PET Viability Studies
Henry Gewirtz
Definitions of Myocardial Hibernation, Stunning, and Viability
As in any area of medicine, especially one that has more than its share of controversy, it is important to define terms if one is to have any hope of making sense of the problem. Although the pathophysiology of myocardial hibernation and stunning remains controversial, the following points are clear. Both entities share in common impaired contractile function of the left ventricle. Classically, stunning was thought to differ from hibernation since the former was characterized by relatively normal myocardial blood flow and myocardial oxygen consumption out of proportion to the decreased contractile function of the myocardium involved.1,2,3,4 In contrast, hibernation classically was thought to involve a matched reduction in myocardial blood flow, contractile function, and oxygen consumption.1,2,3,4 In patients with ischemic heart disease and in animal models,5,6 PET studies have demonstrated that both patterns of myocardial blood flow and impairment contractile function may exist, sometimes even in the same subject.7 It also appears to be true that chronic stunning often precedes hibernation.8,9 It has also been suggested that hibernation may occur in the absence of prior stunning.1,6,10 Further, the underlying histopathology is also somewhat controversial but has been characterized by glycogen accumulation, myocyte dedifferentiation, increased interstitial fibrosis, and in some but not all series, evidence of myocyte loss by apoptosis.1,11,12,13,15 A detailed review of the data relevant to this controversy is beyond the scope of this chapter. What is important for the purposes of this review is that both states, however arrived at, are characterized by dysfunctional myocardium, and both have the potential to improve with myocardial revascularization. Thus, there is a need to distinguish viable myocardium (i.e., hibernating or stunned) from nonviable myocardium, which is largely composed of scar tissue.
Myocardial viability, in turn, has been defined in one of two ways. The first employs what may be termed a cellular definition. This approach rests on the notion that accumulation of various radiotracers (e.g., Thallium, technetium-99m-sestamibi, rubidium-82) requires cellular concentrating mechanisms such as the sodium-potassium ATPase pump (Thallium, rubidium-82) or the electrochemical gradient of intact mitochondria (Tc-99m-sestamibi), and since these pumps require energy to function, a cell that can accumulate the tracer of interest must be viable.16,17 An alternate and perhaps more widely accepted definition holds that viable myocardium is best characterized by return or improvement of contractile function following coronary revascularization.18,19,20 The argument in support of this definition runs to the effect that what is important to the individual is whether or not myocardium with impaired contractility is functionally improved when it is revascularized. A surrogate end point such as tracer accumulation, although it is a marker for an energy-requiring metabolic process, has a lesser oxygen consumption requirement (only about 20% of basal MVO2) than that of contraction, which ultimately is what the patient lives or dies by. The argument against this definition is the often subjective nature of contractile function assessment, typically by echocardiography, though gated MRI has also been used, and the fact that myocardial tethering, interstitial fibrosis, and cellular dedifferentiation all may occur, so that even if the segment(s) in question are successfully revascularized, contractile function still may not be restored depending on when contractile function is reevaluated. Nonetheless, the improvement of contractile function of the left ventricle following coronary revascularization remains the most widely accepted definition of myocardial “viability,” and those tests that best predict this outcome are thought to be the best for viability assessment.
Methodology
SPECT (Tl , MIBI)
The first radionuclide tracer to be employed for the assessment of myocardial viability was Thallium. The tracer has some properties of a potassium analog, so accumulation of the tracer in the myocardium is thought to be indicative of functioning Na-K ATPase pumps. The tracer is typically injected with the patient at rest, and images of the heart are obtained 10–15 minutes later and again at periods varying from 4–24 hours later. The utility of the 24-hour time point, however, is controversial and there are data that show that whatever redistribution (see below) is destined to occur does so within 4 hours and that waiting longer to image does not improve upon this, especially since 24-hour images are count poor and may be very difficult to interpret.21
Since the initial distribution of Thallium tends to be blood flow dependent and late images mark the viable Na-K ATPase pump pool, initial defects that appear to resolve on delayed images are most specific for myocardial viability. The original description of this finding was reported in 1979 prior to widespread appreciation of the concepts of myocardial hibernation and stunning, although the report in fact noted that such segments must have both low flow and viability22 (i.e., exhibit what is now termed myocardial hibernation). Since then it also has been shown that milder fixed defects on Thallium scans also have substantial viable myocardium and are likely to improve contractile function upon successful revascularization.21
When improvement of left ventricular contractile function, either global or segmental, is used as the “gold standard” for myocardial viability, the rest redistribution Thallium study, as it is commonly known, has been shown to be quite sensitive for detection of viable myocardium, but specificity and hence overall predictive accuracy has been variable with a range of roughly 60%–75%, though with high negative predictive accuracy in the range of 90%–95%.23,24,25 Stated in other terms, if the rest redistribution Thallium study is negative for myocardial viability, then it is highly unlikely such segments will recover or improve function upon revascularization. In contrast, if the segment either shows definite redistribution or a fixed but relatively mild defect and hence viability, it is more likely than not that contraction will improve after revascularization, especially if the segment is hypokinetic as opposed to akinetic or dyskinetic.24
Although rest Tc-99m-MIBI scans are less commonly employed for viability assessment, reported results23 have been comparable to that of Thallium, notwithstanding the fact that MIBI does not exhibit appreciable redistribution. Rest MIBI scans obtained 1 hour post tracer injection have been shown to closely resemble redistribution Thallium images and hence provide comparable information regarding cellular viability.23 The performance of the tracer in predicting recovery of contractile function of myocardium considered to be viable by this technique likewise is comparable to that of Thallium.23 The positive and negative predictive accuracies for recovery of contractile function in the series of Udelson et al.23 was good (Thallium positive predictive accuracy 75% vs. MIBI 80%, and negative predictive accuracy 92% and 96%, respectively). However, as noted above other reports have not been as favorable for Thallium24,25 with respect to positive predictive accuracy.
