabilitation of RF injuries in elite athletes with a focus on MR imaging of myotendinous and myofascial injuries.
Clinical presentation/mechanism of injury
Depending on the mechanism, injuries can be classified as indirect (or noncontact) due to elongation; direct due to direct impaction, contusion, or laceration; and compartment syndrome secondary to elevated compartmental pressures. RF injuries are predominantly indirect injuries occurring during sprinting, abrupt changes of direction, or kicking activities.
Analysis of kicking and sprinting biomechanics has demonstrated that the RF decelerates the tibia in the swing phase by eccentric contraction with maximum elongation becoming a risk moment of injury. On the other hand, in sprint deceleration, the more erect posture and posterior leaning increase eccentric loading on the RF. , ,
Della Villa and colleagues studied injury mechanisms and situational patterns of 9 severe quadriceps muscle injuries in male soccer players. They suggest that the dominant leg is susceptible to high-grade quadriceps muscle injuries. In addition, using video analysis of 20 high-grade RF injuries, Jokela and colleagues demonstrated that the injuries are more common in offensive situations likely due to the high incidence of kicking injuries.
On the other hand, in volleyball, repetitive explosive jumping exerts tremendous stress on the direct tendon (DT) of the RF muscle and can cause enthesitis. Specifically, volleyball players that play central defensive positions (blockers) are prone to the development of enthesitis of the DT of the RF.
In cases of injuries not associated with physical activity, certain drugs (steroids, fluoroquinolone, among others) as well as a history of diabetes, gout, systemic lupus erythematosus, rheumatoid arthritis, kidney failure, and obesity may all be predisposing factors for muscle injuries.
Generally, in acute injuries, symptoms (sudden pain and impaired ipsilateral limb function) often occur almost immediately after the injury, but in some instances, symptoms may have an insidious onset. Furthermore, a tear may remain nearly asymptomatic because of recruitment of other quadriceps muscles. For this reason, MR imaging plays an essential role in determining the presence, location, extent, and severity of an RF injury and, consequently, in guiding proper therapeutic management.
MR imaging techniques
The main objective of diagnostic imaging is the evaluation of the extent of connective tissue involvement, because the prognosis of a muscle injury appears to be directly related to the degree of affectation of the connective tissue. ,
MR imaging has become the imaging modality of choice for accurately analyzing the connective tissues. It is recommended to use a multichannel multipurpose surface coil to ensure higher resolution images. The patient is placed in a supine position and a skin marker should always be placed to indicate the site of symptoms thus ensuring adequate coverage and, in the case of multiple imaging findings, aiding in determining the clinically relevant lesion.
Some MR imaging centers perform protocols with initial wide field-of-view images that include both hips and thighs; these images serve to accurately localize both acute and chronic injuries and allow evaluation of the contralateral hip and thigh both for comparison (eg, of muscle bulk) and to assess for occult additional injuries. Subsequently, high-resolution multiplanar acquisitions (axial, coronal, sagittal, and sagittal oblique) with thin sections (eg, <4 mm), and small field of view (eg, <30 × 30 cm) are obtained of symptomatic RF. The sagittal oblique images (paralleling the anterior inferior iliac spine (AIIS)) have been shown be useful when assessing very proximal tendinous injuries.
It is helpful to perform fluid-sensitive sequences (intermediate-weighted) with an intermediate echo time (eg, <65 ms) to obtain adequate contrast and spatial resolution of the connective tissues. These sequences enable the detection of edematous changes around the myotendinous junction (MTJ) and the myofascial unit (MFU), ensuring an accurate connective tissue assessment, as well as allowing the delineation of intramuscular or intermuscular fluid collections or hematomas. T1-weighted and gradient-echo sequences are useful in the assessment of subacute hemorrhage or hematoma, in the detection of atrophy and fatty infiltration, and in the detection of scar tissue in chronic injuries that may present chemical shift artifacts, especially on gradient echo sequences. , ,
MR imaging anatomy
Proximal Tendinous Complex
The proximal origin of the RF consists of a proximal tendinous complex (PTC), which is formed by the DT and the indirect tendon (IT), that then converge into a common tendon (CT). , , , ,
The DT originates from the AIIS and the underlying rough surface. It is formed of fibers with a longitudinal craniocaudal direction and a slight medial inclination with respect to the long axis of the muscle. On MR imaging, the DT is mainly hypointense ( Fig. 1 ). Its MTJ lies very proximal in the thigh, and its fibrillar tendinous structure is distributed along the anterior surface of the muscle, continuing distally as the anterior aponeurosis and the MFU. , , , The main function of the DT component of the RF occurs at the beginning of hip flexion.
