Vascular dementia (VaD) is a unique form of cognitive decline caused by impairment of blood flow to the brain. Atherosclerosis is strongly associated with VaD as plaque accumulation can lead to tissue hypoperfusion or stroke. VaD and atherosclerosis are both diagnosed relatively late in their disease courses, prompting the need for novel diagnostic approaches such as PET to visualize subclinical pathophysiologic changes. This review discusses the use of PET in the assessment of VaD and cerebrovascular disease, focusing on the application of [18F] fluorodeoxyglucose to study neurometabolism and [18F] sodium fluoride to quantify arterial calcification.
Key points
- •
[18F] fluorodeoxyglucose (FDG) PET reveals specific patterns of hypometabolism in the brain among the various forms of dementia with scattered areas of focal, cortical, and subcortical hypometabolism seen in vascular dementia (VaD).
- •
In the setting of a stroke, reduced FDG uptake is observed in definitive margins at the site of infarction with hypermetabolism seen in the peri-infarct region.
- •
[18F] sodium fluoride (NaF) PET is a highly specific biomarker of high-risk atheroma development that correlates with future risk of cardiovascular and thromboembolic diseases.
- •
Implementation of both FDG and NaF PET imaging may enable earlier detection of microscopic changes associated with VaD and atherosclerosis that precede structural alterations or cognitive impairment.
Introduction
Vascular dementia (VaD) is a progressive cerebrovascular disease (CVD) caused by an impairment of blood supply to the brain, leading to functional disturbances and cognitive decline. Vascular disorders are responsible for cognitive impairment in up to 5% of individuals over the age of 65 years. Due to a wide range of presenting symptoms and variability in disease onset, the diagnosis of VaD remains a significant challenge.
The brain is a highly active organ requiring a constant supply of nutrients and blood flow to ensure the proper functioning of energy-intensive processes. Although the brain comprises just 2% of the total body mass, it receives nearly 20% of the total cardiac output. When blood flow to the brain is compromised as in CVD, ischemic cell death and loss of neuronal function can ensue within minutes, leading to chronic complications. This dysfunction is believed to arise from a complex mechanism of cerebral hypoperfusion, oxidative stress, and neuroinflammation ( Fig. 1 ). , The progression of cell death and efficacy of potential therapeutic interventions are time-dependent, which raises the need to devise approaches to identify early pathologic changes associated with CVD.
