Radiation-induced injuries (RIIs) are significant complications of radiation therapy used in cancer treatments and affect organs in a systemic fashion such as the heart, lungs, liver, and bone marrow. Such ionizing radiation leads to inflammation, fibrosis, and/or irreparable DNA damage, each of which can significantly impact patient’s quality of life, underscoring the need for advanced diagnostic and imaging techniques. A novel combination of PET/Computed Tomography (CT) with Quantitative MR Imaging has emerged as a crucial tool for early diagnosis and timely evaluation of RIIs. This review focuses on the important role of quantitative PET-CT-MR imaging in diagnosing and monitoring RIIs.
Key points
- •
PET/CT imaging detects tissue subclinical metabolic changes exposed to radiation and can be an early marker for inflammatory/fibrotic changes, particularly in cardiopulmonary and hepatic pathology.
- •
Quantitative MRI complements the visualization of PET/CT metabolic changes with high-resolution structural and volumetric data, enhancing interpretation of PET findings.
- •
Quantitative PET metrics serve as biomarkers for detecting early changes in affected organs via volumetric and metabolic data from novel combination diagnostic imaging modalities.
- •
Integration of quantitative MRI with PET/CT imaging can provide a diagnostic framework that can identify and potentially prevention and RIIs.
CT | computed tomography |
dPET | dynamic PET |
FDG | fluorodeoxyglucose |
GLG | global lung glycolysis |
HD | high-dose |
HMVP | Hepatic Metabolic Volume Product |
HT | hematological toxicity |
RII | Radiation-induced injury |
RILI | radiation-induced lung injury |
RP | radiation pneumonitis |
RT | radiation therapy |
SUV | standardized uptake value |
TNF-β | transforming growth factor-beta |
Introduction
Radiation-induced injuries (RIIs) are an important concern in clinical practice that commonly arises from ionizing radiation exposure during cancer treatments. Such injuries affect systemwide organs, in both acute and chronic forms. The pathophysiology of RIIs involves intricate interactions between apoptotic and necrotic pathways as a result of the direct oxidative damage caused by the ionizing radiation. The free radicals lead to DNA damage and a resulting cascade involving the release of neutrophils, cytokines, and interleukins that can ultimately lead to cell death of both cancerous and normal cells. The resulting tissue damage can lead to complications that greatly decrease the quality of life of patients, and thus understanding the cellular alterations that underlie RIIs is critical for improvement of diagnostic tools and refining therapies and preventative measures.
Molecular imaging, particularly with PET-computed tomography (CT), has emerged as a tool for detecting the systemic effects, both chronic and acute, associated with RIIs. 18F-fluorodeoxyglucose (FDG)-PET imaging visualizes increased glucose metabolism in inflamed tissues, which offer valuable insights into early pathologic changes critical for timely detection of such injuries. For instance, in radiation-induced lung injury (RILI), FDG-PET identifies inflammation through elevated uptake metrics such as global lung glycolysis (GLG) and total lesion glycolysis, which have shown promise as biomarkers for predicting and monitoring pneumonitis and fibrosis. The cardiovascular system, also susceptible to radiation-induced damage, poses severe risks from diseases such as myocarditis, coronary artery disease, and vascular calcification. FDG-PET allows for the assessment of myocardial inflammation and vascular injuries, and such imaging biomarkers provide insight on dose-dependent radiation effects, aiding in risk stratification, and targeted therapy. Similarly, radiation injuries to the liver and bone marrow also require diagnostic approaches. Advanced segmentation techniques, from a combination of PET and MR imaging data, have been shown to offer a comprehensive assessment of liver metabolism and structural alterations, as well as evaluation of red marrow metabolism. Unique to the concurrent use of PET-MRI is the ability to detect structural changes along with the quantification of tissue pathology in subclinical patients; further accuracy can be ensured with advanced segmentation techniques and the fusion of both biochemical and anatomic data allow for a novel approach to mitigating long-term complications. ,
This article focuses on the important role of quantitative PET-CT-MR imaging in diagnosing and monitoring radiation-induced injuries. The novel techniques will allow for accurate injury assessment with an emphasis on earlier detection and timely preventative measures.
