Radiation therapy for head and neck cancer is a cornerstone of treatment but can come with significant and lasting complications. Reduced vascularity and fibrosis heighten the risks of stroke, infection, and diminished quality of life. Advances in imaging technologies, such as fluorodeoxyglucose PET and sodium fluoride-PET, have emerged as critical tools for diagnosing and monitoring vascular inflammation and calcification, enabling earlier interventions and improved patient management. This review examines the pathophysiology of RT-induced damage, with a focus on vascular and connective tissue complications, and highlights the evolving role of PET imaging in early detection and management of these effects.
Key points
- •
Radiation therapy is a key part of the management of head and neck malignancies but can be associated with significant risks of acute and late toxicities.
- •
Fluorodeoxyglucose PET and sodium fluoride PET can enable the diagnosis and monitoring of radiation-induced vascular toxicities.
- •
Earlier detection and improved monitoring of radiation-induced vascular complications, such as for carotid artery stenosis (CAS) and atherosclerosis, may allow for earlier interventions and improve patient quality of life.
CAS | carotid artery stenosis |
CCRT | concurrent chemo-radiotherapy |
CNS | central nervous system |
CT | computed tomography |
F-BPA | fluoride-labeled boronophenylalanine |
FDG | fluorodeoxyglucose |
HNC | head and neck cancers |
IL | interleukin |
NaF | sodium fluoride |
ORN | osteoradionecrosis |
PNS | perineural spread |
RN | radiation-induced necrosis |
RT | radiation therapy |
Introduction/history/definitions/background
Head and neck cancers (HNC) are among the most common malignancies worldwide and a common cause of cancer-related morbidity and mortality ( Figs. 1–7 ). A common treatment approach for HNC is radiation therapy (RT), either definitively with or without systemic therapy or as adjuvant therapy following surgery. Although RT is an effective modality for HNC, it can cause significant adverse complications. The acute effects of RT are largely dependent on the duration of radiation exposure and, therefore, may be managed by altering the therapy schedule. Chronic effects, however, are more so radiation dosage-dependent. The most accepted theory explaining the impact of RT on late complications focuses on the resultant increased fibrosis and decreased vascularity that can lead to hypoxia and various tissue necrosis, infection, and ulceration. The damaging effects of radiation therapy may not be clinically apparent for months or even years