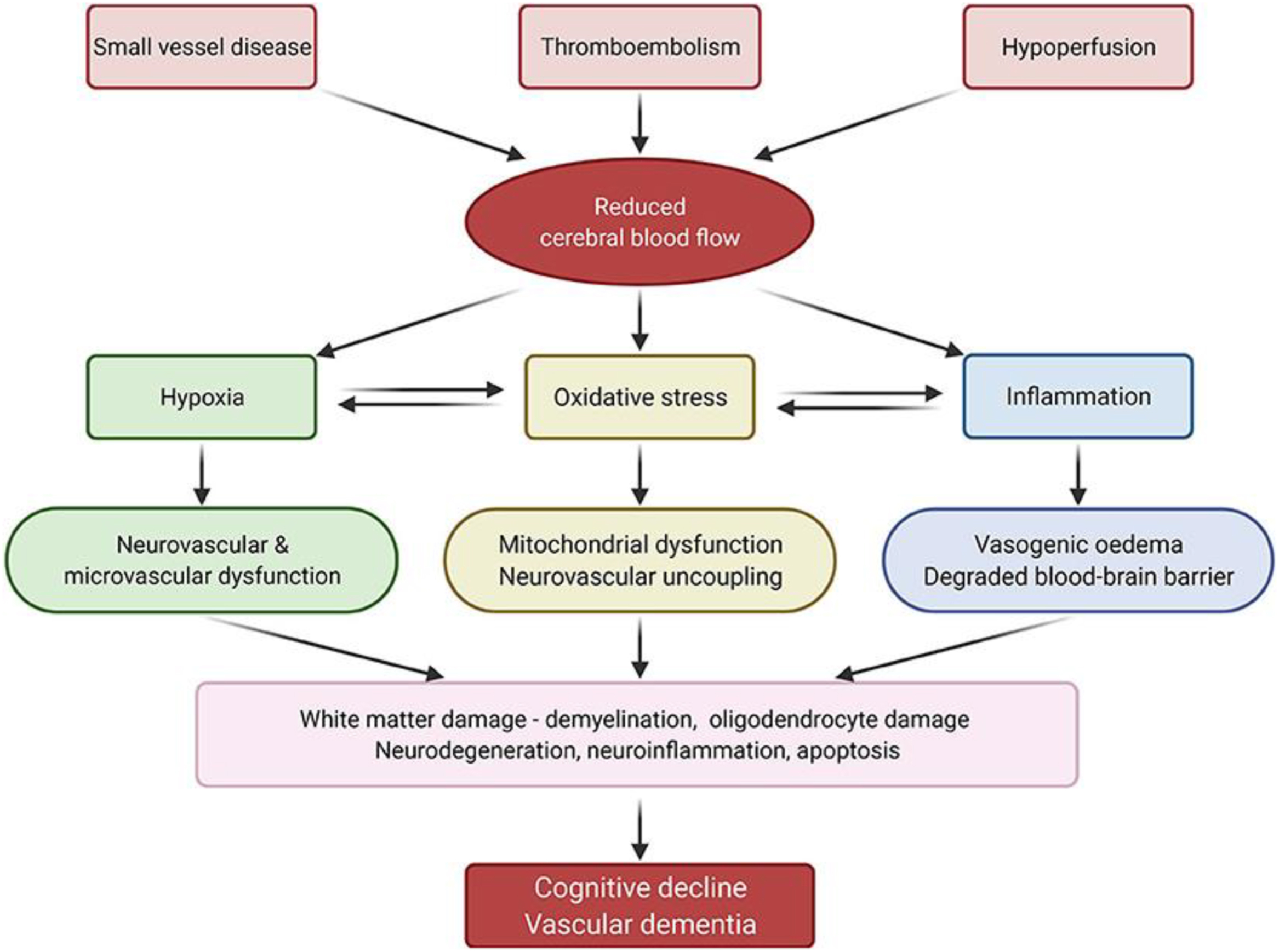
VaD is the second most common form of dementia following Alzheimer’s Disease (AD) and can be classified into various subtypes: strategic infarct dementia, multi-infarct dementia, subcortical dementia, and mixed dementia. Strategic infarct dementia results from a single large ischemic stroke that restricts blood flow to the brain causing acute cognitive deficits, particularly when the infarction occurs in the thalamus, angular gyrus, or basal ganglia. Multi-infarct dementia arises from multiple territorial infarcts in cortical regions that lead to stepwise cognitive decline such as apraxia, aphasia, or visual field cuts. In subcortical dementia, chronic disease of the small vessels supplying the subcortical white matter results in gradual perfusion deficits leading to a decline in information processing, memory, and other executive functions. Mixed dementia refers to a combination of dementia pathologies, most commonly of VaD and AD. Patients with mixed dementia present with overlapping clinical phenotypes due to the presence of interrelated neurovascular and neurodegenerative processes.
The restriction of blood flow to the brain in the setting of VaD is most frequently caused by cerebrovascular atherosclerosis and thromboembolism. Atherosclerosis is a chronic inflammatory disease characterized by the accumulation of lipid-laden plaques inside the walls of large- and medium-sized blood vessels. Over time, atheroma development leads to blood vessel narrowing and tissue hypoperfusion and may pose a significant risk of causing a stroke or transient ischemic attack when the plaque ruptures and forms a platelet-rich thrombus or dislodges and obstructs cerebral blood flow in the smaller arteries of the brain (ie, thromboembolism). Accordingly, atherosclerosis and VaD share several common risk factors, including hypertension, obesity, diabetes, and advanced age. Acute stroke is considered to be the greatest risk factor for VaD, though chronic disruption in brain perfusion due to atherosclerotic stenosis is also a significant contributor to the more gradual onset of cognitive decline.