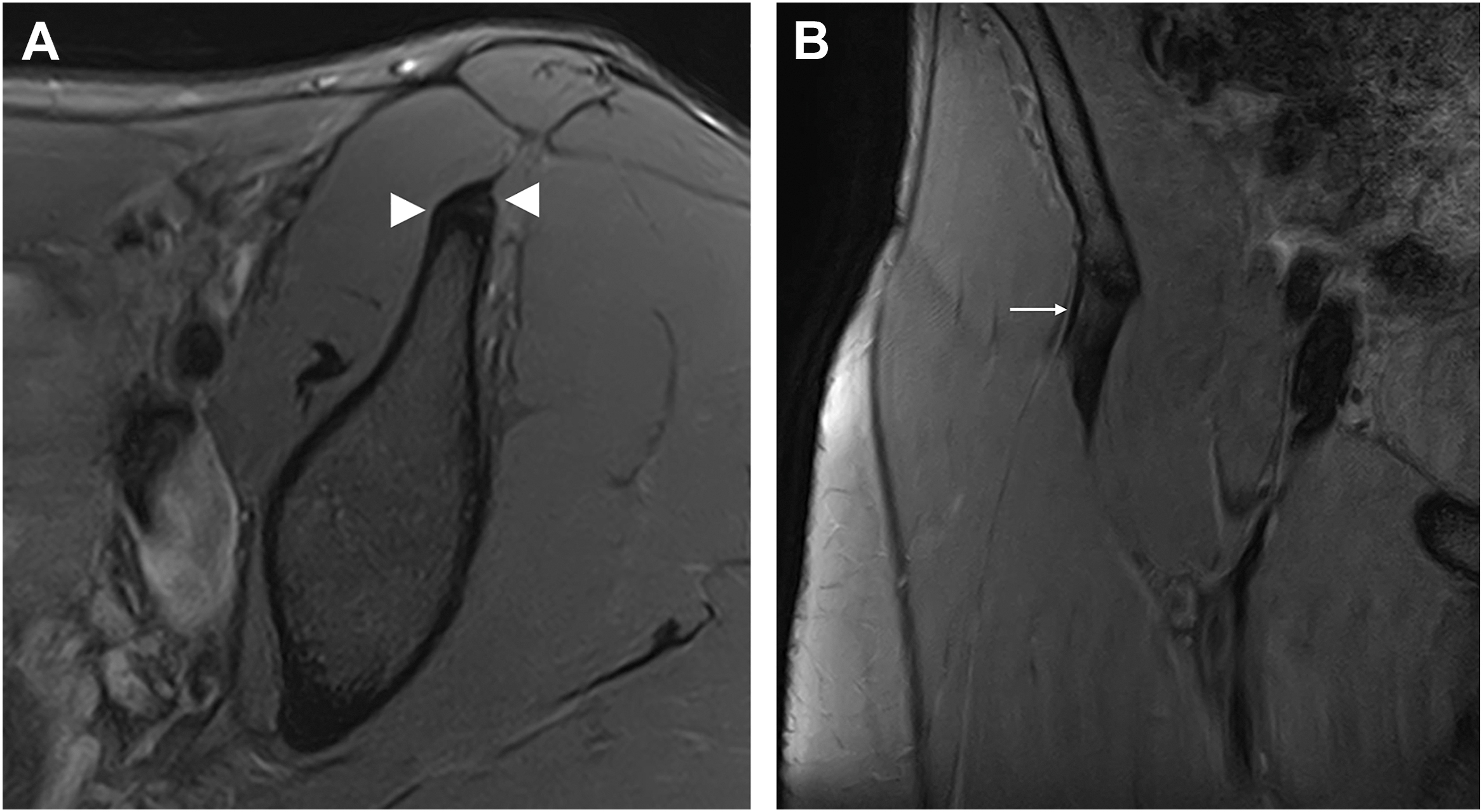
The IT has a wide origin from the supraacetabular sulcus and the lateral aspect of the hip joint capsule. This tendon is the largest proximal tendon and develops prior to the DT; embryologically, until the sixth fetal month, only the IT can be distinguished. , , The IT has a triangular morphology, follows a posteroanterior course in the axial plane ( Fig. 2 A, B ), and is closely related to the hip joint capsule ( Fig. 2 ). It usually has a medial inclination with respect to the longitudinal axis of the muscle. The main function of the IT occurs once hip flexion has begun.
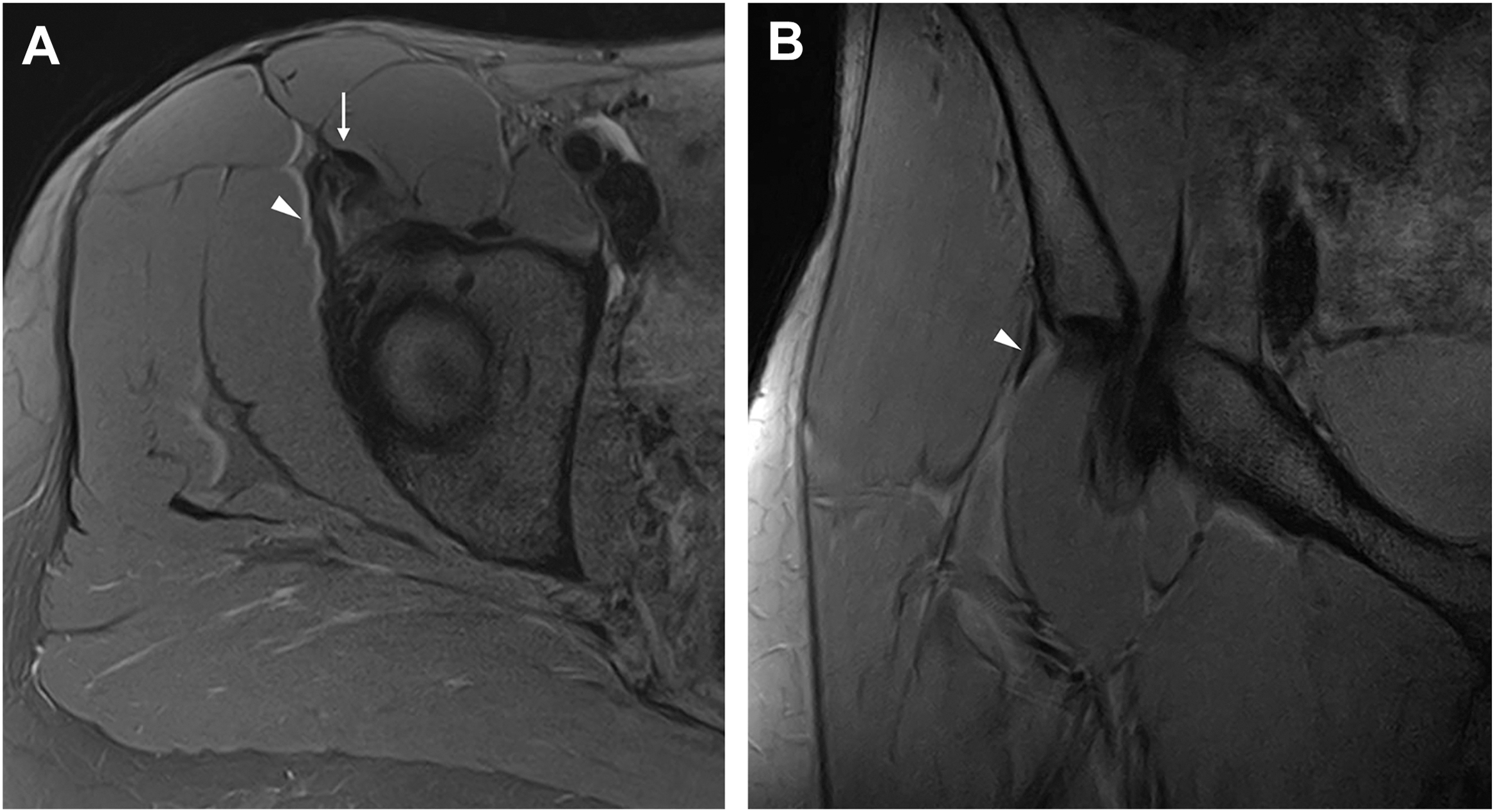
The DT and IT join to form the CT approximately 2 cm from the origin of the DT and 5 cm from the origin of the IT. Between 10% and 20% of the CT fibers are an indistinguishable fusion of fibers from the DT and IT whereas the DT and IT contributions to the CT can be distinguished in the remainder of the CT.