Radiation-induced injuries
Mechanism of Injury
RII results from both direct and indirect interactions between ionizing radiation and biological tissues; this, in turn, leads to molecular, cellular, and systemic damage. On the molecular level, radiation deposits energy that ionizes atoms and disrupts chemical bonds, directly damaging critical biomolecules such as DNA. Indirectly, this energy triggers the radiolysis of water, generating reactive oxygen species such as hydroxyl radicals, that cause oxidative damage to DNA, proteins, and lipids; cellular responses to this damage includes impaired DNA repair, apoptosis, or senescence, with varied outcomes dependent on the severity of the injury and the cell type. These tissue-level effects manifest as inflammation, fibrosis, and vascular injury, driven by cytokine release, immune cell infiltration, and endothelial dysfunction. , Furthermore, high doses of irradiation or chronic radiation exposure (such as for reirradiation in the setting of locoregional recurrences) may disrupt organ function due to cumulative toxicity and impaired regenerative processes. Imaging techniques such as PET enable the quantification of such effects, offering insights into metabolic and structural changes within irradiated tissues, including normal tissues receiving radiation dose.
Normal Anatomy and Imaging Technique
Understanding the normal anatomy and imaging techniques for radiation-sensitive organs is crucial for accurately identifying and managing RIIs. The lungs, heart, liver, and bone marrow are among the most affected organs during radiation therapy (RT), each with unique anatomic features and susceptibilities. The lungs, vulnerable at levels of the lobes, bronchi, alveoli, or vasculature, play a vital role in gas exchange and are prone to radiation-induced damage such as pneumonitis and fibrosis. Structural and functional imaging, particularly through CT and FDG-PET, help in assessing these injuries. Similarly, the heart’s complex anatomy—including the myocardium, coronary arteries, valves, and pericardium—renders it vulnerable to complications like myocarditis and pericarditis; echocardiography, cardiac MRI, and FDG-PET are invaluable tools in evaluating myocardial metabolism and inflammatory changes. The liver, with its lobular structure and vascular portal triad, can experience radiation-induced veno-occlusive disease and hepatocellular injury, which are effectively evaluated using MR imaging and PET imaging techniques. Meanwhile, the bone marrow, critical for hematopoiesis, is susceptible to cellular depletion and fibrosis, with whole-body MR imaging and FDG-PET offering insights into marrow health and function.
PET imaging techniques play a central role in detecting and quantifying radiation-induced organ damage. FDG-PET assesses metabolic activity, providing a sensitive measure of inflammation and damage. Standardized Uptake Value (SUV) metrics derived from PET images provide a semi-quantitative method of tracking metabolic changes and monitoring therapeutic responses. Beyond FDG, novel tracers, such as 18F-fluoromisonidazole and 68Ga-fibroblast activation protein inhibitor, have expanded the scope of PET imaging, enabling detailed analyses of tissue hypoxia and fibrosis, respectively. These advancements offer unparalleled insights into the chronic effects of radiation on various organs and facilitate tailored therapeutic interventions.
Advanced imaging protocols have further refined the precision of evaluating RIIs by addressing limitations such as partial volume effects. MRI-based segmentation techniques, leveraging T1-weighted and T2-weighted imaging, as well as diffusion-weighted imaging, allow for detailed visualization and quantification of organ-specific damage. PET-MRI co-registration mitigates partial volume errors, enhancing the accuracy of metabolic and structural assessments. , Dynamic imaging modalities, including dynamic contrast-enhanced MRI and dynamic PET imaging, provide valuable data on tissue perfusion and tracer kinetics, enabling early detection of radiation-induced vascular and parenchymal changes. , , Together, these sophisticated imaging techniques can enhance our understanding of radiation-induced pathology and support the development of strategies to mitigate its adverse effects.
