Abstract
Objective
Current diagnostic methods of inflammatory periodontal diseases, e.g. , visual evaluation, periodontal probing, and radiographs, are either subjective or insensitive. Intra-oral high-frequency ultrasound was investigated to quantify inflammation by detecting tissue dimensional and perfusion changes.
Methods
A cohort of 15-month-old mini-pigs, 4 female/male each, was analyzed. Pre-molars (PM) 3 and 4, as well as first molars (M1), were scanned. In bi-weekly time intervals all 4 quadrants were randomly enrolled and bacterial injection followed each quadrant scan in a weekly fashion. Soft tissue dimensions were obtained from B-mode images and statistically analyzed to identify correlations to inoculation time, i.e. , response to bacterial loading, tooth type and sex, using analysis of variance and regression analysis. Color flow velocity and power-weighted color pixel density was obtained and statistically analyzed analogous to soft tissue.
Results
Soft tissue thickness increased significantly post-inoculation at 1 and 2 mm below the free gingival margin for both genders and all observed teeth. The significance lasted for weeks 2, 4 and 6, except for female M1s (4 weeks). Color flow velocity was significantly higher compared with baseline for 6 weeks, except for male PM4 (2 weeks). Color flow power did not show significance for PM3 and 4, only in M1 (except male week 4). Significance also extended to tooth type and sex.
Conclusion
Periodontal tissue dimension and color flow velocity increased in correlation to bacterial inoculation. Further studies are needed to obtain an understanding of the underlying biology observed here. Eruption of dentition may have been a confounding factor for inflammation interpretation.
Introduction
Periodontitis is a multifactorial chronic inflammatory disease characterized by progressive destruction of the periodontium and negatively impacting 42.2% of the US adult population [ ]. This process is triggered by an imbalance between subgingival microbiota and the inflammatory response of the host. The literature suggests that severe periodontitis is the sixth most prevalent condition in the world [ ]. Age-related prevalence shows a steep increase between the third and fourth decades of life, with peak incidence at around 38 years of age [ ]. Prevalence and severity of the disease increases with increasing age and tooth loss [ ]. Risk factors include smoking, diabetes mellitus [ , ], cardiovascular disease, obesity, and others [ ]. Patients with diabetes mellitus are associated with increasing clinical attachment loss [ ] and reduced response to periodontal therapy [ ].
The junctional epithelium acts as a mechanical barrier as well as a physiologic reactor to maintain homeostasis of normal flora. The rapid turnover rate of the epithelium leads to shedding of the mucosal surface exposed to bacterial colonization, which limits bacterial invasion. Once this balance is disrupted, the innate immune defense is activated by increasing vascular permeability to allow influx of neutrophil and macrophage cells. Vasculitis and acute inflammatory response lead to increased blood flow, dilated and engorged blood vessels, and exudate in the gingiva, which appears erythematous, edematous and rolled at its margin. The gingiva will start to lose its collagen content due to increased proteinase activity [ ]. This local immune response, if not able to contain the bacterial insult, will shift to a more chronic and destructive immune response, leading to accumulation of inflammatory mediators, e.g. , interleukin-1, tumor necrosis factor-α and prostaglandins, and resulting in progressive destruction of the periodontium [ ]. Clinical signs will appear as periodontal attachment loss, recession, bone loss, mobility and ultimately tooth loss.
In periodontology, diseases are defined clinically by measures of probe depth, attachment loss, bleeding on probing, etc ., which are limited in constituting an objective path to segregate subjects into diseased cases versus healthy subjects [ ]. Radiographs have been used to evaluate the amount of alveolar bone loss but the current clinical diagnostic standards are primitive, subjective and insensitive, making it very difficult to diagnose disease in the initial stages. Quantifying periodontal disease in a meaningful and reproducible manner has been an ongoing challenge for oral epidemiologists and clinicians [ ]. Currently the standard of care for diagnosis of periodontal disease is X-rays and the percentage bone loss by age helps to determine the rate of disease progression [ ]. The literature suggests that radiographic assessments underestimate bone loss compared with surgical measurements by 1.4 ± 2.6 mm [ ]. Early diagnosis of periodontal inflammation and tissue dimensional changes could result in preservation of periodontal tissue and a positive treatment outcome. Non-invasive and point-of-care ultrasound that would quantify inflammation by detecting sub-clinical vascular changes would be beneficial for early diagnosis of periodontal disease and as a diagnostic tool to assess and monitor response to therapy. The cascade of pathophysiological changes in periodontal disease that leads the variation in vascular changes can also be longitudinally monitored, and preliminary clinical results of our group have already shown clinical feasibility [ ]. Other researchers have extensively investigated the applications of ultrasound in the field of dentistry, which has been used for a wide range of applications including measuring periodontal pocket, enamel and dentin thickness; caries and crack detection in enamel; imaging of different layers of tooth and the supporting structures, including the pulp, root surface, alveolar bone, gingival tissue measurements; detection of soft tissue lesions with bony involvement; detection of hard tissue junction (dentino-enamel junction); and measurement of gingival layers (rete-pegs) [ ].