The complex proximal anatomy of the RF results in a PTC with a Y-shaped tridimensional structure which is then covered by a common paratendon. , , , ,
An additional constant contribution to the origin of the PTC has recently been described. It is a membrane that connects the CT to the anterior superior iliac spine (ASIS). In 1875, Macalister described it as an anatomic variant, but in an anatomic study carried out with 42 hemipelves, the membrane was found to be present in all cases as a constant component. In the majority of the 42 hemipelves, the membrane was thick, in the anteroposterior axis its anterior margin was at the same level as the ASIS or more anterior and extended to the distal portion of the CT. With respect to it, this membrane demonstrates a lateralized inclination while the PTC demonstrates a medial inclination. Therefore, it has been proposed that the membrane may play a role in stabilizing the tendon complex. On MR imaging, the membrane is visualized as a thin hypointense line anterior to the PTC and closely related to the sartorius and iliac muscles ( Fig. 3 ).
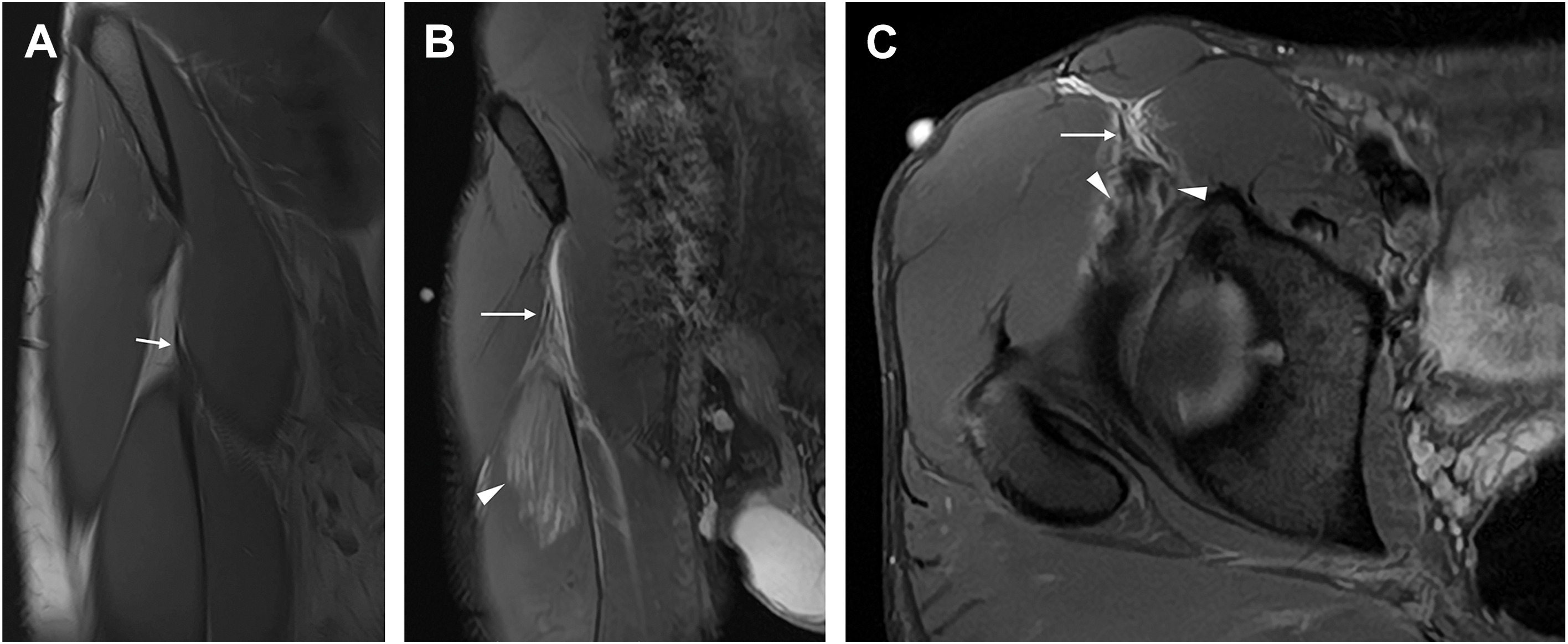
Many variations from the classic description of the anatomy of the RF have been described including at the level of the origin, muscle belly, distal insertion, and perimuscular region. Most of the variations affect the origin. A third tendon originating from the IT constitutes one of these variations at the origin , ; although not always visualized, according to Tubbs and colleagues, it is frequently present (83%). The third tendon, also known as the capsular head, originates from the IT and typically has a bandlike shape. It follows an inferolateral course forming an angle of approximately 60° with respect to the long axis of the IT and inserts along the anterior surface of the greater trochanter between the insertion of the gluteus minimum tendon and the iliofemoral ligament ( Fig. 4 ). , Armstrong and colleagues have considered that the posterior aspect of the intramuscular extension of the IT (also known as central septum more distally, see below) belongs to the third tendon or capsular head.