Specific Toxicities
Pulmonary toxicity
RILI is one of the most significant dose-limiting complications of RT in thoracic cancers. It presents via 2 main forms: acute radiation pneumonitis (RP) and chronic radiation-induced pulmonary fibrosis. RP typically develops within weeks to months after RT completion and is characterized by alveolar damage, increased capillary permeability, and neutrophilic infiltration due to elevated levels of pro-inflammatory cytokines such as interleukin-1, tumor necrosis factor-alpha, and transforming growth factor-beta (TGF-β) ( Fig. 1 ). Clinically, patients may experience nonproductive cough, dyspnea, and fevers. Chronic progression to pulmonary fibrosis, which occurs months to years later, results from prolonged alveolar and vascular damage; this process involves excessive collagen deposition by fibroblasts, leading to irreversible scarring, reduced lung compliance, and impaired gas exchange. Molecular imaging with FDG PET has enabled earlier detection of inflammatory changes, enhancing the ability to intervene before fibrotic changes develop. However, despite therapeutic advancements, such as intensity-modulated RT, stereotactic body RT, and proton therapy, mitigating pulmonary toxicity remains a challenge in thoracic RT.
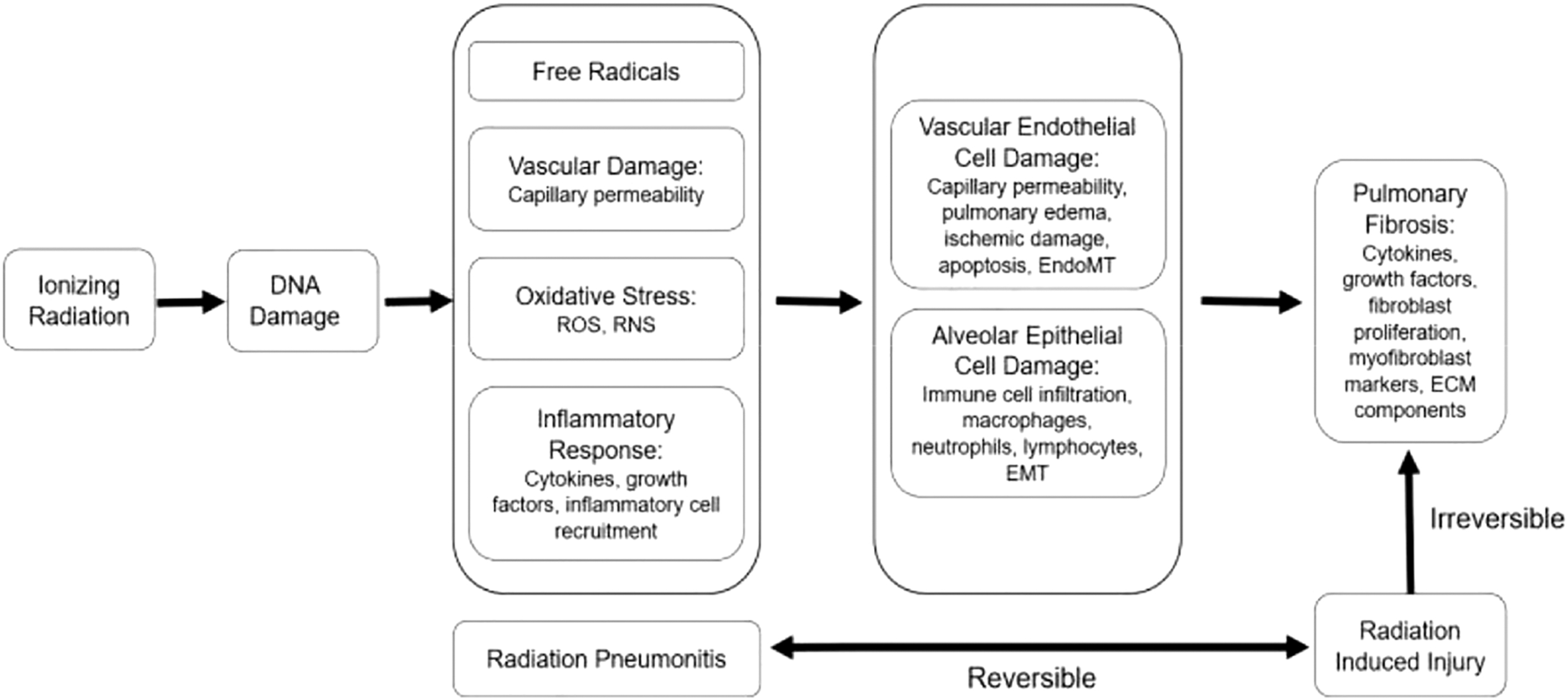
Cardiovascular toxicity
Cardiovascular toxicity is a critical late effect of RT, particularly in patients treated for malignancies of the breasts, lungs, or mediastinum. , RT induces endothelial damage, disrupts vascular integrity, and triggers chronic inflammation, promoting pathologic processes such as accelerated atherosclerosis, myocardial fibrosis, and pericardial inflammation. Key molecular pathways, such as TGF-β, drive the fibrotic remodeling of cardiac tissues. Patients face an elevated risk of coronary artery disease, valvular dysfunction, arrhythmias, and heart failure, which typically manifest years to decades after treatment. Concurrent systemic therapies, such as anthracyclines or trastuzumab, may synergistically exacerbate these risks. PET imaging has shown potential for early detection of subclinical cardiac injury. Increased FDG uptake in cardiac tissues can signify early inflammatory responses, such as atherosclerosis, enabling timely interventions and appropriate referral to specialty care providers in cardiology ( Fig. 2 ). The integration of advanced imaging modalities into routine post-treatment surveillance can improve the long-term cardiovascular burden associated with RT.