For a long time, sonography was out of the realm of being a radiologic option and two main limitations prevented its use as a periodontal imaging modality that otherwise would be excellent with respect to soft tissue contrast. First was achievable image resolution. Sonography was too coarse with respect to the often thin (1–2 mm) periodontal tissues. This is especially true for pathologic cases, i.e. , where imaging might help the provider to inform their therapy options or assess and monitor response to therapy for already thin tissues. Current clinical ultrasound transducers are available with a 33 MHz imaging frequency [ ] and pre-clinical systems achieve >70 MHz [ ]. At these high frequencies, the expected pixel size would be 46.7 and 22 for 33 and 70 MHz, respectively, assuming a sound of speed of 1540 m/s. A 1 mm-thick tissue would be resolved with up to 45 image pixels in the axial direction. Using an f-number of 3, an up to 66-μm lateral resolution would be feasible. For 1-D arrays, the elevational slice thickness would depend on the lensing added to the acoustic stack and matching layer. A second roadblock for periodontal ultrasonography was the physical size of the available transducers. General transcutaneous scanning for peripheral vasculature, obstetrics, cardiac tissues or general abdominals did not pose strong size limitations on its dedicated transducers except for ergonomic reasons [ ]. Endocavitary imaging, however, led to the development of new geometries suited for this more demanding application, but this was exclusively limited to transvaginal and transrectal imaging due to identified medical needs. Oral scanning, i.e. , another endocavitary application that requires its own specific geometry and design, had been lacking attention. A clinically practical transducer needs to physically fit into the oral cavity and offer enough space to maneuver for reaching any clinically driven position and orientation. Concurrently, it needs to also offer a competitive image resolution. Here we are using a relatively new transducer that was designed by principal investigators and the industry to address this void.
The use of innovative imaging technologies in periodontics such as ultrasound could be beneficial to quantifying inflammation by detecting sub-clinical vascular and other soft tissue changes, and thus potentially aiding the early diagnosis of periodontal disease and therefore preventing disease progression. Clinical applications have recently been reviewed by several groups [ ]. Studies have used color flow and pulsed wave ultrasound to detect blood vessels such as the greater palatine artery in the oral cavity to aid in better surgical treatment planning [ ]. This technology has also proven to be efficient in comparing the effect of age, sex and blood pressure on blood flow velocity in dental pulp [ ]. Longitudinal monitoring is also attractive, especially when using Doppler ultrasound, due to its non-invasive and non-ionizing nature, and its ability to produce high soft tissue contrast. In addition, improvement of periodontal health is known to also have a positive impact on systemic health [ ]. The aim of this study was to monitor periodontal gingival blood flow and tissue dimensions assessed by ultrasound in a pre-clinical model during induced periodontal inflammation.
Materials and methods
Pre-clinical model
This study was conducted at the University of Michigan, Michigan Medicine (Ann Arbor, MI, USA) according to protocol #PRO00010333 and approved by the Institutional Animal Care & Use Committee. It was a longitudinal bacteria-induced periodontitis study in a porcine model. Eight Sinclair mini-pigs (4 females and 4 males, 15 months of age) were obtained from Sinclair Bio Resources (Auxvasse, MO, USA). A porcine model was used as the inflammatory process is similar to that seen in human periodontal diseases [ ].
All experiments were conducted under general anesthesia and all animals were fasted the night before intramuscular anesthesia induction with telazol and tylazine in the housing area prior to weighing and transporting to the lab. The animals were also injected intramuscularly with glycopyrrolate in the housing area and intubated prior to being placed on 3% isoflurane for the duration of the procedure. A face mask was used with isoflurane to aid intubation. The animals were placed onto an approved external heat source on the procedure table and instrumented for continuous monitoring (heart rate, rectal temperature, SpO 2 levels, respiration rate) throughout the procedure. Intravenous fluids were administered (0.9% NaCl, 5–10 mL/kg/h) for the duration of anesthesia through an intravenous catheter placed in the ear vein. The animals were anesthetized for a maximum of 12 times (once for initial cleaning, once for initial imaging and ligature placement, and up to 10 times for follow-up imaging). If a procedure took a short amount of time, i.e. , only inoculations, atipamezole was administered at 0.1–1.0 mg/kg for the reversal of xylazine.