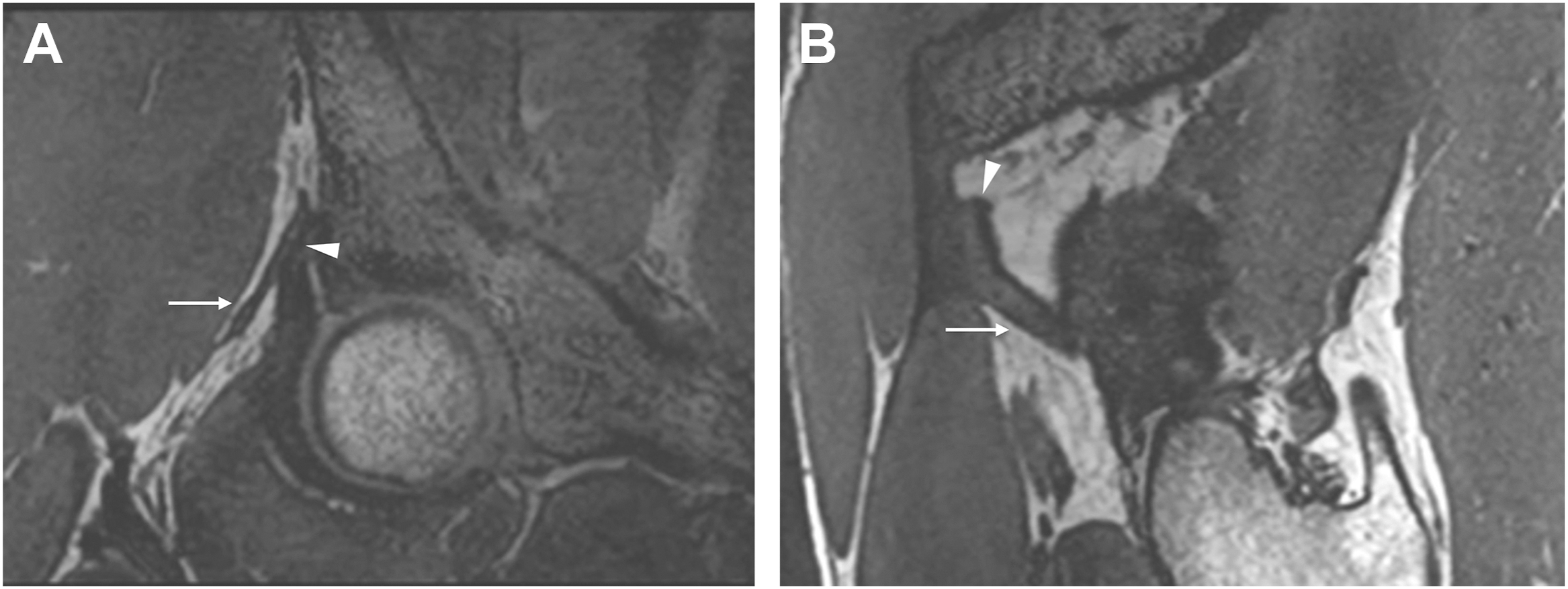
Proximal Myoconnective Transitions
The DT myoconnective junction (MJ) (or MTJ) is located very proximally in the thigh, and its fibrillar tendinous structure is distributed along the anterior surface of the muscle continuing distally as the anterior aponeurosis ( Fig. 5 ) and MFU. , , ,
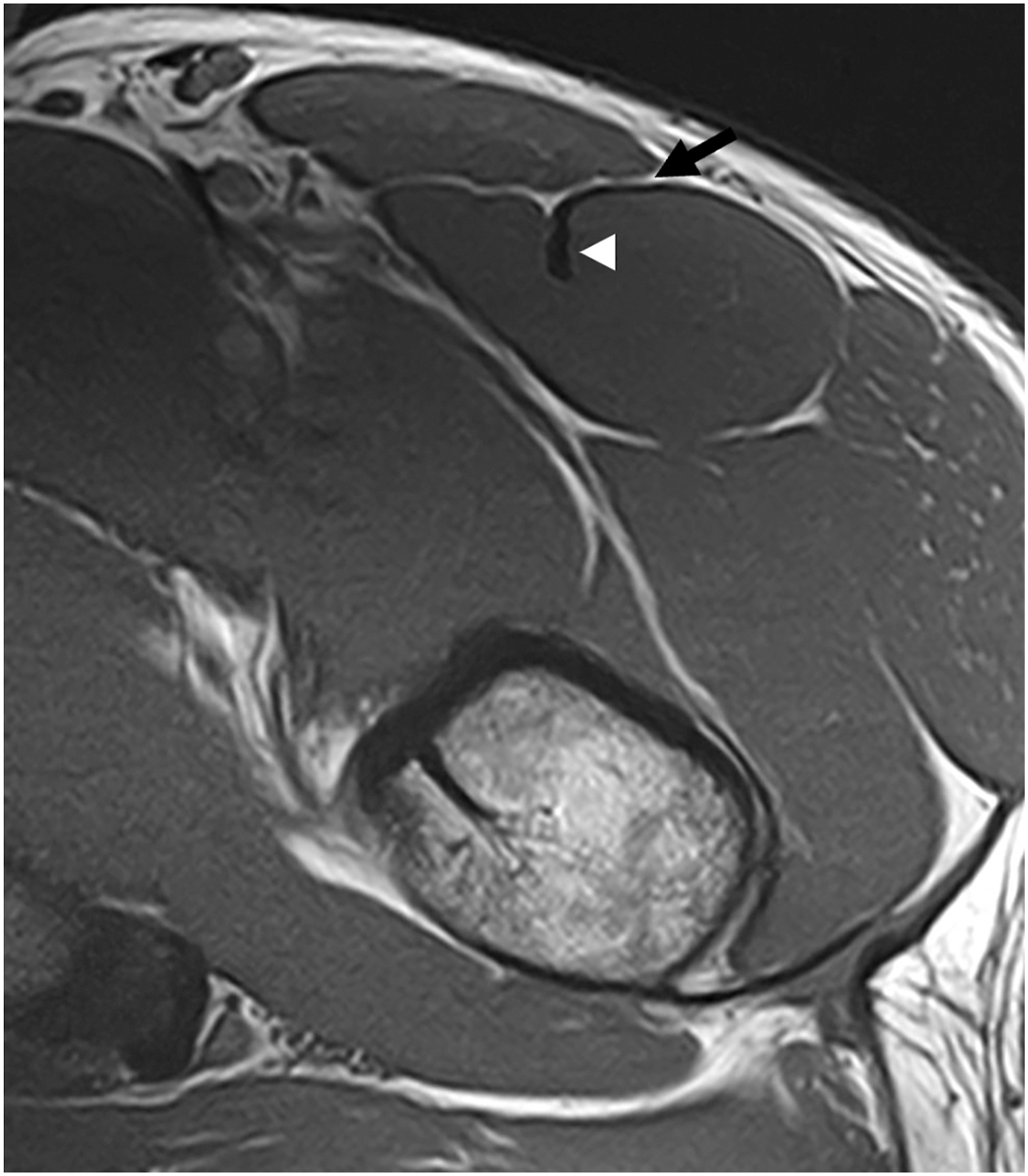
The IT extends along the anterior midline of the muscle and subsequently forms the central septum of the RF, which thins out in the lower third of the thigh, acquiring a thin linear shape in the sagittal plane with an elongated bipennated MJ (or MTJ) (see Fig. 5 ). , , , , , , , , ,
Distal Myoconnective Transitions
The distal myoconnective transition of the RF is located along the posterior aspect of the muscle belly. The proximal third of the distal MFU is usually indistinguishable on MR imaging due to the thin nature of the fascia. This proximal third is said to have a structural role. In the middle third of the distal myoconnective transition, connective tissue and the myoaponeurotic junction are usually identified on the posterior surface of the RF on MR imaging. In the distal third, the thickness of the aponeurosis increases and forms the distal MTJ which can be clearly identified on MR imaging ( Fig. 6 A–C ).