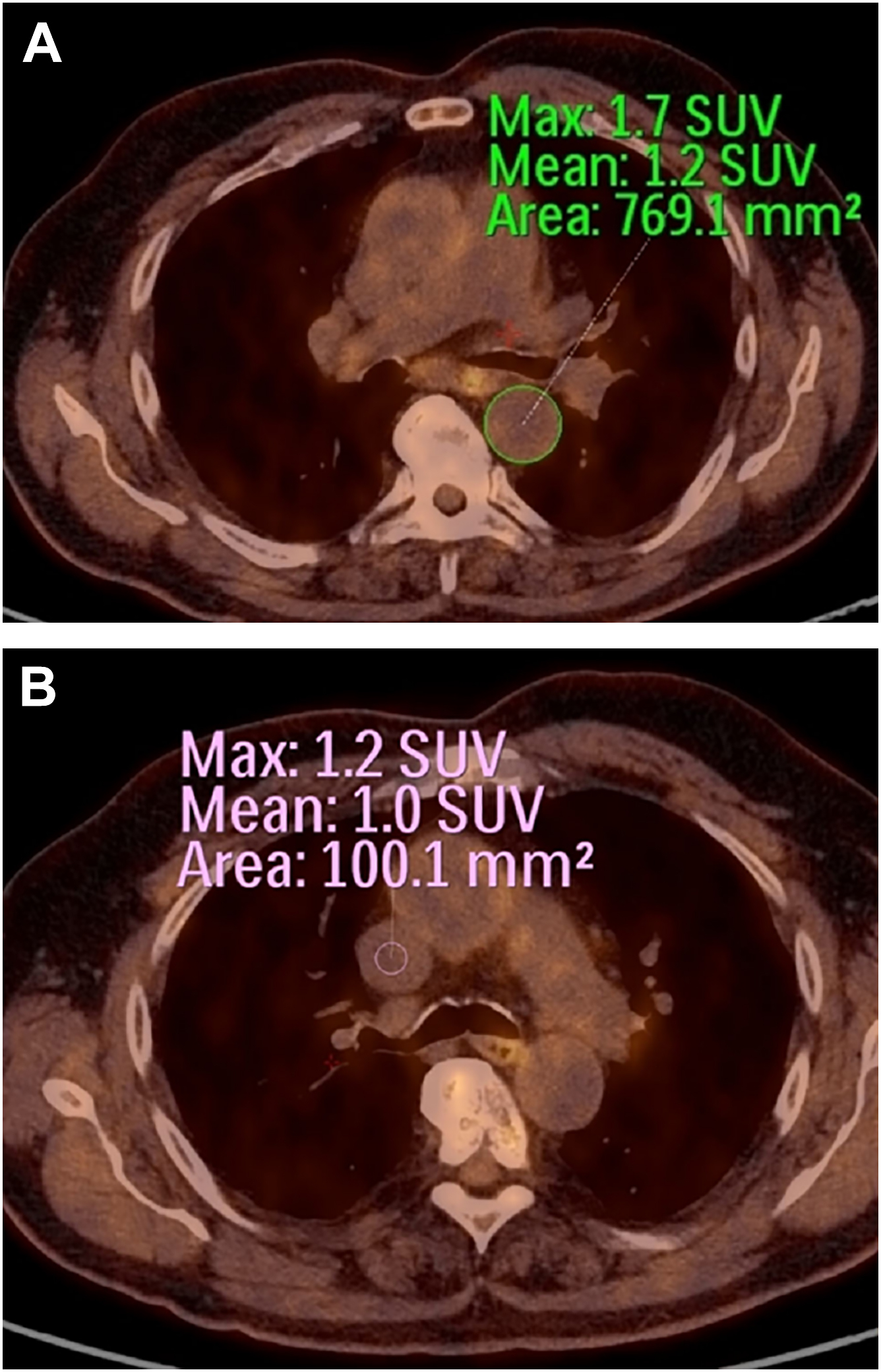
Bone marrow suppression and hematologic considerations
RT often impacts healthy tissues, including bone marrow—the central site of blood cell production. Due to their high mitotic activity, bone marrow cells are particularly susceptible to radiation-induced DNA damage, particularly to progenitor and stem cells. These injuries disrupt the marrow’s ability to generate red blood cells, white blood cells, and platelets, potentially leading to conditions such as anemia, leukopenia, and thrombocytopenia, respectively.
