Abstract
Objective
Perfluoropropane droplets (PD) are nanometer-sized particles that can be formulated from commercially available contrast agents. The preferential retention of PDs in diseased microvascular beds can be detected by ultrasound imaging techniques after acoustic activation and offers an opportunity for the detection of such processes as scar formation or inflammation. We hypothesized that in the presence of ischemia/reperfusion (I/R) injury, retention of intravenously injected PDs would be enhanced.
Methods
Using an established intravital microscopy model of rat cremaster microcirculation, we determined the retention and subsequent acoustic activation behavior of PDs in exteriorized rat cremaster tissue. DiI-labeled droplets (200 µL) were administered intravenously. Acoustic activation was achieved with a clinical ultrasound system at two ultrasound frequencies (1.5 and 7 MHz).
Results
Fluorescent microbubbles could be detected in the microvasculature after intravenous injection of PDs and subsequent acoustic activation. Increased retention of PDs was observed in the I/R group compared with control group with both ultrasound frequencies ( p < 0.05). Using higher-resolution microscopy, we found evidence that some droplets extravasate to the outside of the endothelial border or are potentially engulfed by leukocytes.
Conclusion
Our data indicate that targeted imaging of the developing scar zones might be possible with ultrasound activation of intravenously injected PDs, and a method of targeting therapies to these same regions could be developed.
Introduction
Over the past few decades, microbubbles have been extensively used for contrast enhancement in diagnostic ultrasound imaging across various clinical applications, including tumor detection and enhanced endocardial wall definition in echocardiographic imaging [ , ]. In response to ultrasound, microbubbles either oscillate (stable cavitation) or collapse (inertial cavitation) depending on the applied acoustic pressure [ ]. These oscillations in the capillaries can cause microvascular leakage and can be leveraged for blood-brain barrier disruption [ , ]. Additionally, commercially available microbubbles can be compressed into nanodroplets, which exhibit unique acoustic responses and offer enhanced diagnostic potential compared to microbubbles [ ].
The physical interaction between acoustic pressures and phase-change contrast agents alters the local permeability of cell membranes and microvasculature, enabling applications such as drug delivery, targeted vessel occlusion and enhanced cavitation activities [ ]. For the purposes of this study, perfluoropropane droplets ( PD ) are nanometer-sized particles with a liquid-phase metastable perfluoropropane core encapsulated within a monolayer of lipids. Their unique properties significantly enhance their cardiovascular diagnostic and therapeutic potential [ , ]. When subjected to acoustic pressure beyond a certain threshold, these droplets vaporize, causing the liquid core to transition to gas [ ]. PDs demonstrate improved stability in blood circulation and can extravasate into tissues due to their nanometer size [ ]. As a cavitation agent, PDs offer therapeutic potential, drug-loading capacity and high-specificity localized drug delivery [ ]. Intravenous injection of microbubbles or PDs is generally safe with minimal known side effects, as the perfluorocarbon gas is rapidly exhaled from the body [ , ].
Ischemia/reperfusion ( I/R ) injury triggers oxidative stress and inflammation [ , ]. In animal models of coronary ischemia and reperfusion, the acute inflammatory response is most marked at 24 h post-reperfusion followed by myofibroblast transformation, collagen formation and chronic inflammatory cells that are predominant at 7 d post-reperfusion. Inflammation is an orchestrator of scar formation, mainly by the stimulation of fibroblasts by inflammatory cells, which in turn drives the production of scar tissue [ ]. At each of these time periods following I/R, there is evidence of droplet retention resulting in acoustic activation of the developing scar zone [ ]. The predominant location of the retained droplets has not been elucidated.
Inflammation is associated with microvascular retention of targeted microbubbles [ ]. Targeted microbubbles allow selective accumulation and retention of ultrasound contrast agents in ischemic or inflamed areas due to their specific affinity for endothelial biomarkers such as p-selectin, von Willebrand’s factor and integrins [ ]. These findings have led to the preclinical use of targeted microbubbles for the early detection of myocardial reperfusion injury [ , ].
Over 20 y ago, Fisher et al. [ ] discovered that non-targeted microbubbles with a negative charge could be retained within capillaries through complement-mediated attachment to the endothelium. The degree of retention correlates with the extent of ischemia or inflammation in myocardial or kidney reperfusion injury [ , ]. The preferential retention of PDs in diseased microvascular beds can be detected by ultrasound imaging post-acoustic activation, providing an opportunity to detect processes such as scar formation. PDs after acoustic activation produce significant enhancement with harmonic and multi-pulse fundamental non-linear imaging sequences, allowing the imaging of accumulated droplets within the infarct zone several minutes after intravenous administration of PDs [ ]. In this study, we use non-targeted PD, which can be manufactured from commercially available microbubbles, to explore wider applications. Using a rat cremaster muscle model to simulate a microvascular environment, we aimed to determine the effect of high mechanical index (MI) activation/cavitation impulses on droplets within a normal microvascular flow setting. We hypothesized that I/R would enhance the retention of intravenously injected PDs. Additionally, we also aimed to identify the spatial location of the retained droplets and microbubbles post-vaporization using high-resolution intravital microscopy.