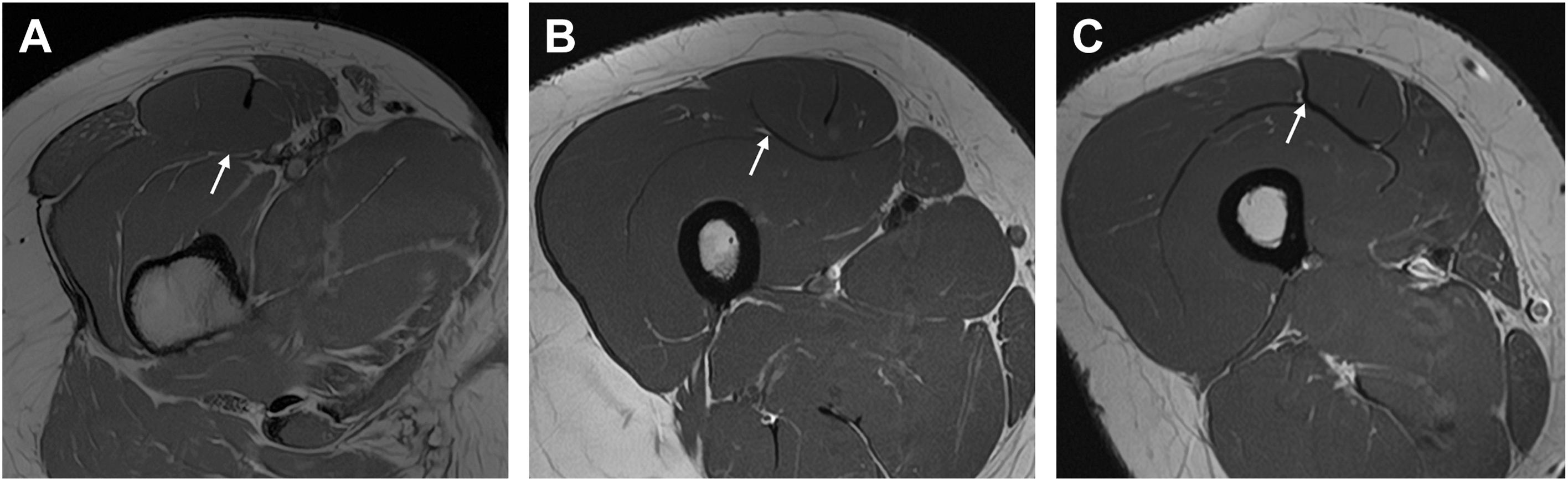
Injuries
Generalities
Enthesitis of the DT of the RF muscle is a rare pathology, caused by overuse and repetitive microtrauma. Microtrauma causes tendon micro-lesions which, despite subsequent tissue repair, lead to tendon degeneration. This results in a structural weakening of the tendons which become subject to partial or complete tears. On both MR imaging and ultrasound, enthesitis is characterized by a thicker and inhomogeneous DT due to the presence of areas of myxoid degeneration and, occasionally, calcifications.
As already indicated, muscle injuries in adults typically affect the MJ, which is made up of the MTJ and the MFU or myoepimysial unit. These 2 components are a continuum of different types of connective tissue and the related muscle fibers. The MTJ corresponds to the interface between the muscle and the tendon (and aponeurosis) and is the most frequently affected structure. It contributes mainly to the transmission of force instead of providing structural muscle support such that injuries in this area lead to a greater degree of disability than those in other areas. The MFU is adjacent to the MTJ. The MFU contributes more to overall muscle structural support than to mechanical force transmission.