To establish a baseline with minimal dental plaque, oral prophylaxis/tooth cleaning was performed for all quadrants of teeth 1 week before (week -1) the start of the actual ultrasound scanning (described in the next section). One week was added after oral prophylaxis to give time to the soft tissue to equilibrate. Baseline ultrasound scans were performed at week 0. Longitudinal ultrasound scans were performed bi-weekly ( Table 1 ). At baseline one randomized quadrant was scanned. Every 2 weeks a new randomized quadrant was added and scanned (baseline) before being inoculated with bacteria. All scans were followed by inoculations except for the final scan. Once added to the study, the quadrants were inoculated every week.
Relative biological age (weeks) | 0 | 1 | 2 | 3 | 4 | 5 | 6 | 7 | 8 | 9 | 10 |
---|---|---|---|---|---|---|---|---|---|---|---|
Quadrant A no. scans: 6 | Base Inoc | Inoc | Scan Inoc | Inoc | Scan Inoc | Inoc | Scan Inoc | Inoc | Scan Inoc | Inoc | Scan |
Inoculation age | 0 | 1 | 2 | 3 | 4 | 5 | 6 | 7 | 8 | 9 | 10 |
Quadrant B no. scans: 5 | Base Inoc | Inoc | Scan Inoc | Inoc | Scan Inoc | Inoc | Scan Inoc | Inoc | Scan | ||
Inoculation age | 0 | 1 | 2 | 3 | 4 | 5 | 6 | 7 | 8 | ||
Quadrant C no. scans: 4 | Base Inoc | Inoc | Scan Inoc | Inoc | Scan Inoc | Inoc | Scan | ||||
Inoculation age | 0 | 1 | 2 | 3 | 4 | 5 | 6 | ||||
Quadrant D no. scans: 3 | Base Inoc | Inoc | Scan Inoc | Inoc | Scan | ||||||
Inoculation age | 0 | 1 | 2 | 3 | 4 |
A small notch (2 mm long, 0.5 mm wide and 0.5 mm deep) was placed using a high-speed handpiece on the midfacial location of the crowns of pre-molars 3 and 4, as well as the first molars, to provide a landmark for reproducible scan. After baseline imaging, a silk ligature was placed sub-gingivally as a standard procedure to induce inflammation by increasing plaque accumulation [ , ]. Additional periodontitis-inducing bacteria ( Porphyromonas gingivalis, Fusobacterium nucleatum and Treponema denticola ) [ , ] were inoculated into the sulcus at the rate of 5 µL/min to accelerate the disease process [ ]. Another quadrant was added to the experiments every 2 weeks and the same sequence of procedures were followed ( Table 1 ). The pigs were scanned up to 10 weeks from baseline bi-weekly with an additional quadrant every other week and were euthanized according to protocol guidelines after 10 weeks.
Ultrasonography
A US FDA-approved clinical ultrasound system (ZS3, Mindray Innovation Center NA, San Jose, CA, USA) was used for this study, together with a small-footprint 128-element linear array practical for intra-oral imaging (L30-8, Mindray Innovation Center NA), shown in Figure 1 . The transducer has an 18-MHz center frequency and was operated in CSH24 mode, which enables compounding as well as harmonic imaging (12–24 MHz). By using the virtual convex mode, a lateral field of view of 12.8–18.9 mm was achieved at a depth of 0 and 15 mm, respectively. The ultrasound probe was prepared with a 5 mm standoff gel pad to place the soft tissue into the elevational focus of 8 mm without the need for a large amount of ultrasound gel [ ]. The latter was used not only for coupling of the acoustic field into the soft tissue, but also to minimize the amount of mechanical pressure on the periodontal tissues. For that we maintained a small distance between the standoff pad of the transducer and the soft tissue. This helped to prevent any additional pressure that could distort or displace the soft tissue. While ultrasonography cannot per se image tissue perfusion, color flow was acquired to function as a surrogate marker. Care was taken to hold the transducer steady as a color flow frequency of 15 MHz was used with a wall filter setting of low. At 15 MHz the nominal wavelength is 102.7 µm and, consequently, assuming a 3% wall filter, 1.54 µm phase sensitivity was achieved. While it is assumed that the system has sophisticated algorithms to filter clutter, this estimate illustrates the potential sensitivity to blood flow. Still images and cine-loop data were exported as DICOM files for offline processing.