after treatment is administered. The complications of RT discussed in this article are categorized as resulting from damage to salivary glands, vascularity, connective tissue, and endocrine or immune function. These side effects present lifelong challenges to patients and their caregivers and require longitudinal strategies to mitigate their damages to quality of life and basic functioning.
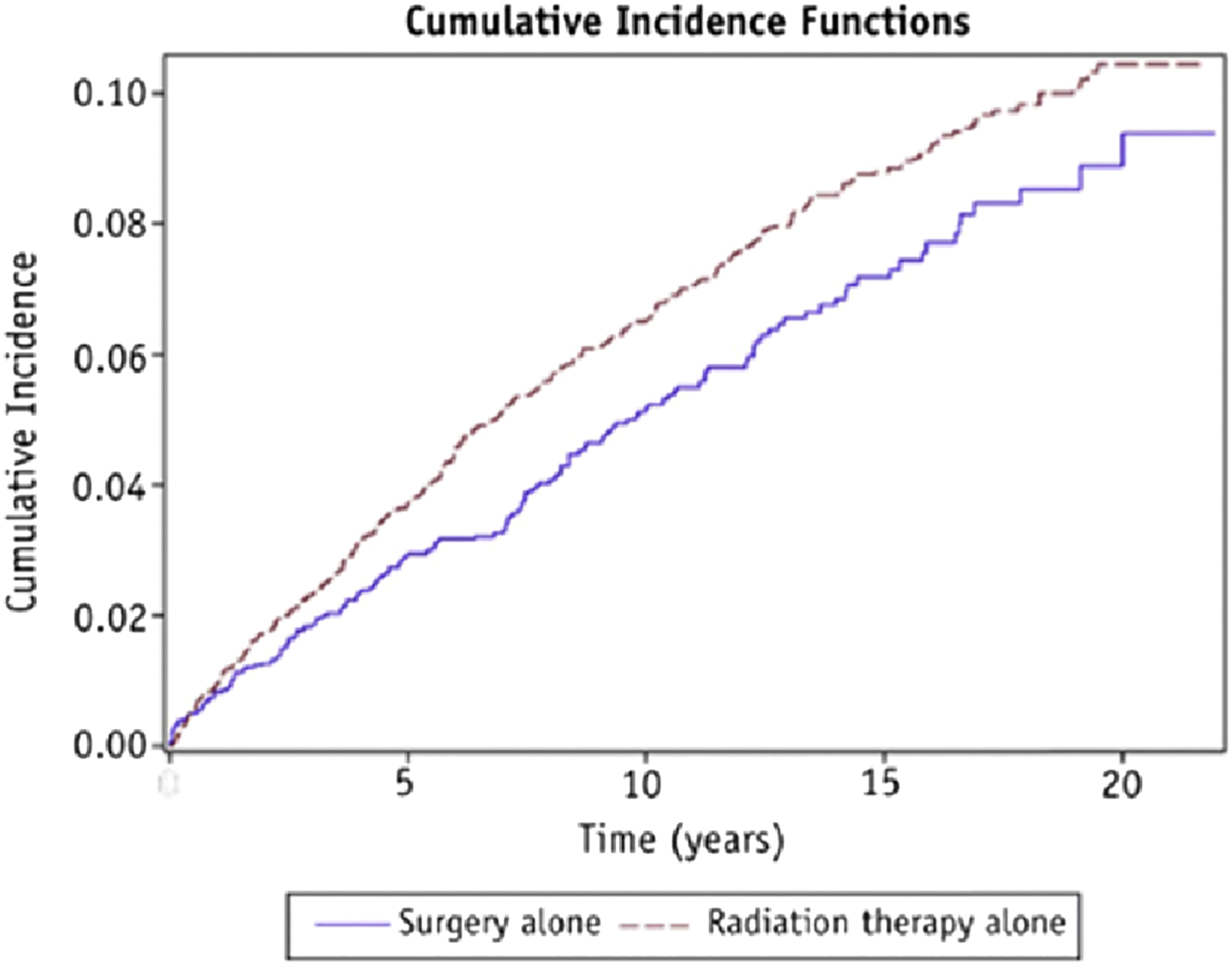
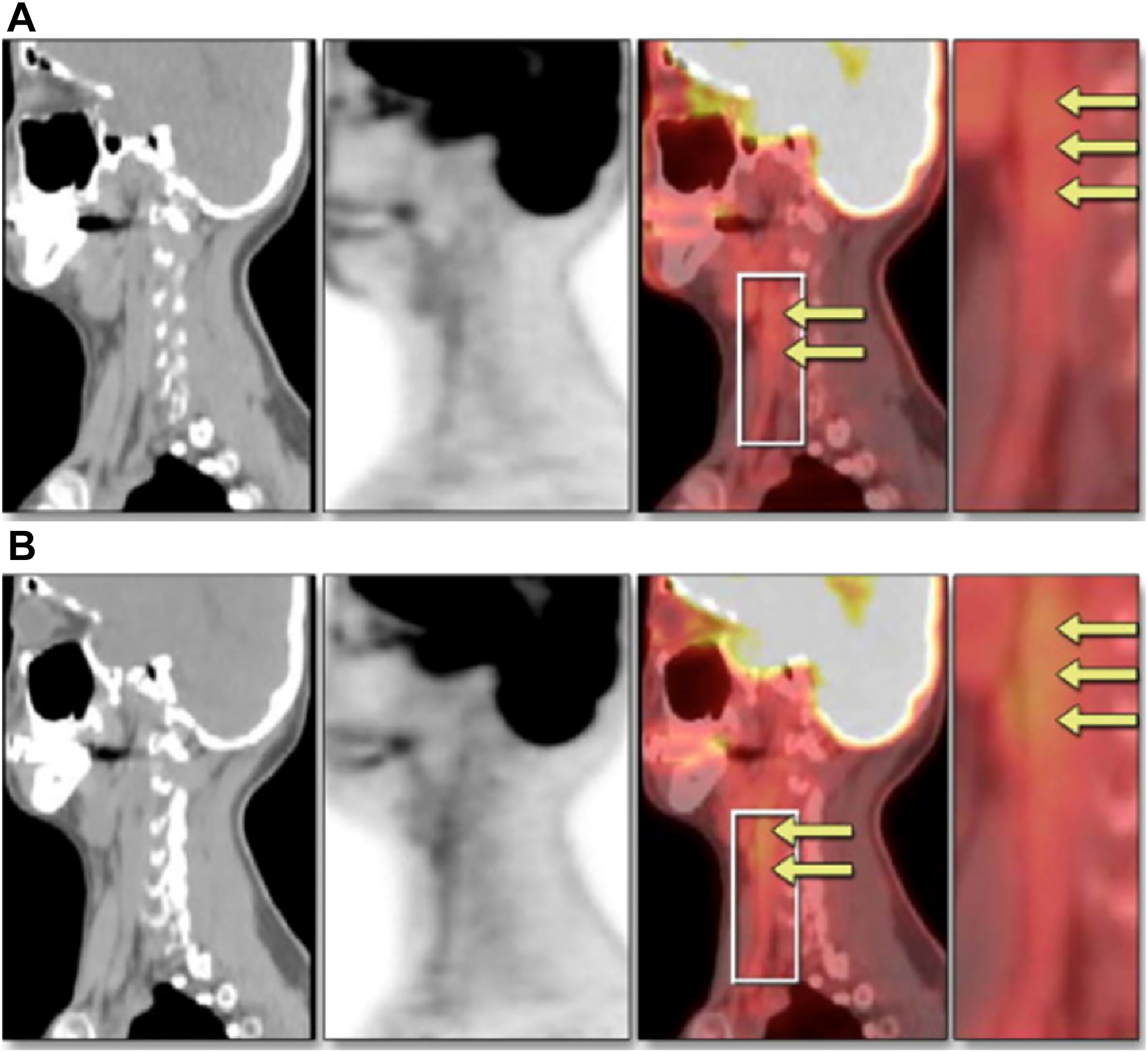
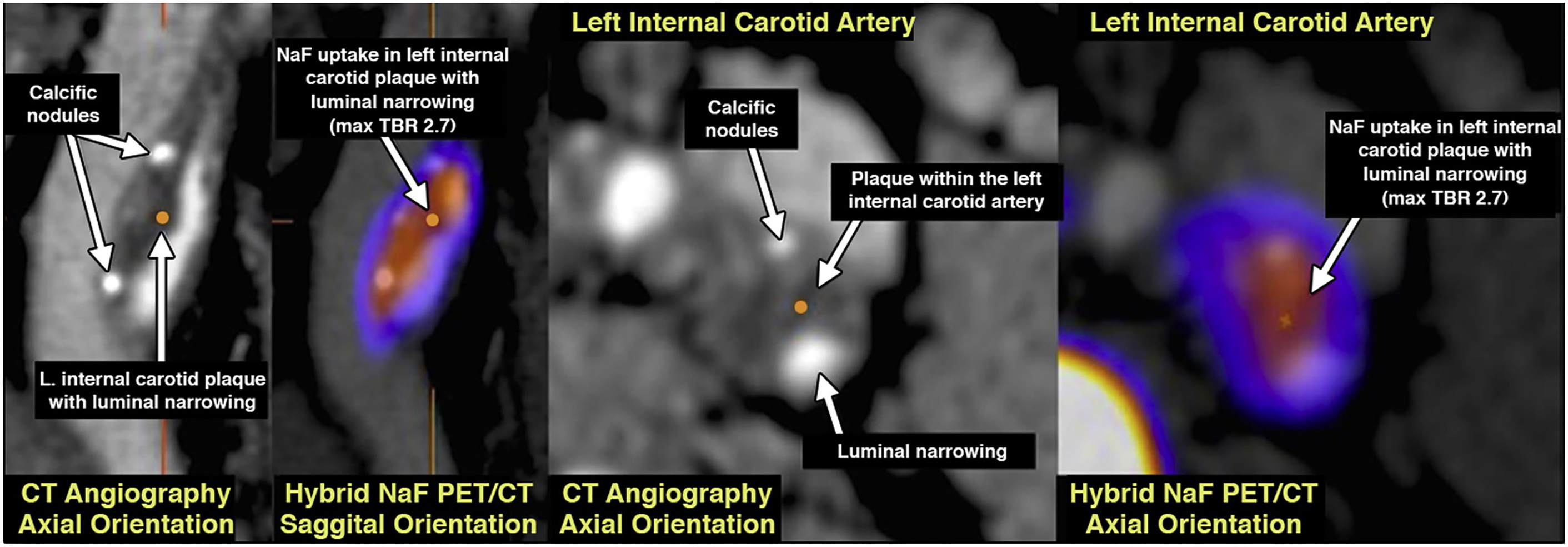
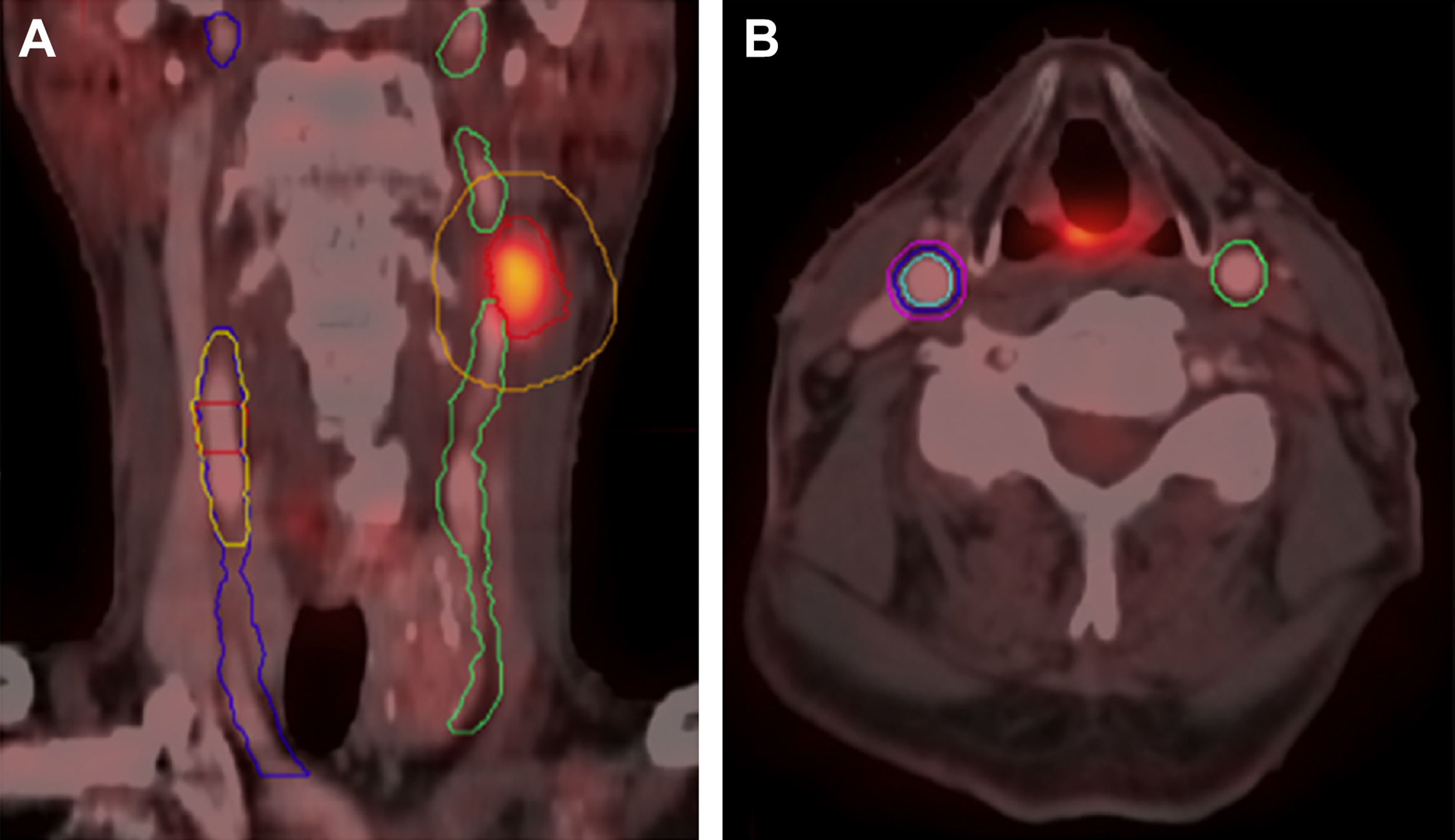
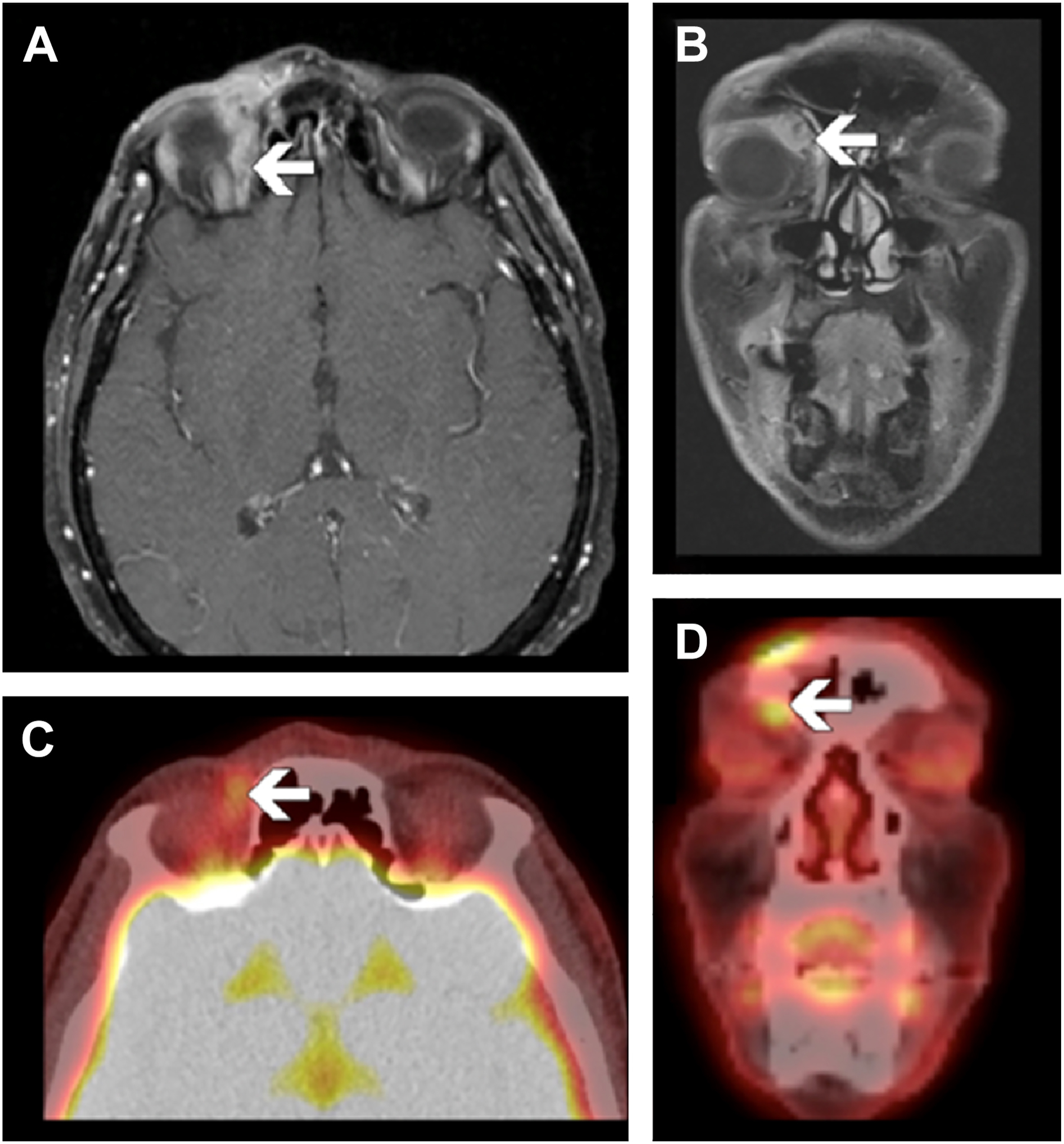
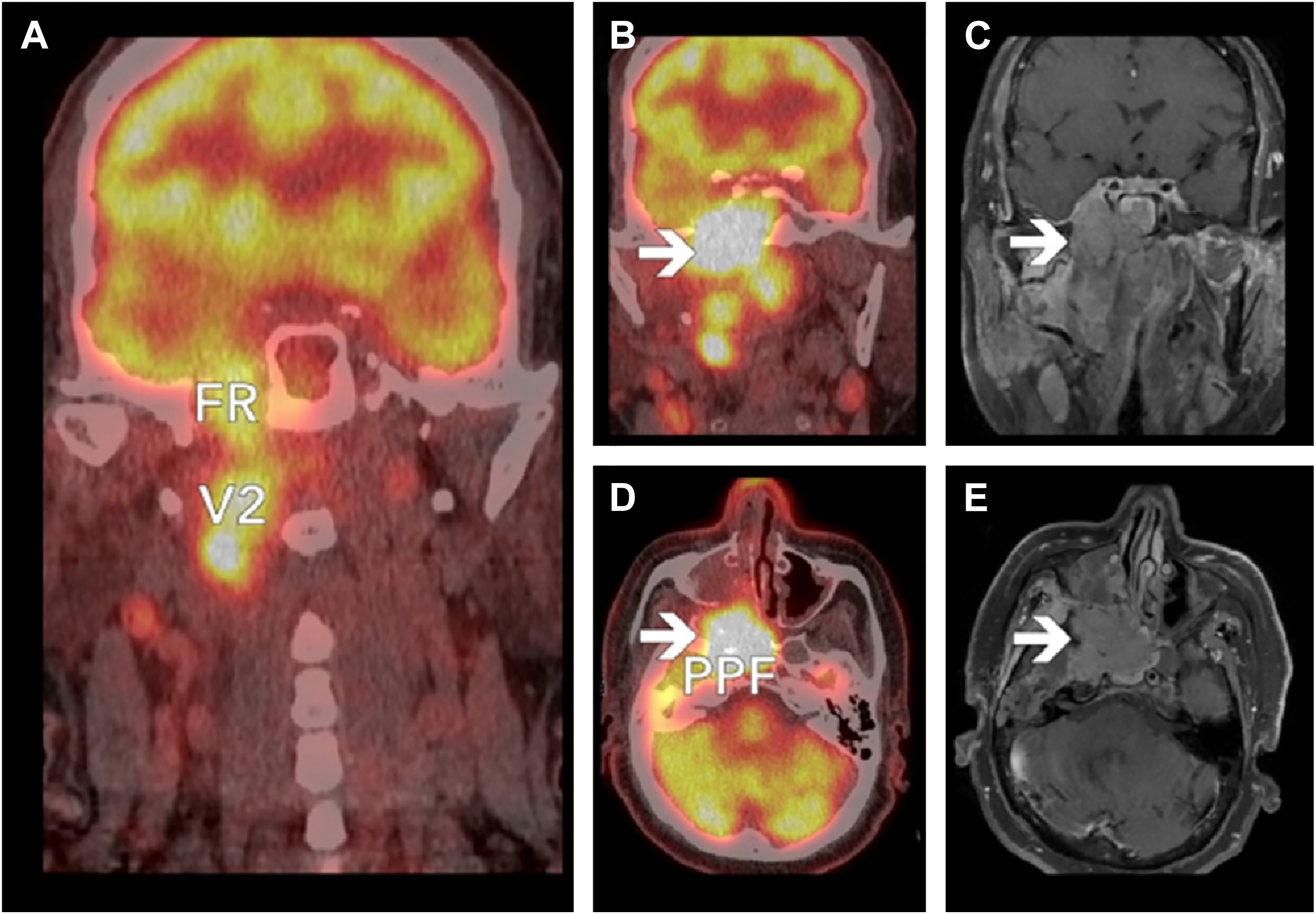
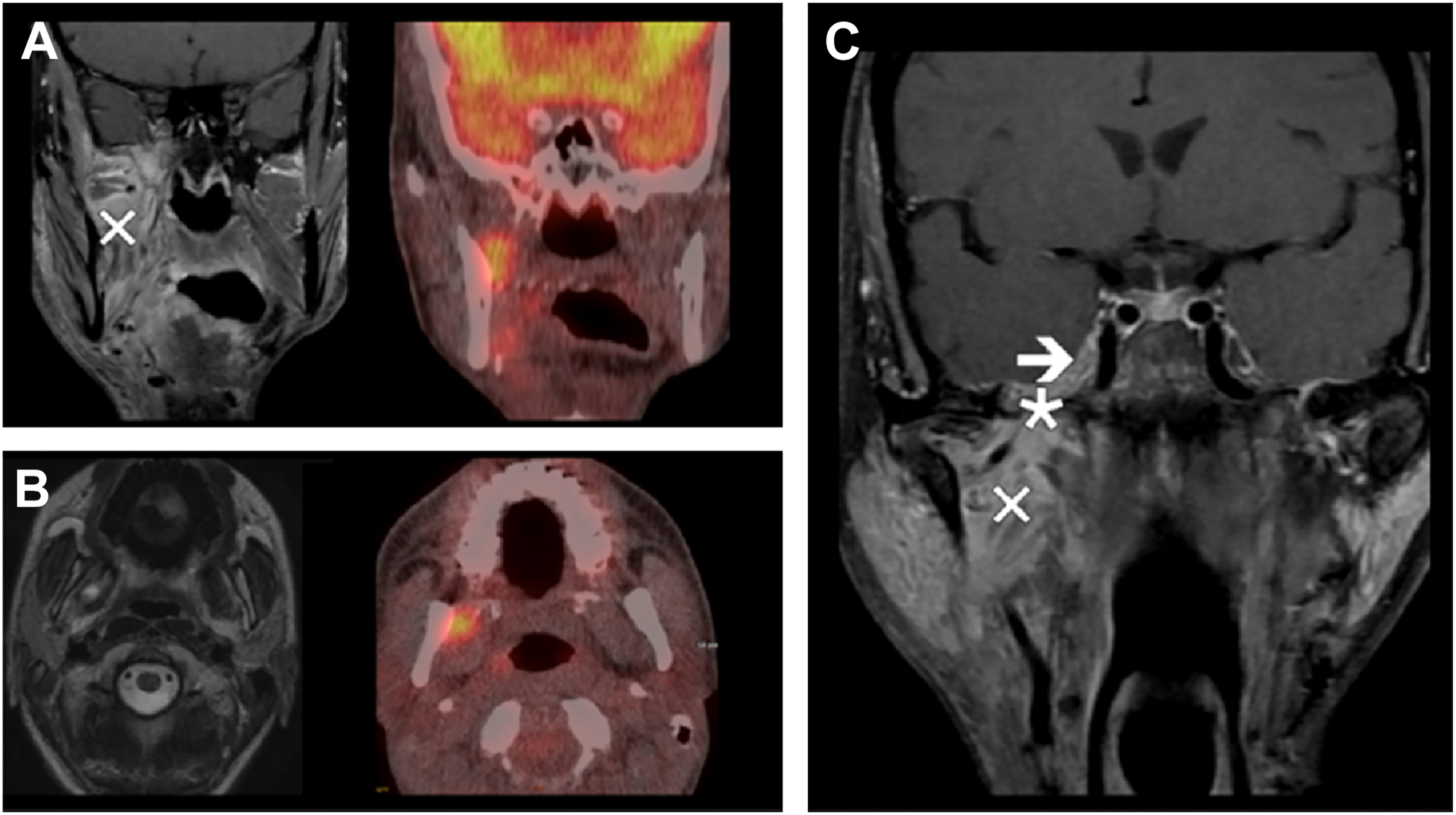
While pretreatment dental assessment is often solicited for HNC patients before they receive RT, they can still suffer from oral adverse effects. Chronic dry mouth, or xerostomia, and its resultant thick saliva, can result from RT in an irreversibly damaging process for salivary gland cells. Serous salivary glands, namely the parotid and submandibular, are particularly sensitive to radiation, and thus RT often affects the quantity and quality of saliva, altering its consistency from watery to viscous. Xerostomia can increase susceptibility to opportunistic infections, denture stomatitis, alteration in secretory immunoglobulin