Conventional structural imaging, such as computed tomography (CT) and MR imaging, plays a critical role in the clinical management of patients with CVD. MR imaging remains the key neuroimaging modality of VaD and is preferred to CT because of its higher sensitivity and specificity when detecting pathologic changes. Conventional MR imaging can visualize markers of VaD, such as cerebral microbleeds, silent infarcts, and white matter changes. , Structural imaging approaches, however, have limited ability to capture the early biologic underpinnings of VaD. The gradual ischemia and loss of neurons that begin at the microscopic level cannot be visualized by CT or MR imaging until these changes manifest as gross atrophy, at which point disease progression is irreversible. It is in this context that molecular imaging with PET may serve a critical role in the early detection of VaD.
For over 4 decades, [18F] fluorodeoxyglucose (FDG) PET has been used to identify metabolic changes in neurologic diseases including dementia. As an analog of glucose, FDG is irreversibly taken up by neurons and serves as a measure of glycolytic activity in the brain. Among the various forms of dementia, alterations in glucose metabolism, as assessed by FDG PET, have been shown to manifest in patients before the development of cognitive impairment or structural changes in the brain.
Atherosclerosis is a chronic CVD that cannot be visualized by structural imaging until late in its disease course. The progression of atherosclerosis involves a stress (ie, hypertension, lipid retention) that leads to dysfunction of endothelial cells lining the arterial vessels, which induces localized inflammation and formation of fibrous plaque. The inflammation and microcalcification of plaques that underlie this disease can be detected by FDG and [18F] sodium fluoride (NaF) PET, respectively. FDG has been extensively studied in atherosclerosis given the high metabolic activity that characterizes the inflammatory state, but data supporting its use are mixed. Calcification as assessed by NaF PET, on the other hand, is a sensitive and specific marker of early-stage (ie, subclinical) atherosclerosis.
This article focuses on the role of FDG PET in detecting VaD and NaF PET in imaging atherosclerosis preceding symptomatic or structural changes to emphasize how this approach can be used to fully characterize VaD risk. Pertinent research findings and future directions will also be discussed in the context of CVD.
The role of [18F] FDG PET in dementia
Patterns of metabolic activity, as assessed by FDG PET, enable the distinction of normal age-related cognitive decline from dementia. In normal aging, axonal degeneration and progressive regression of dendritic trees result in neuronal atrophy that drives cognitive decline. Uptake of FDG is diffusely reduced throughout the brain, with the greatest decreases observed in the bilateral cingulate cortices, parietal regions, and superior temporal lobe. ,
The cognitive dysfunction observed in VaD arises from neurovascular mechanisms separate from that of normal aging, leading to the death of neurons. As observed with FDG PET, VaD and each of its subtypes manifest distinct changes in glucose metabolism relevant to their unique pathology. In multi-infarct VaD, FDG PET reveals scattered regions of focal cortical and subcortical hypometabolism that differ from the pattern of tempo-parietal hypometabolism seen in AD ( Fig. 2 ). Kerouche and colleagues demonstrated decreased FDG uptake in the deep gray nuclei, cerebellum, primary cortices, middle temporal gyrus, and anterior cingulate gyrus in patients with subcortical VaD relative to those with AD. In contrast, patients with AD were found to have lower metabolism in the hippocampal region, orbitofrontal, posterior cingulate, and posterior parietal cortices relative to patients with VaD. The voxel-based multivariate analysis employed by Kerouche and colleagues separated VaD from AD with 100% accuracy as well as healthy controls from VaD and AD with 72% and 96% sensitivity, respectively.