Materials
Preparation of PDs
Perfluoropropane microbubbles (PMB ) were formulated by the dissolution of 1,2-dipalmitoyl-sn-glycero-3-phosphatidylcholine (DPPC), 1,2-dipalmitoyl-sn-glycero-3-hosphatidylethanolaminepolyethyleneglycol-5000 (DPPE-PEG-5000) and 1,2-dipalmitoyl-sn-glycerol-3-phosphatidic acid (DPPA) in a molar ratio of 82:10:8 in an excipient liquid comprised of propylene glycol, glycerol and normal saline (1:1:8, v:v:v). After adding 1.5 mL of the resulting solution to a 2 mL vial, the solution was degassed on a vacuum manifold, followed by purging with perfluoropropane (Fluoromed, Round Rock, TX). PMBs were formed by shaking the vial on a modified dental amalgamator for 45 s. The PMBs were diluted in normal saline (1:1 v:v) and loaded into a 10 mL syringe and cooled in an isopropyl alcohol bath (–10°C) for 3 min. The cooled PMB suspension was then pressurized by clamping the syringe with a rubber stopper in a clamp which applied a controlled pressure for 3 min [ ]. The condensed PMBs go through phase change and become PDs. The size and the zeta potential of PDs were measured with a Nano Zetasizer (Malvern Nano ZS, Malvern Instruments Ltd., Malvern, Worcestershire, UK) and the concentration was determined with Nanosight laser scattering analysis (Nanosight NS 300, Malvern Instruments Ltd.). PMBs were fluorescently labeled with a commercially available lipophilic fluorophore, 1,1′dioctyldecyl 3,3,3′,3′tetramethylindocarbocyanine ( DiI ) [ ] for fluorescence imaging, with an emission spectrum at 565 nm. Fluorescent labeling was achieved by adding DiI to the PD microbubble preparation prior to the droplet formation method described above, based upon PDs used in studies by Marshalek et al. [ ]. The droplets were then diluted in saline with 1:1 prior to use.
Animal preparations
All protocols were approved by the Institutional Animal Care and Use Committee at the University of Pittsburgh. The rat cremaster muscle was exteriorized through a scrotal incision and secured to a translucent pedestal [ ]. The blood flow to the cremaster tissue was interrupted for 10 min with a ligature around the cremasteric artery proximal to the muscle body to mimic ischemia, followed by a 20-min reperfusion period to cause reperfusion injury. A 200 μL intravenous injection of DiI-labeled droplets (DiI-PD) at 4.05×10 10 droplets/mL concentration was administered following I/R. At 2 min post-injection, the cremaster muscle was treated with a clinical ultrasound system (Siemens Sequoa 512, Siemens Healthcare, Erlangen, Germany) in Contrast Pulse Sequences mode at 1.5 MHz (with the 3V2c probe) or 7 MHz (with the 15L7 probe) for 10 frames over 2 s at a MI of 1.1 ( Fig. 1 ). Control animals received identical PD injection and ultrasound treatment, but did not undergo I/R.
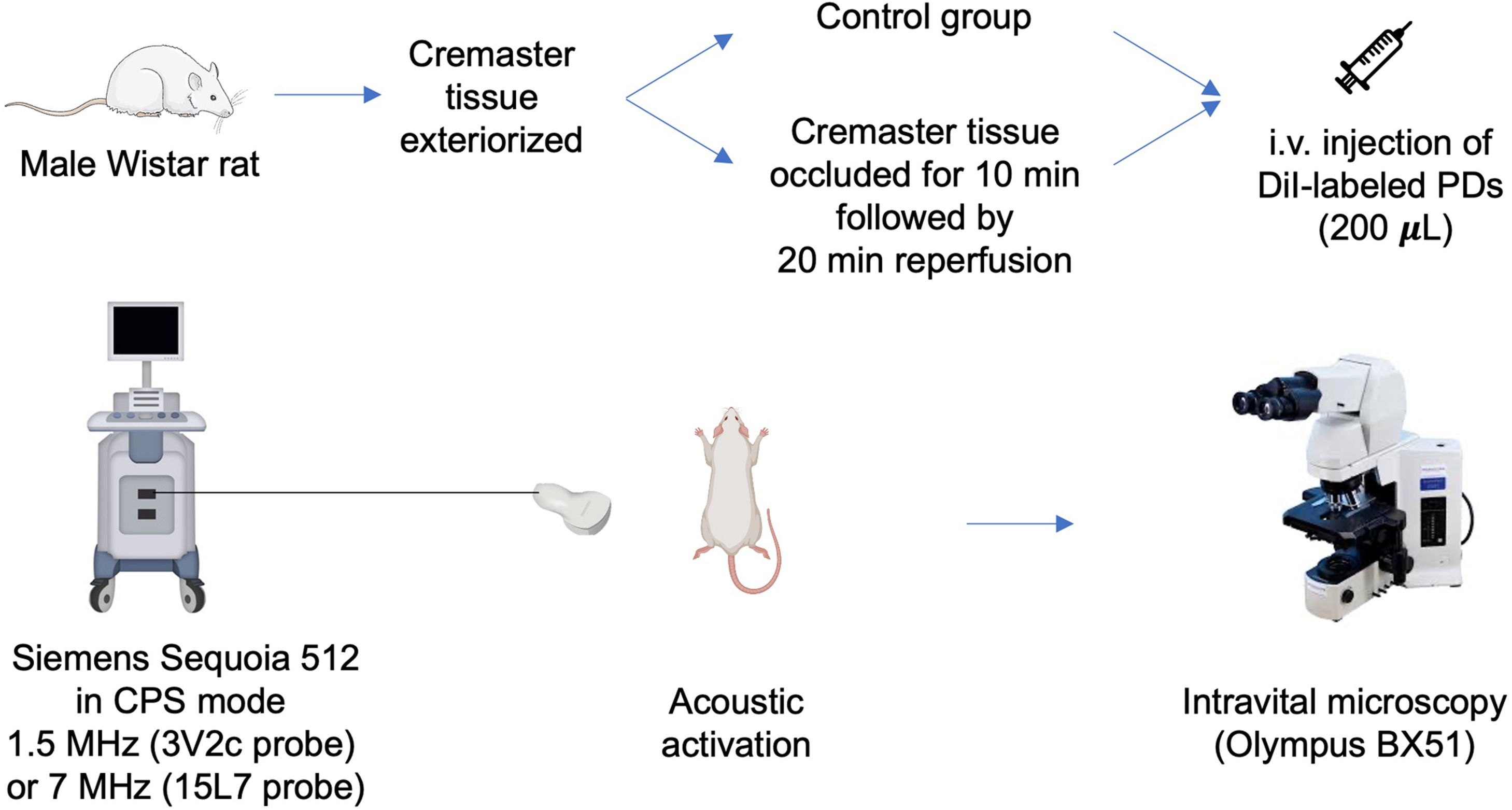
Intravital microscopy and imaging analysis