A, salivary stones and cysts, and burning mouth syndrome. It can also accelerate periodontal disease, and, most commonly, it can increase the occurrence or severity of dental caries. Factors enhancing the occurrence of caries after RT include the increase in the number of oral caries producing bacteria ( Streptococcus mutans and Lactobacillus species), reduced concentrations of antimicrobial proteins in the saliva, and loss of its mineralizing components.
Not only is salivary production impacted by RT, but adverse vascularity changes also present as a major issue following radiation for HNC. Carotid artery stenosis (CAS) can lead to reduced blood flow to the brain and, in doing so increase the risk of stroke and mortality. Two retrospective series comparing HNC patients to matched controls from population-based stroke registries found high correlations between RT and rates of stroke. Another severe complication of reduced vascularity is osteoradionecrosis (ORN), which involves bone death that is further worsened by reduced osteocyte population in the affected bone, most frequently the mandible. ORN is a lifelong risk for patients whose RT was high-dose, especially to the mandible, and tooth extractions and dental disease in irradiated areas further increase the susceptibility of developing and severity when having ORN.
Connective tissue changes can also develop as a result of RT. Fibrosis, or thickening of connective tissue, can develop in the skin in various RT-exposed sites, including subcutaneous tissues, muscles, or other organs. This complication typically begins 8 to 12 weeks after the initiation of RT, and it can easily develop into a lifelong decrement in patient quality of life and basic functioning. RT localized in the neck can cause woody texture of the connective tissue in a way that limits mobility, enhances lymphedema, and tightens muscles such as the scalenes, trapezius, and sternocleidomastoid muscles. Fibrosis can also occur in the pharynx and esophagus, leading to stricture and temporomandibular joint problems, including mandibular dysfunction.
The endocrine system is also susceptible to damaging complications from RT, primarily including both hypothyroidism and hyperparathyroidism. This may occur when the thyroid is not properly contoured or optimally avoided during the optimization process of RT, or in response to potential overdosing in the thyroid area due to the steep dose gradients between the HNC tumor and surrounding normal tissues. Immunologically, impaired leukocyte function has also been implicated as a factor in the pathophysiology of RT injury.