In skeletally immature athletes, the most fragile point in the muscle-tendon-bone chain is the unfused physis. The fusion of the secondary ossification center of the AIIS occurs at approximately 16 years of age. Therefore, patients under 16 years of age are at higher risk of suffering bone/apophyseal avulsions than myotendinous injuries. The AIIS avulsion is the second most common type of apophyseal avulsion injury in the pelvis, surpassed only by hamstring (ischial tuberosity) and followed by sartorius (ASIS) avulsion injuries. ,
Injuries at the Rectus Femoris Origin
Direct tendon ( ± osseous or periosteal avulsion)
The DT injury is characterized by a partial or complete tear with different orientations: transverse, longitudinal or mixed. Fiber retraction is only evident in tears with a transverse or mixed component ( Fig. 7 ).
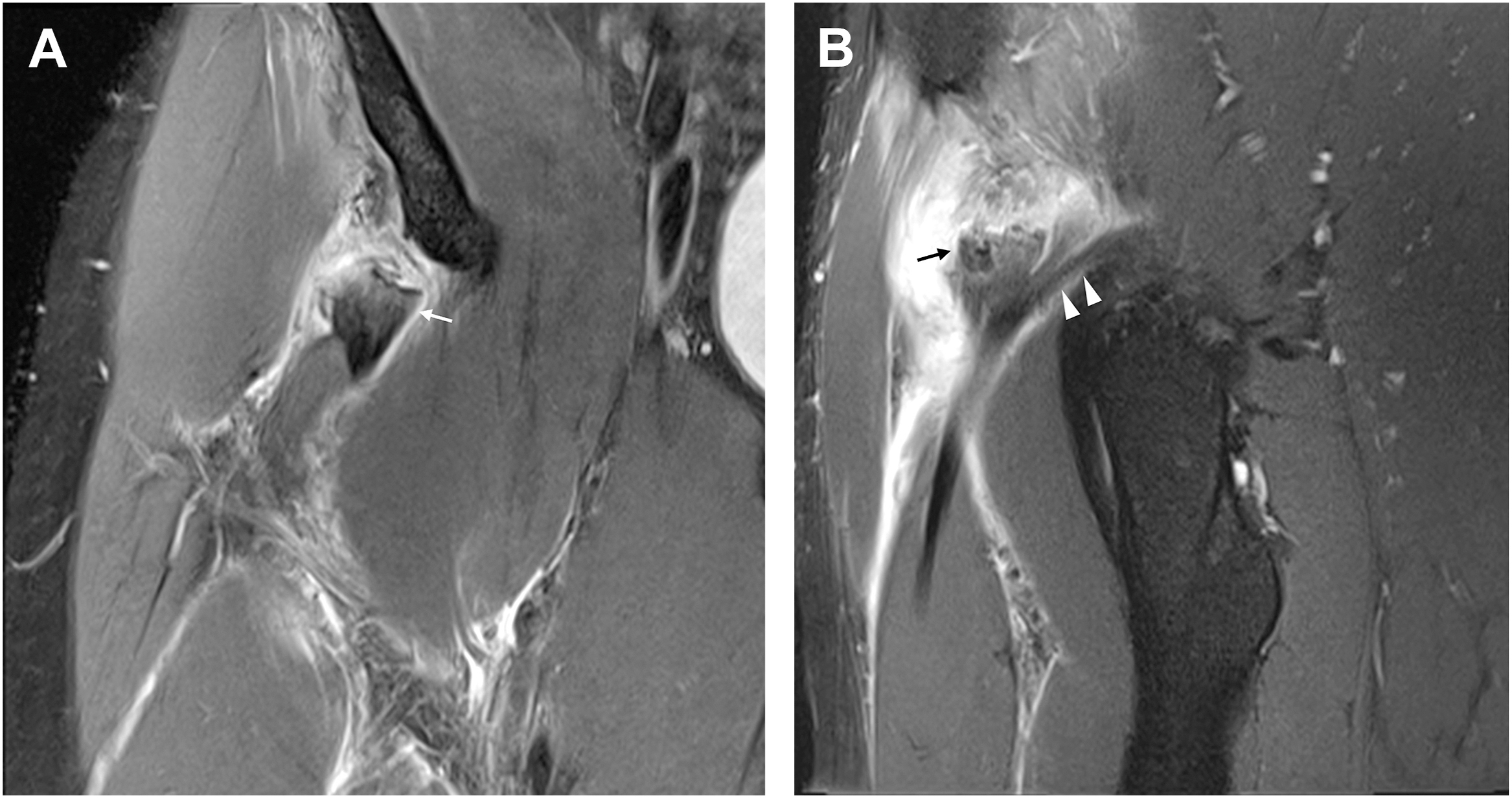
In avulsions of the AIIS, anterolateral separation of the secondary ossification center (apophysis) is seen ( Fig. 8 ). Occasionally, as opposed to a significant avulsion of the AIIS, a microavulsion or periosteal avulsion occurs and results in a subperiosteal hematoma that can track cranially along the iliac fossa ( Fig. 9 ).