All the rats were randomized into four groups: low-frequency control ( n = 8), high-frequency control ( n = 7), low-frequency I/R ( n = 8) and high-frequency I/R ( n = 7). Intravital microscopy (Olympus BX51, Olympus America, Inc., Center Valley, PA) was performed on the exposed rat cremaster muscle for real-time visualization of droplet retention in vivo . For each animal, 10 random files of brightfield and fluorescence images were captured using a 20× lens. Images were analyzed using MATLAB (MathWorks, Natick, MA) and ImageJ. The histogram of each fluorescence image was obtained. A threshold value was determined from the averaged histograms of background images such that 99.9% of the pixels were below this threshold. All the pixels above the threshold were considered “enhanced area” and the number of enhanced pixels normalized by the total area of the image was quantified for each image. Higher-resolution images were taken using a 60× lens to determine the location of the activated droplets.
Statistical analysis
All data were expressed as the mean ± standard error (SEM) and analysis of variance (ANOVA) was used to compare differences between groups. ANOVA and multiple comparison procedures Kruskal–Wallis post-hoc test were performed using Prism 9 software (GraphPad Software, Boston, MA). A p value <0.05 was considered to indicate statistically significant.
Results
I/R injury enhanced accumulation of PD
The mean size of the PDs was 180 ± 70 nm, with the majority (>90%) of them being less than 275 nm and the mean concentration was 8.1 × 10 10 droplets/mL [ ]. The zeta potential of the PDs before DiI labeling was 0.53 ± 1.2 mV ( n = 21). It is unlikely that there would be significant change in the zeta potential due to fluorescence labeling as the amount of DiI used was less than 0.2% of the lipids used in the formulation, although we did not measure the zeta potential of the DiI-PD directly. Since the zeta potential is very close to neutral, it is doubtful that the droplets will have significant non-specific binding and/or endocytosis secondary to any net positive charge.
Retention and acoustic activation of intravenously injected DiI-PDs were observed in the microvasculature in the cremaster tissue in fluorescent mode ( Fig. 2 ). The fluorescent signals were stationary, indicating that these droplets were retained in the tissue microenvironment before being acoustically activated by imaging pulses. With the setting used for this study, the nanodroplets were not visible in fluorescence mode due to their nanometer sizes and the compact arrangement of fluorophores. Once they were activated by ultrasound, the nano-sized droplets become micron-sized microbubbles, and the fluorescent signals on these bubbles can be observed. Theoretically, there could be PDs in the blood vessels that get activated. However, these bubbles would flow away from the ultrasound-treated area and fail to be captured. By calculating the total enhanced area of the fluorescent signals normalized by the total area of the images, increased retained PDs were observed in the I/R group compared with the control group with both low-frequency and high-frequency activation ( p < 0.05) ( Fig. 3 ). There was a trend, though not statistically different, toward more intensity area with high-frequency activation compared with low-frequency activation (7 vs. 1.5 MHz) in the I/R groups.