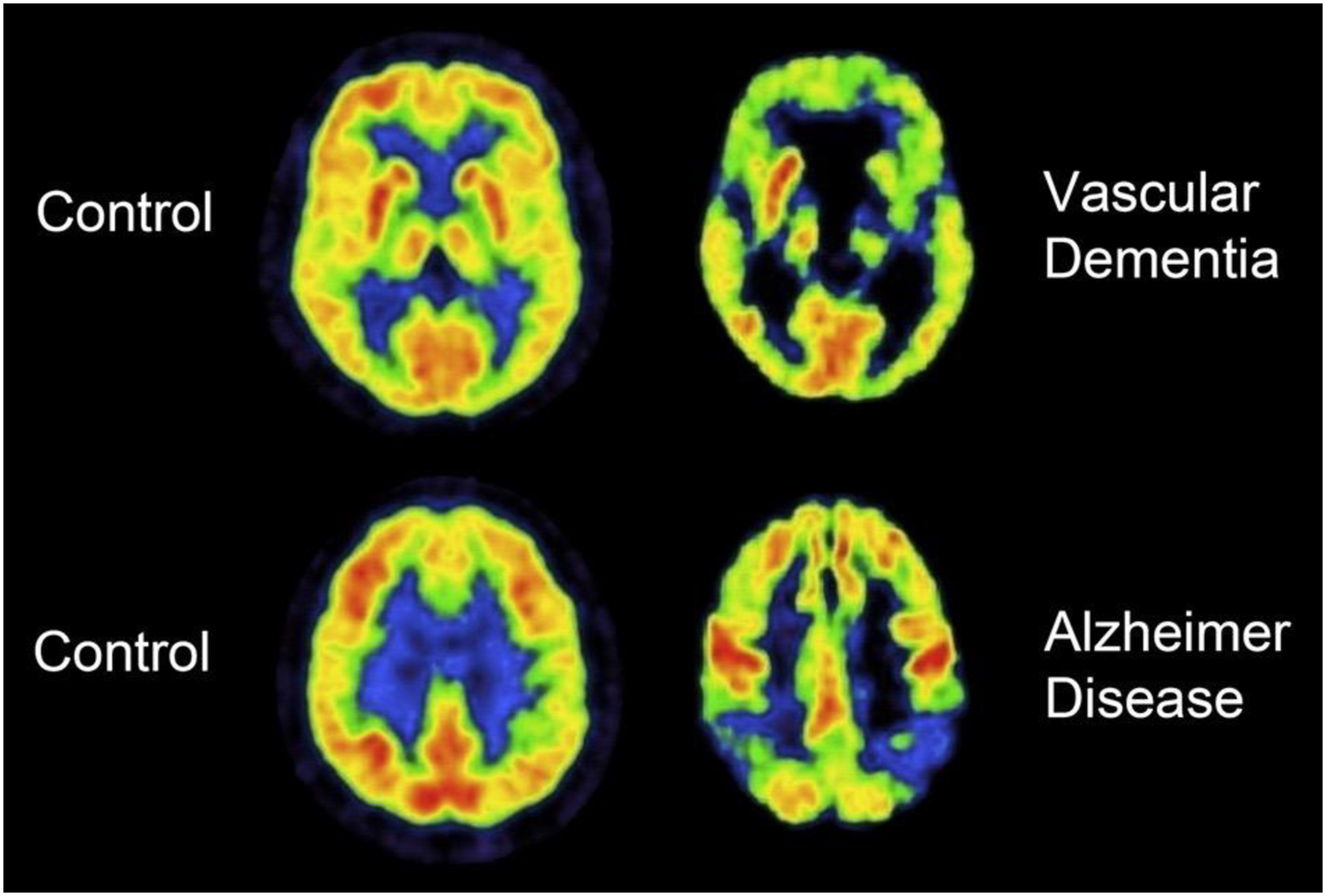
In the setting of a single strategic infarct, FDG PET demonstrates reduced glucose metabolism in definitive margins around the area of infarction—typically the anterior, middle, or posterior cerebral artery—with concomitant encephalomalacia visible on CT. Infarction of the frontal lobe or internal capsule may result in hypometabolism in the contralateral cerebellum, a phenomenon known as crossed cerebellar diaschisis ( Fig. 3 ). , In the area surrounding the infarcted region, also known as the ischemic penumbra, several studies have reported increase in FDG uptake. , This hypermetabolism may be driven by an increased demand for anaerobic glycolysis or inflammation in the peri-infarct region.