In addition to direct cellular damage, radiation can harm the bone marrow microenvironment, including vascular structures that further impair hematopoiesis. Mesenchymal stem cells, which support the hematopoiesis, have been found to sustain greater radiation-induced damage than hematopoietic stem cells themselves, which worsens the overall impact on marrow function. , The clinical effects of radiation-induced injury vary widely and are often dose-dependent, with broader exposure leading to more severe and prolonged suppression of marrow activity and resulting anemia.
Research by Muralidharan and colleagues demonstrates that even low doses of proton and iron ion radiation can significantly decrease early and late multipotent progenitor cell numbers in mouse bone marrow for up to 10 months after exposure. Similarly, another study found that peripheral blood changes, including reductions in blood cell counts, can occur at radiation doses below 1 Gy. These findings underscore the profound sensitivity of bone marrow to radiation and the long-lasting nature of the damage. While RT remains an indispensable tool in oncology, its effects on bone marrow highlight the need for protective strategies and diagnostic tools to mitigate these side effects.
Quantitative PET metrics
Overview
Quantitative PET metrics offer precise, objective measurements that can enhance the understanding of disease burdens and assist in the generation of a treatment response. SUV—including SUVmax, SUVmean, and SUVpeak—has been widely used as a semi-quantitative metric of uptake in FDG-PET/CT for the diagnosis of malignant tumors and evaluation of tumor therapies. SUV allows for a detailed analysis of metabolic activity within specific regions, providing a more detailed understanding of tissue activity than can be assessed by visual analysis alone. Other semi-quantitative metrics, such as Metabolic Tumor Volume and Total Lesion Glycolysis, can quantify the volume and total metabolic burden of active tissues; together, these metrics provide a broad evaluation of tumor metabolic activity and are particularly valuable for assessing heterogeneity within affected tissues ( Fig. 3 ). , However, as semi-quantitative metrics, they have many downsides such as vulnerability to intra-individual and inter-individual variability influenced by biological factors (eg, patient weight, blood glucose levels, and respiratory movements) and technical factors (eg, scanner type, uptake time, and inaccurate calibration of the PET scanner or use of contrast agent). , Additionally, differences in size, shape, and thresholding methods of the region of interest further complicate the reproducibility and reliability of these metrics, often leading to different results for the same lesion. These variations can lead to inconsistent measurements that complicate clinical interpretation.