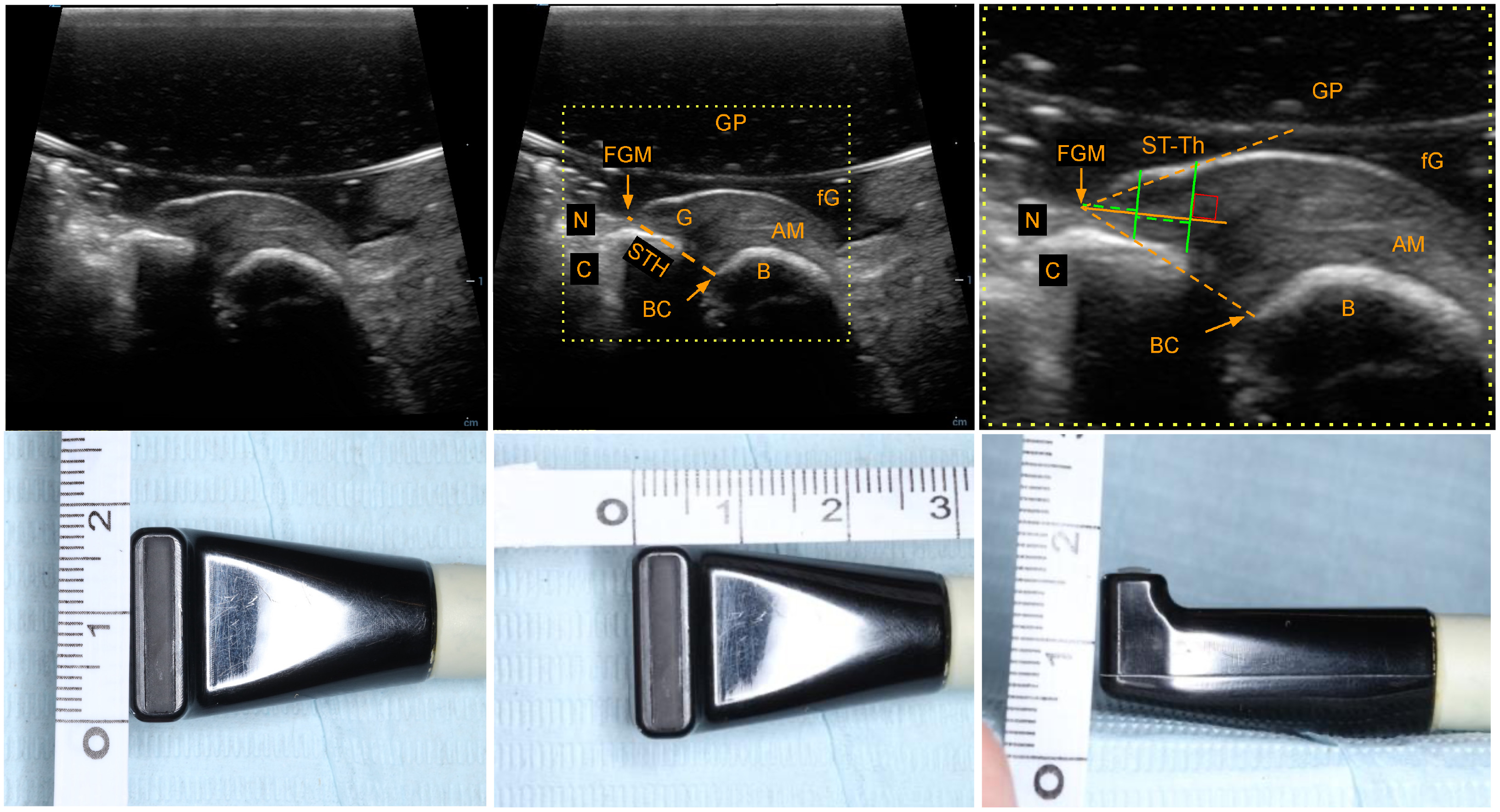
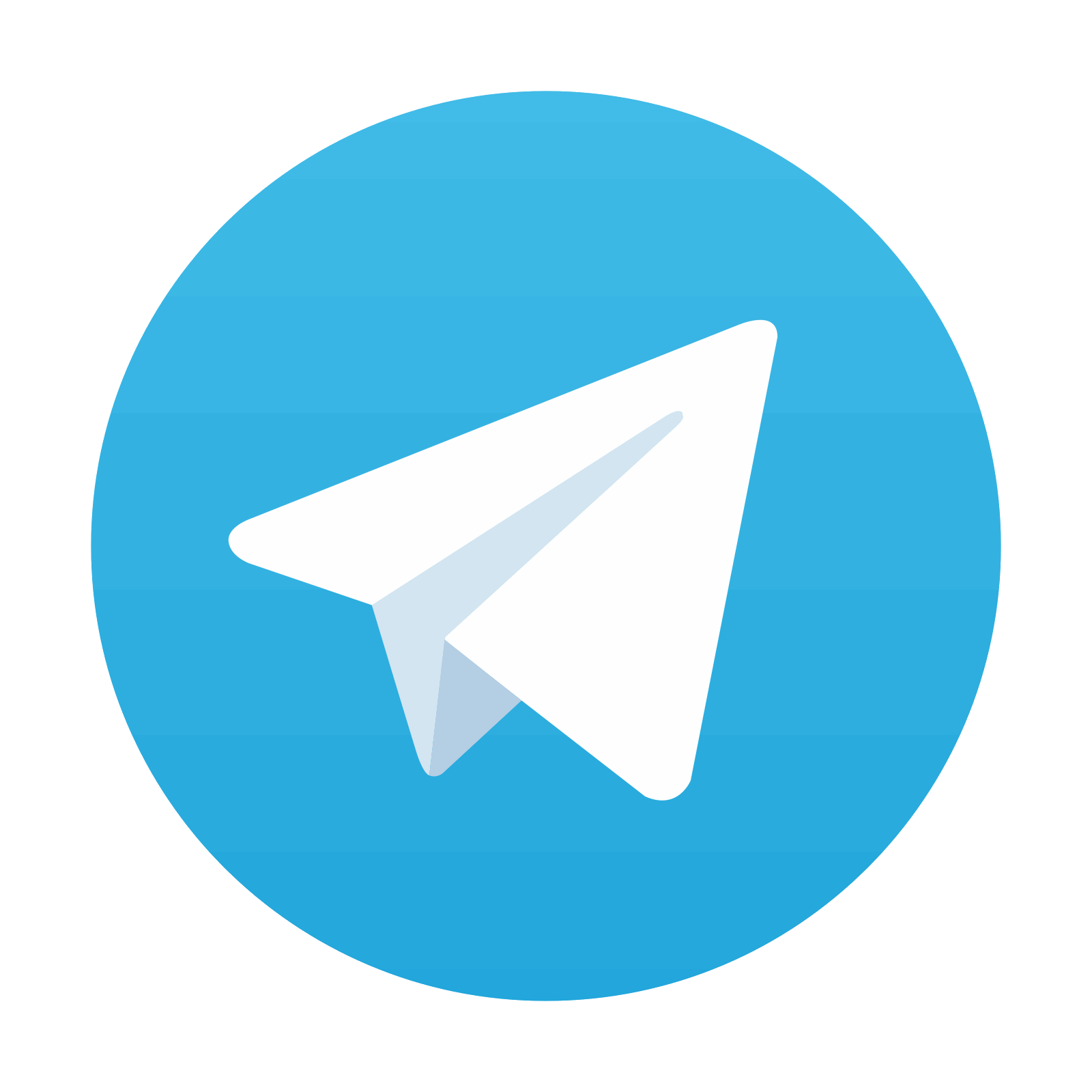
Stay updated, free articles. Join our Telegram channel

Full access? Get Clinical Tree
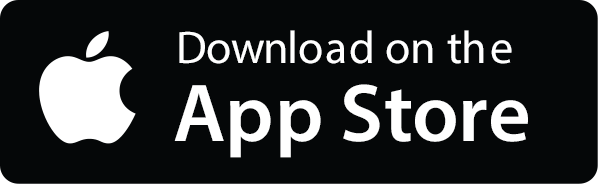
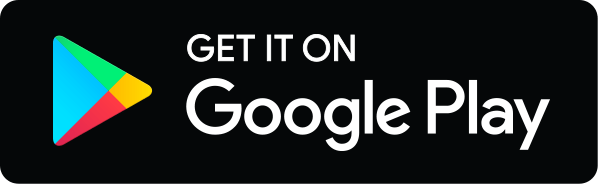