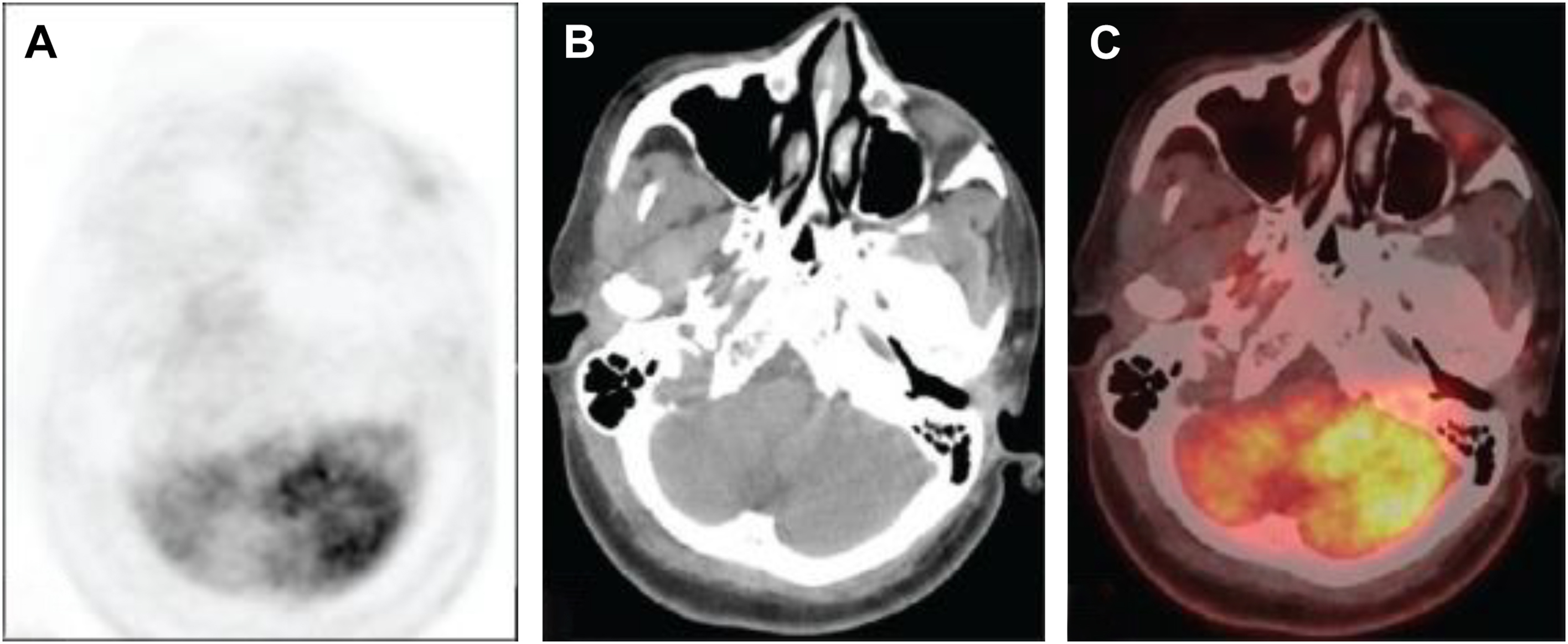
From a clinical perspective, a major advantage of FDG PET over other imaging modalities is its ability to detect metabolic changes in the brain long before the onset of VaD symptoms. In a study of 14 patients with CVD risk factors, Borja and colleagues identified significantly lower global brain FDG uptake relative to the matched controls. Nam and colleagues observed hypometabolism in the frontal cortex of 264 patients with metabolic syndrome, which was most strongly predicted by levels of fasting blood glucose and glycated hemoglobin (HbA1c). Other studies have further demonstrated negative associations of brain FDG metabolism with body mass index (BMI), serum cholesterol, and hyperglycemia. Taken together, these findings point to an association between CVD risk and subclinical metabolic alterations in the brain detected by FDG PET.