Discussion: vascular complications of radiation therapy in head and neck cancer
Vascular injury is a widely recognized complication of RT, as increases in vasculitis, atherosclerosis, and stroke following head and neck RT have been reported in several studies. , Complexity of head and neck vascular anatomy and the variability of HNC locations makes vascular avoidance during RT impractical. Vasculitis is marked by vessel wall inflammation, and the exact pathogenesis following RT remains unclear. It is recognized that ionizing radiation damages the endothelial cells, leading to permeability changes, inflammation, and infiltration of macrophages. Proinflammatory cytokines released by macrophages, including interleukin (IL)-1, IL-6, and tumor necrosis factor alpha, lead to oxidative stress. Low-density lipoprotein (LDL) molecules oxidized by free radicals are captured by macrophages and form lipid-laden macrophages (foam cells), eventually leading to atherosclerotic plaque depositions. In the evaluation of vascular complications, intima-media thickening is a key marker of postradiotherapy vascular changes.
Traditional anatomic imaging modalities such as computed tomography and MRI are insensitive to early molecular changes of vasculitis or atherosclerosis and provide limited value in early intervention in these complications. PET, however, is a versatile imaging modality that is characterized by the ability for molecular and functional assessment. In this section, we discuss the value of fluorodeoxyglucose (FDG)-PET and sodium fluoride (NaF)-PET in the assessment of vasculitis and atherosclerosis.
Vasculitis
While FDG-PET/computed tomography (CT) has long been used in oncology for diagnosis, staging, prognostication, and treatment response characterization, it has also been proven valuable in the study of inflammatory disorders by tracking glucose utilization in activated inflammatory cells. Several studies have applied FDG-PET/CT in the assessment of vasculitis following RT specific to HNC. In a study of 22 patients with HNC who underwent RT, Chen and colleagues obtained FDG-PET/CT scans before RT and 3 months after RT. They observed significantly higher mean and maximum target to background ratio and mean partial volume corrected standardized uptake value at 3 months after RT compared to baseline in the carotid arteries. Similarly, Borja and colleagues obtained FDG-PET/CT before RT and 3 months after RT in 30 HNC patients and observed significantly higher SUV mean in the left common carotid and arch of the aorta after RT.
These studies demonstrate the capability of FDG-PET/CT in quantitatively characterizing inflammation after RT in HNC patients. Pre-RT FDG-PET/CT and post-RT FDG-PET/CT is already commonly performed as clinical standard practice in HNC patients for cancer localization, staging, and therapy response monitoring. Therefore, further analysis of vascular inflammation with existing PET/CT studies can potentially be integrated into the standard of care without additional radiation burden for patients. Additionally, efforts are underway to investigate the differential vasculitis-inducing effects of photon and proton therapy using FDG-PET/CT. In a recent study of patients with non-small cell lung cancer, Evanson and colleagues observed significantly more RT-induced inflammation (measured by FDG-PET/CT) in the ascending aorta, descending aorta, and aortic arch of patients treated with photon RT compared to proton RT. Further studies with larger sample sizes, differing RT modalities specific to the application of HNC, differing radiation dose constraints, and considerations for different vascular disease risk factors are warranted to elucidate the pathogenesis of RT-induced vasculitis and guide clinical management.
Atherosclerosis
Accelerated atherosclerosis is a well-documented and feared complication of RT in HNC patients due to the affects that irradiation has on the carotid arteries and their related stroke risk. In a retrospective study, Arthur and colleagues examined the risk of ischemic stroke in 14,069 patients with squamous cell carcinoma of the head and neck treated with surgery and/or RT. Compared to surgical treatment, RT as standalone treatment was associated with a cause-specific hazard ratio of 1.70, and exposure to any RT in treatment regime posed a cause-specific ischemic stroke hazard ratio of 1.46. This result highlights the need for close monitoring of atherosclerosis progression following RT.
Due to the role of macrophage and inflammation in the pathogenesis of atherosclerotic plaques previously described, FDG uptake in atherosclerotic plaques has been documented in several studies. However, the use of FDG-PET in characterizing atherosclerosis is limited by several factors, including poor correlation with anatomic imaging-characterized structural changes, inconsistent correlation with macrophage marker CD86, and significant FDG uptake in background and surrounding tissues. NaF-PET has recently emerged as a promising alternative in the evaluation of atherosclerotic plaques. While FDG is taken up by metabolically active cells and, therefore, characterizes inflammation, NaF integrates into areas of microcalcification, therefore, providing an alternative means of assessing atherosclerotic plaques.
In a pioneering study by Castro and colleagues, NaF uptake in the left carotid artery was significantly correlated with the risk of cardiovascular events estimated by Framingham model, CHA2DS2-VASc score, and level of physical activity. Furthermore, Derlin and colleagues directly compared FDG-PET and NaF PET in a prospective study and discovered superior colocalization with arterial calcification was achieved by NaF (77.1%) compared to FDG (14.5%). These promising results warrant further prospective studies specifically in the context of RT-induced atherosclerosis and may provide insight into the progression of these posttherapy complications in HNC patients.
Discussion: central nervous system complications
In addition to the vascular complications associated with RT, there are several important central nervous system (CNS) complications to consider. The first issue deals with the propensity of HNC to spread to surrounding nerve tissues, a phenomenon referred to as perineural spread (PNS). This progression is associated with a poor prognosis and higher recurrence and lower survival rates for patients, and it is, therefore, crucial to be able to detect and intervene as early as possible in these scenarios. Typically, CT and MRI are used to monitor these patients. However, there is increasing interest and support for the use of FDG PET to diagnose PNS. One of the reasons for this is that RT may inhibit the ability to detect early PNS using MRI due to the increased inflammatory markers from treatment. For this, PET outperforms other imaging modalities. In a recent prospective study, FDG PET demonstrated a 100% detection rate in patients with PNS. This excellent negative predictive value demonstrates that FDG PET should be considered for the early detection of PNS. Through its superior detection, FDG PET may optimally aid in target delineation for RT target volumes, potentially improving outcomes for these patients.