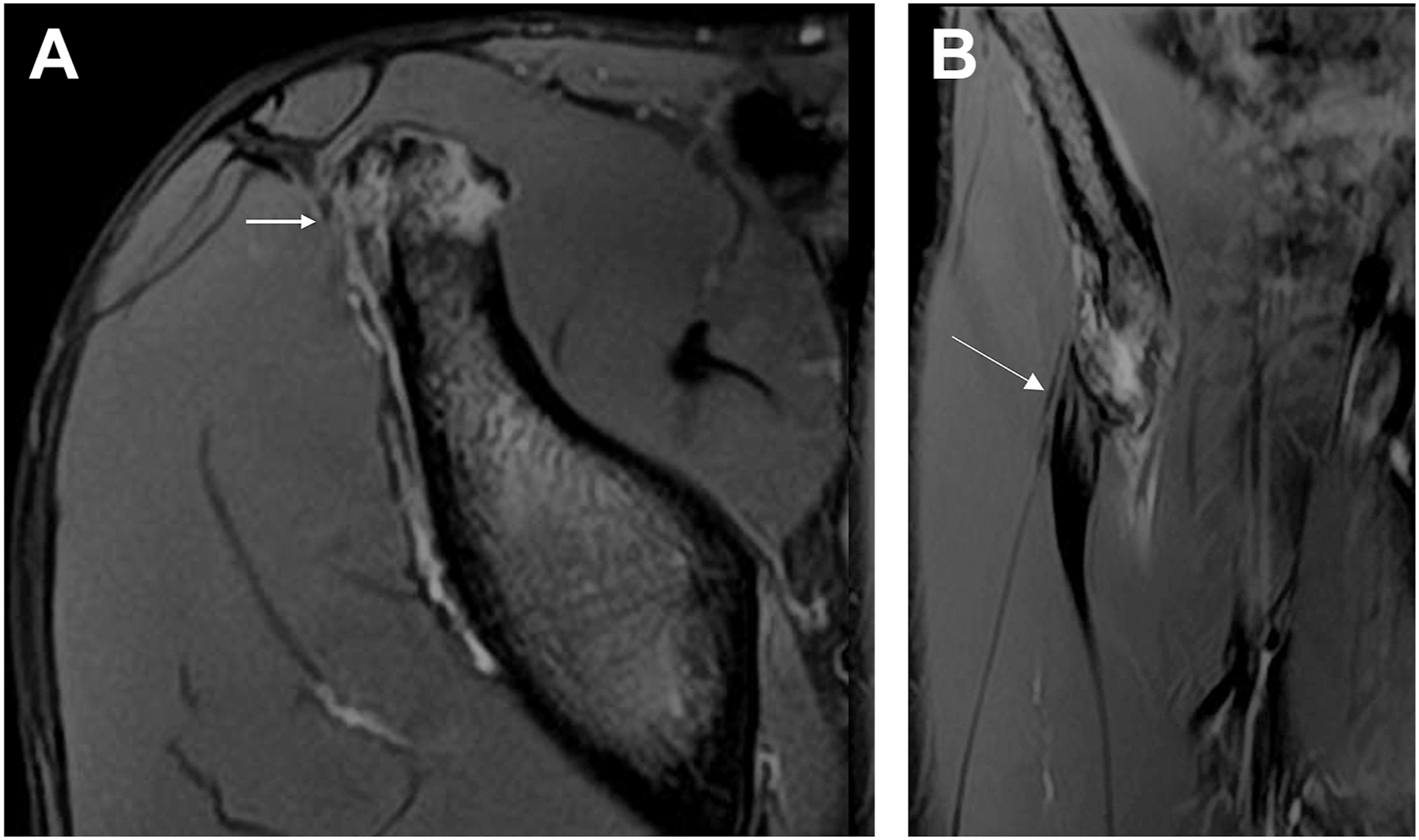
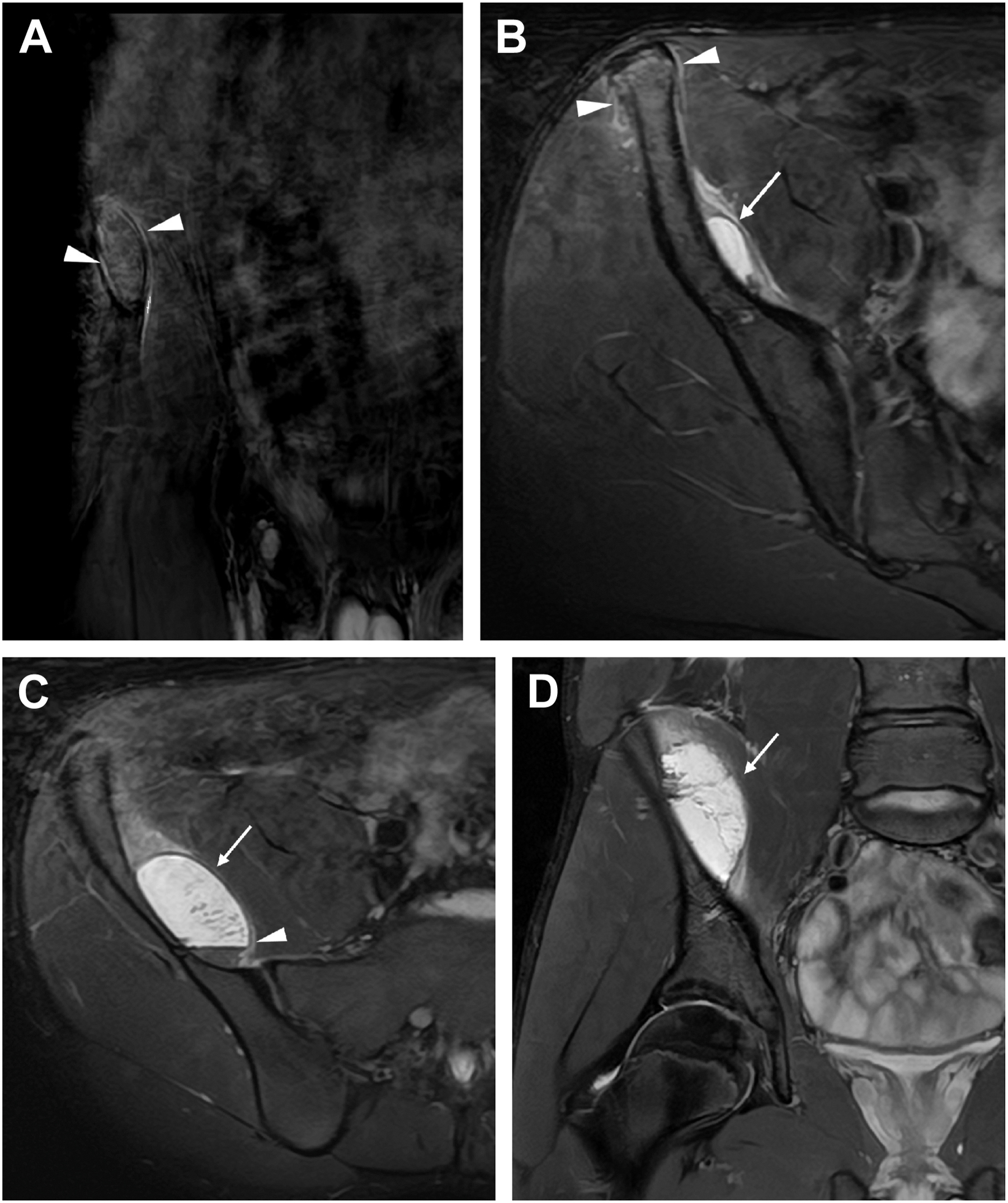
Indirect tendon
Although complete or partial tears of the IT have been reported to be more frequent than direct and CT injuries, the authors’ experience does not support that suggestion. Given the frequent mild heterogeneity of the magnetic resonance signal in the proximal IT due to its close relationship with adjacent adipose tissue and the iliofemoral ligament and potential inhomogeneous fat saturation, signal changes may be misinterpreted as tendon injuries and this may increase the reported incidence of injury. Isolated injuries of the IT are uncommon.