FDG PET has additionally elucidated the association between early-stage atherosclerosis and VaD. In an analysis of 547 asymptomatic middle-aged participants with evidence of subclinical atherosclerosis from the Progression of Early Subclinical Atherosclerosis (PESA) study, Cortes-Canteli and colleagues. showed that global FDG uptake is inversely correlated with the 30-year Framingham Risk Score of CVD risk. Carotid plaque burden assessed by a 3-dimensional vascular ultrasound was found to have an inverse association with global brain FDG uptake. A voxel-based approach indicated that the regions of the brain most susceptible to CVD risk factors included the parietotemporal areas (eg, angular, supramarginal, and inferior/middle temporal gyri) and the cingulate gyrus.
A separate longitudinal study from the PESA cohort revealed an accelerated decrease in cortical glucose metabolism among patients with persistent high CVD risk relative to those with low risk, shown by a comparison of baseline and follow-up FDG PET scans (median follow-up of 4.7 years). In particular, patients with high CVD risk demonstrated an average reduction in global brain metabolism that was nearly 3 times that of patients with low risk (4.3% vs 1.5%). Furthermore, progression of subclinical carotid atherosclerosis was related to cerebral hypometabolism in the temporal gyrus, hippocampus, occipital lobe, precuneus, and angular gyrus. These regions strongly overlap with those known to be impaired in patients with dementia, particularly AD and VaD. Indeed, the presence of CVD may directly lead to the onset of dementia as in VaD, or may increase the vulnerability of neurons to neurodegenerative pathology as in AD. The latter point is further supported by findings of significant elevations in the neurofilament light chain, a marker of axonal injury, among patients with high CVD risk.
The role of [18F] NaF PET in atherosclerosis
The role of atherosclerosis in the etiology of dementia has become increasingly notable. In a longitudinal study of 2364 neurologically asymptomatic patients, Bos and colleagues reported correlations between dementia risk and increased calcification volume in the aortic arch, extracranial, and intracranial carotid arteries as assessed by CT. This association was strongest in the extracranial carotid arteries, but the presence of correlations with dementia for other vascular beds led the authors to conclude that atherosclerosis influences dementia pathology not only through its association with stroke but also through its role in chronic cortical ischemia.
Given the relationship between atherosclerosis and dementia, detecting the accumulation of atherosclerotic plaques in the arteries supplying the brain is critical for risk stratification of VaD. Structural imaging modalities such as ultrasound, CT, and MR imaging have limited ability to visualize early-stage manifestations of atherosclerosis as the macroscopic structural alterations detected by these techniques represent an advanced form of the disease. In contrast, PET is capable of imaging the microscopic molecular changes associated with atheroma development—inflammation (FDG) and microcalcification (NaF). The role of FDG in this domain is considered controversial as inflammation is a relatively nonspecific marker of atherosclerosis. Macrophages are the predominant inflammatory cell type to take up FDG, and they are found diffusely in both early-stage fatty streaks and late-stage vulnerable plaques. The presence of significant inflammation across different states of disease progression therefore complicates accurate atherosclerotic risk stratification with FDG PET. Indeed, Blomberg and colleagues found that uptake of NaF, but not FDG, in the thoracic aorta was associated with increased CVD risk. Similarly, Arani and colleagues reported a correlation between abdominal aorta NaF uptake and CVD factors that was not observed with FDG.
In contrast to FDG, NaF is a more specific marker of arterial microcalcification that distinguishes high-risk plaque formation ( Fig. 4 ). Irkle and colleagues demonstrated in carotid endarterectomy samples that NaF preferentially binds to areas of active microcalcification over areas of macrocalcification, the latter of which characterizes stable plaques visible on CT. In further support of this claim, a histopathologic study by Youn and colleagues observed high NaF activity in vulnerable coronary artery plaques and low NaF activity in plaques with macrocalcification. A prospective clinical trial by Joshi and colleagues also demonstrated correlations between carotid artery NaF activity and intravascular ultrasound findings of plaques with a high risk of rupture: positive remodeling, microcalcification, and the presence of a necrotic core. NaF uptake was significantly elevated in culprit versus nonculprit lesions as determined by angiography.