Another CNS complication to consider in monitoring HNC post RT is in assessing for metastases. FDG PET has a well-documented role in finding metastases for a variety of cancers, and in HNC, metastases significantly impacts prognosis. In head and neck squamous cell carcinoma, for example, the spread to even just 1 lymph node is associated with a reduction in the survival rate by approximately 50%. While not the primary method for monitoring for spread to the lymph nodes, FDG PET has a high sensitivity and specificity for and has greater diagnostic capability than either CT or MRI alone, and it is less invasive than fine-needle aspiration. It is worth mentioning here that although it is not the focus of this article, FDG PET/CT is the preferred method for assessing for second primary tumors and distant metastasis to areas like the lungs, bone, and liver. Total body PET, therefore, is an optimal means to assess distant areas as well the more localized spread to the lymph nodes, and it can have valuable prognostic and therapeutic implications. Assessing for disease spread becomes even more challenging following RT. FDG is not only taken up by cancerous tissues, but by any highly metabolic tissues. This means that post RT there will be more FDG uptake in the surrounding tissues due to the inflammatory changes. Unfortunately, this limitation of PET can cause many false positives if the scan is performed soon after treatment. It is generally accepted that PET imaging will have difficulty distinguishing post-RT effects and inflammation from tumor until at least 8 to 12 weeks post RT. However, PET/CT does have a very high negative predictive value (>95%) in these cases, meaning if no uptake is visualized, no other interventions are necessary at that time. A PET NECK trial demonstrated this by comparing the FDG PET/CT surveillance with a cohort of patients who had a planned neck dissection at 12 weeks post treatment to monitor disease. FDG PET/CT performed equally as well as neck dissection in these cases and, therefore, should be preferable as it is more cost-effective and less invasive.
While RT can indirectly cause CNS complications in detecting PNS or metastases, one final complication can be the direct result of RT—that of radiation-induced necrosis (RN). To evaluate how PET might be useful in detecting this phenomenon, a background understanding of RN is helpful. The exact mechanism of RN is not well understood, though several hypotheses have been presented including direct radiation damage to the glial cells in the brain parenchyma, or the more commonly accepted theory that vascular damage to the surrounding tissue causes the necrosis. Factors that contribute to the development of RN include the radiation dose, size of the area irradiation, and use of concurrent or sequential chemotherapies and other systemic therapies. While RN is associated with treatment for brain lesions, it has been shown to be a complication of RT in HNC as well. Currently, a definitive diagnosis of RN is achieved by visualization of necrosis—usually coagulative and liquefactive—in the white matter either through surgical exploration or on histologic specimen after biopsy. The difficulty with this approach is that many of the lesions targeted by RT are themselves inoperable, making biopsy in these cases inherently risky, if possible at all. However, it is critical to be able to differentiate between disease progression following RT and the development of RN, as the 2 have vastly different therapeutic strategies. MRI is often not effective at making this distinction. PET, on the other hand, has shown promise in this area, although study is needed. There are varying levels of data on the use of PET for this purpose, as studies using FDG that show good sensitivity and specificity for detecting RT lack corresponding histologic verification, whereas other studies have not shown FDG to be an ideal tracer. However, several protein-based tracers have demonstrated the ability to identify RN using PET. Fluoride-labeled boronophenylalanine (F-BPA) and C-labeled methionine (C-MET) are 2 of the more successful tracers to date. One study using F-BPA was able to accurately distinguish tumor spread from RN by using a lesion/normal (L/N) ratio. While some overlap persisted, they found that a smaller L/N ration (<2.0) was associated with RN, and a higher ratio (>2.5) was indicative of disease spread. These studies admittedly have limitations, most notably of limited sample size based on disease rates and rates of RN, but the promise shown by PET in this critical area warrants further investigation and clinical use.
Summary
RT is a common and effective treatment for head and neck cancer but it is also associated with significant acute and chronic complications. These adverse effects are closely tied to the duration and dosage of RT. Acute side effects, such as inflammation and mucositis, can often be managed by adjusting the treatment schedule, but chronic complications are more difficult to control, as they stem from cumulative radiation damage. The most widely accepted theory explaining the long-term effects of RT involves increased fibrosis and reduced vascularity, leading to tissue hypoxia, necrosis, and a heightened risk of infection. These complications often manifest long after treatment, affecting salivary glands, connective tissues, vascular systems, and endocrine or immune functions. These chronic issues represent lifelong challenges for patients, requiring ongoing care to manage symptoms and maintain quality of life.
One long-term effect of RT in HNC patients is vascular injury, which can lead to vasculitis, atherosclerosis, and increased risk of stroke. The complexity of the head and neck anatomy and the tumor’s proximity to key vascular structures makes it difficult to avoid collateral damage during RT. Ionizing radiation damages endothelial cells, triggering inflammation, oxidative stress, and the formation of atherosclerotic plaques. FDG-PET imaging has emerged as a valuable tool for monitoring vascular inflammation and atherosclerosis after RT. Studies have demonstrated its ability to track glucose uptake in inflamed tissues, providing a noninvasive method to detect vasculitis early. Additionally, the use of NaF-PET offers promising insights into atherosclerotic plaque formation by identifying areas of microcalcification, a key marker of cardiovascular risk. Early detection and monitoring are critical in preventing severe outcomes such as strokes in these patients.
Central nervous system (CNS) complications from RT, such as PNS and radiation necrosis, are also significant concerns in HNC patients. PNS, where cancer spreads along nerve pathways, is associated with a poor prognosis. Early detection and optimal RT target volume delineation are crucial for improving outcomes, and FDG-PET has shown excellent sensitivity in identifying PNS, outperforming MRI, which can struggle to detect these changes due to inflammation caused by radiation. FDG-PET is also valuable in differentiating between radiation necrosis and tumor recurrence, a critical distinction that influences treatment decisions. Although the precise mechanisms of radiation necrosis are still debated, PET imaging has shown promise in identifying necrotic tissue, aiding in early diagnosis and management.
In conclusion, while radiation therapy is an essential component of HNC treatment, its long-term effects on vascular, connective, and nervous tissues demand careful monitoring. Imaging techniques like FDG-PET and NaF-PET play a pivotal role in detecting and managing radiation-induced complications, helping clinicians make informed decisions about patient care.
Clinics care points
- •
Long-term Surveillance and Follow-up for Vascular Complications: Radiation-induced vascular complications, such as CAS and atherosclerosis, may emerge years after treatment. Long-term follow-up is essential, especially for patients who received high-dose radiation or have traditional cardiovascular risk factors. Regular cardiovascular assessments, including carotid ultrasound, FDG-PET, or NaF-PET, should be part of ongoing surveillance to monitor for inflammation and plaque development. Monitoring should continue for decades post therapy, with a focus on stroke prevention. Early interventions, such as lifestyle modifications and the use of statins or antiplatelet agents should be considered based on imaging results.