Indirect RF tendinopathy can be related to hip pathology. Since the IT merges posteriorly with the hip joint capsule, tendon damage may extend to the superior capsule and labrum. Conversely, superior capsular damage may extend to the IT. In cases of hip arthritis, paralabral cysts and synovial cysts may develop around or within the IT, leading to tendinopathy.
Common tendon
Injury to the CT can be partial or complete tear. Fiber retraction is only evident in tears with a transverse or mixed component.
Combined
Injuries to the origin of the RF are usually injuries that affect several tendon structures ( Fig. 10 ). Some have suggested that proximal RF origin injuries may extend from the indirect to the DT and then to the CT. On the other hand, Moraux and colleagues reported that any pattern of tendon tear may be encountered, with a partial or total tear of the DT or IT often extending to the conjoined tendon.
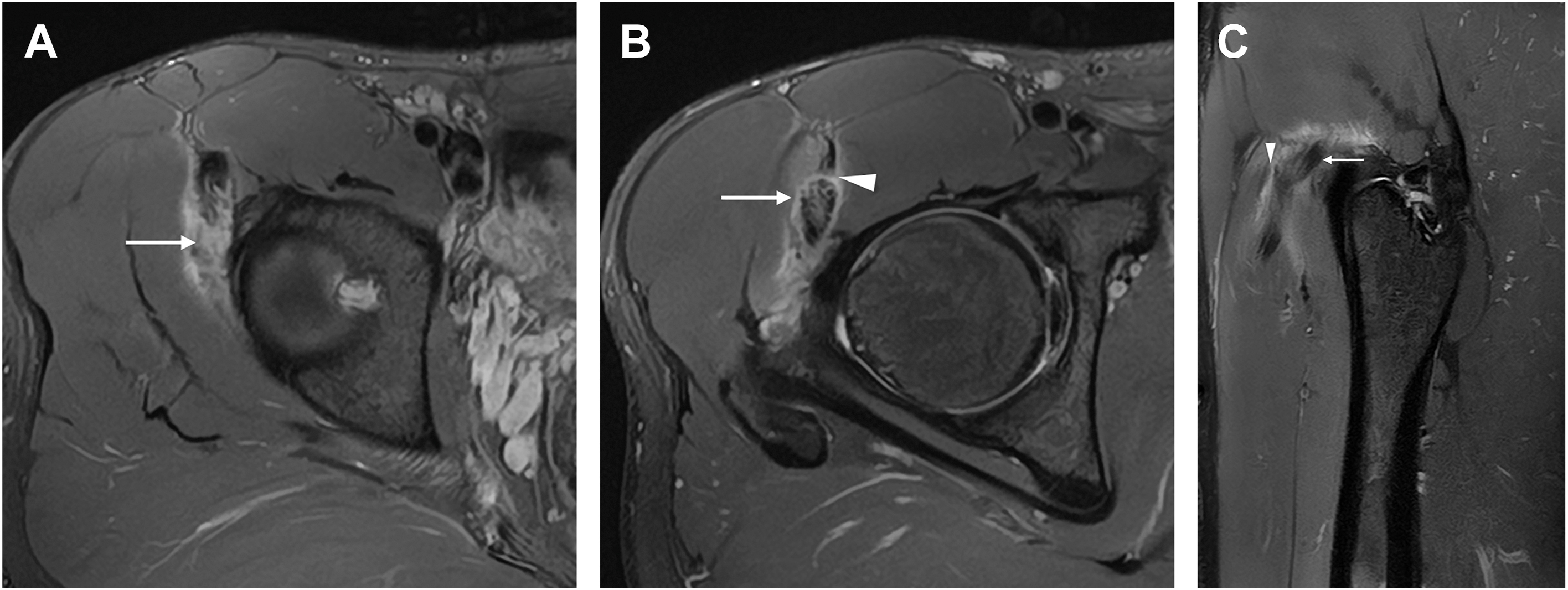
Proximal-Thigh Injuries
If the affected MJ is the proximal one, the injuries are typically located along the anterior aspect of the muscle. These can be tendinous, myotendinous, aponeurotic, myoaponeurotic, or myofibrillar injuries, or a combination thereof.
These injuries can be classified as
- 1.
Tendinous/aponeurotic injuries: These are injuries with connective tissue involvement, often with an observable gap ( Fig. 11 ). The central septum is a thick connective tissue and is considered a tendon, so its injuries are considered tendinous injuries. It is very common to find tears that affect the posterior portion of the central septum. Some consider the posterior portion of the central septum to be related to the third RF tendon. Injuries to the thin anterior peripheral connective tissue are considered aponeurotic injuries.