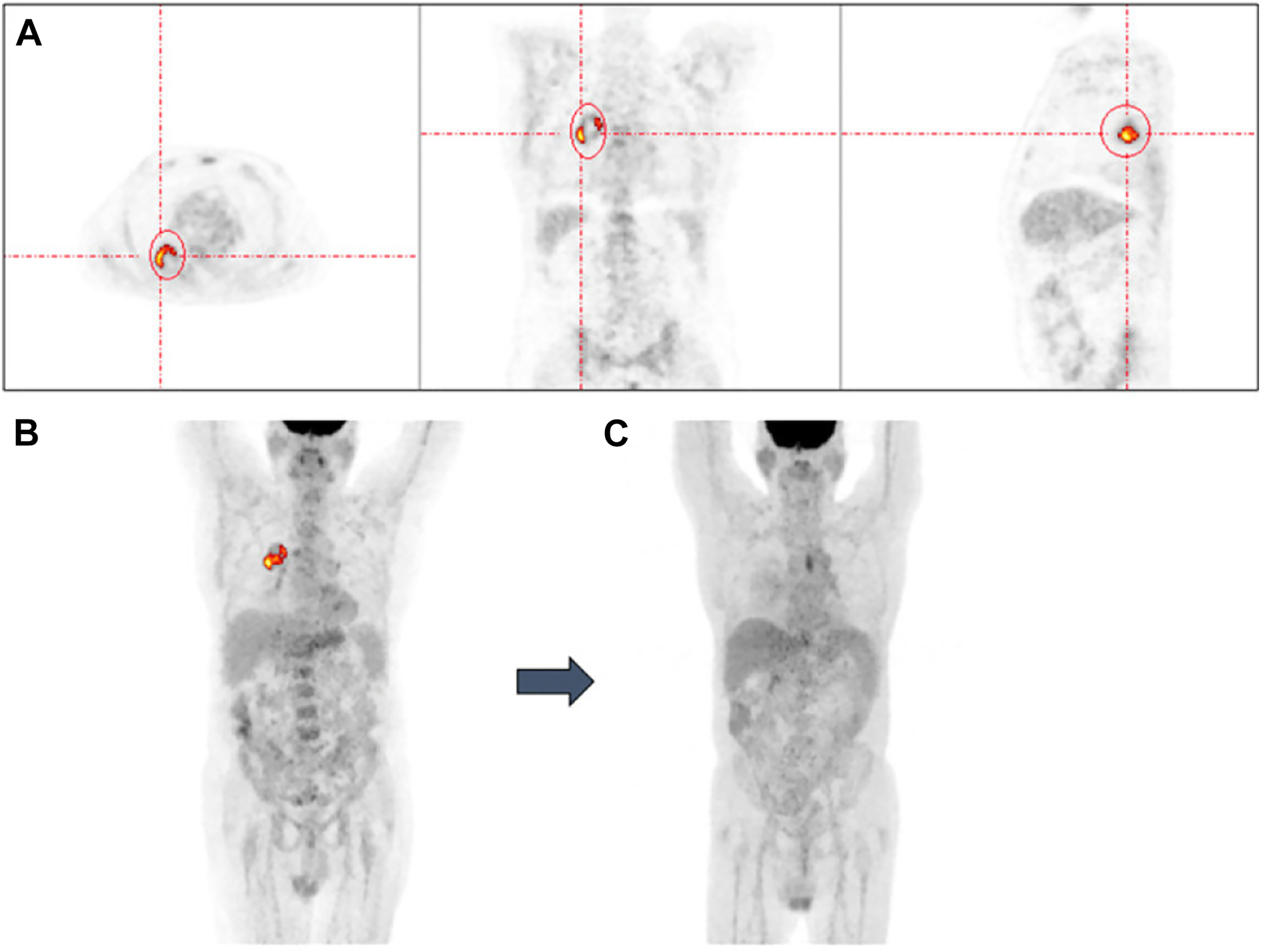
In contrast, fully quantitative PET metrics eliminate much of these variabilities and guesswork inherent in semi-quantitative analyses, leading to much greater precision. Dynamic PET (dPET) imaging is one such method that involves the calculation of kinetic parameters. These parameters, such as k1 (tracer delivery rate), k2 (backflow rate), k3 (rate of tracer trapping in tissue), k4 (rate of tracer release or dephosphorylation), and Ki (net influx rate), provide detailed descriptions of the rates of tracer delivery, uptake, and retention within tissues, generating more accurate insights into how tracers are distributed and absorbed by metabolically active tissues ( Fig. 4 ). Relative to SUV metrics, dPET has been shown to improve detection of tumor progression from radiation injury in patients with brain malignancies. The combination of these parameters with SUV data has also been shown to improve the delineation of benign and malignant tissue, as well as enhance the accuracy of tumor grading. ,