- •
Early Detection of Osteoradionecrosis and Preventive Care: Patients who undergo RT to the head and neck are at lifelong risk of osteoradionecrosis, particularly in the mandible. Preventive care should focus on minimizing trauma to irradiated bone, and regular dental assessments are critical. Avoid invasive dental procedures when possible, and if necessary, consider prophylactic measures such as hyperbaric oxygen therapy or antibiotics. Close monitoring for early signs of bone necrosis should lead to prompt intervention to prevent the progression of ORN.
- •
Management of Xerostomia and Dental Health Post-RT: Xerostomia, or dry mouth, is a common long-term effect of RT and significantly affects oral health. Regular dental evaluations should be conducted to monitor for dental caries, periodontal disease, and oral infections. Preventive care, including fluoride treatments, saliva substitutes, and antimicrobial rinses, should be initiated early to reduce oral health risks. Saliva stimulants such as pilocarpine may also be used to increase salivary flow, and dietary counseling should be provided to help mitigate the impact on oral health.
- •
Advanced Imaging for Early Detection of PNS and CNS Complications: Central nervous system complications, including PNS and radiation necrosis, can occur long after RT. Advanced imaging, such as FDG-PET, is highly effective in detecting PNS and differentiating between radiation necrosis and tumor recurrence. Regular imaging follow-ups should be considered, particularly for patients at high risk for CNS involvement. Early detection allows for timely interventions, potentially including surgery or adjustments to radiation treatment plans.
- •
Fibrosis Management and Quality of Life Improvement: Radiation-induced fibrosis can severely impact connective tissue function, particularly in the neck and jaw. Early physical therapy is essential to preserve mobility and prevent fibrosis from worsening. Regular assessments of neck mobility and swallowing function are necessary to detect fibrosis-related complications. In severe cases, swallowing therapy or surgical intervention may be required to improve function and overall quality of life.
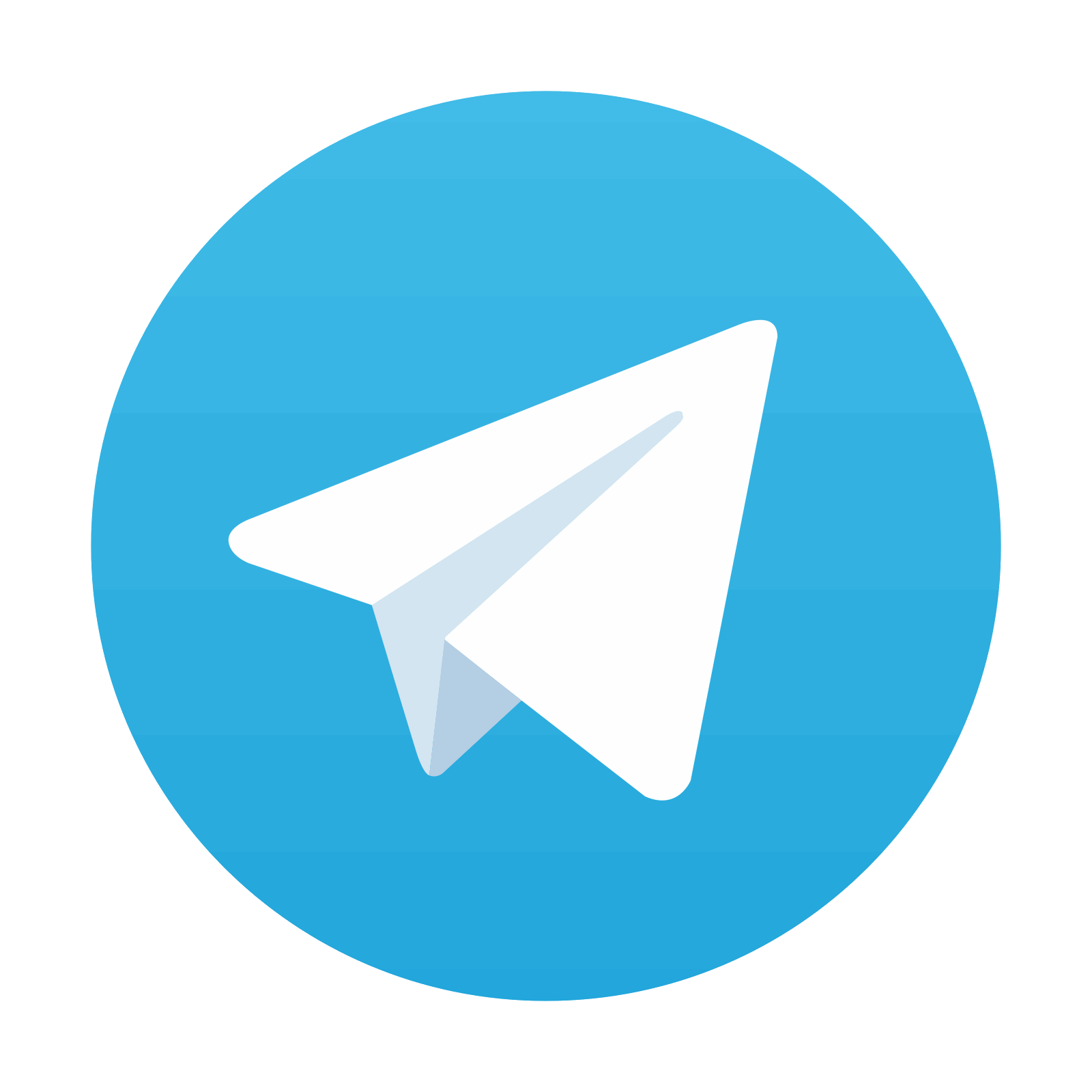
Stay updated, free articles. Join our Telegram channel

Full access? Get Clinical Tree
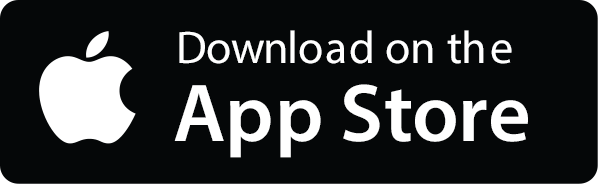
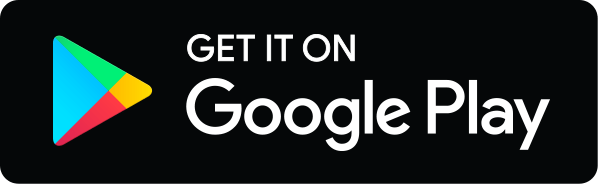