A newer approach, which has been employed in both Japan and Europe for assessment of myocardial viability, has been to combine SPECT imaging of the I-123–labeled fatty acid analog beta-methyl-p-[(123)I]-iodophenyl-pentadecanoic acid (BMIPP) with either tetrofosmin or MIBI to evaluate the relationship between myocardial perfusion (MIBI or tetrofosmin) and fatty acid metabolism. The pattern of reduced BMIPP uptake vis-á-vis myocardial perfusion in small series has shown moderate (~70%–75%) predictive accuracy for recovery of contractile function following coronary revascularization.26,27 Additional large-scale clinical trials will be required to determine the true value of this approach for identification of myocardial viability in regions with contractile dysfunction and their recovery following revascularization.
PET (FDG/NH3, C-11 Acetate-MVO2, Fatty Acid Tracers)
The established “gold standard” for assessment of myocardial viability has been the PET FDG/myocardial perfusion study (N-13 ammonia or O-15 water). The technique was originally described by Schelbert et al.28 and is currently employed by many PET laboratories around the world.7,29,30,31,32,33,34
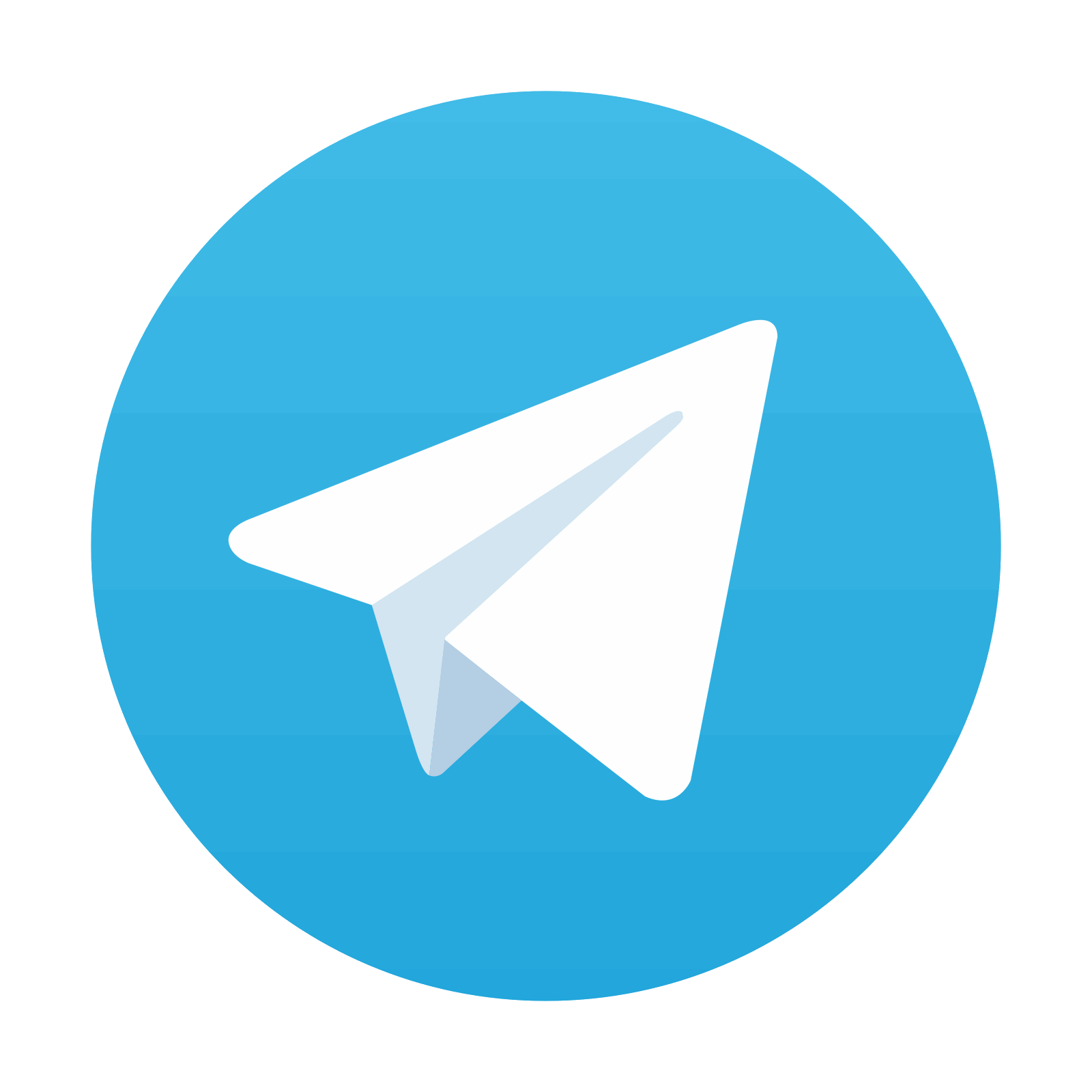
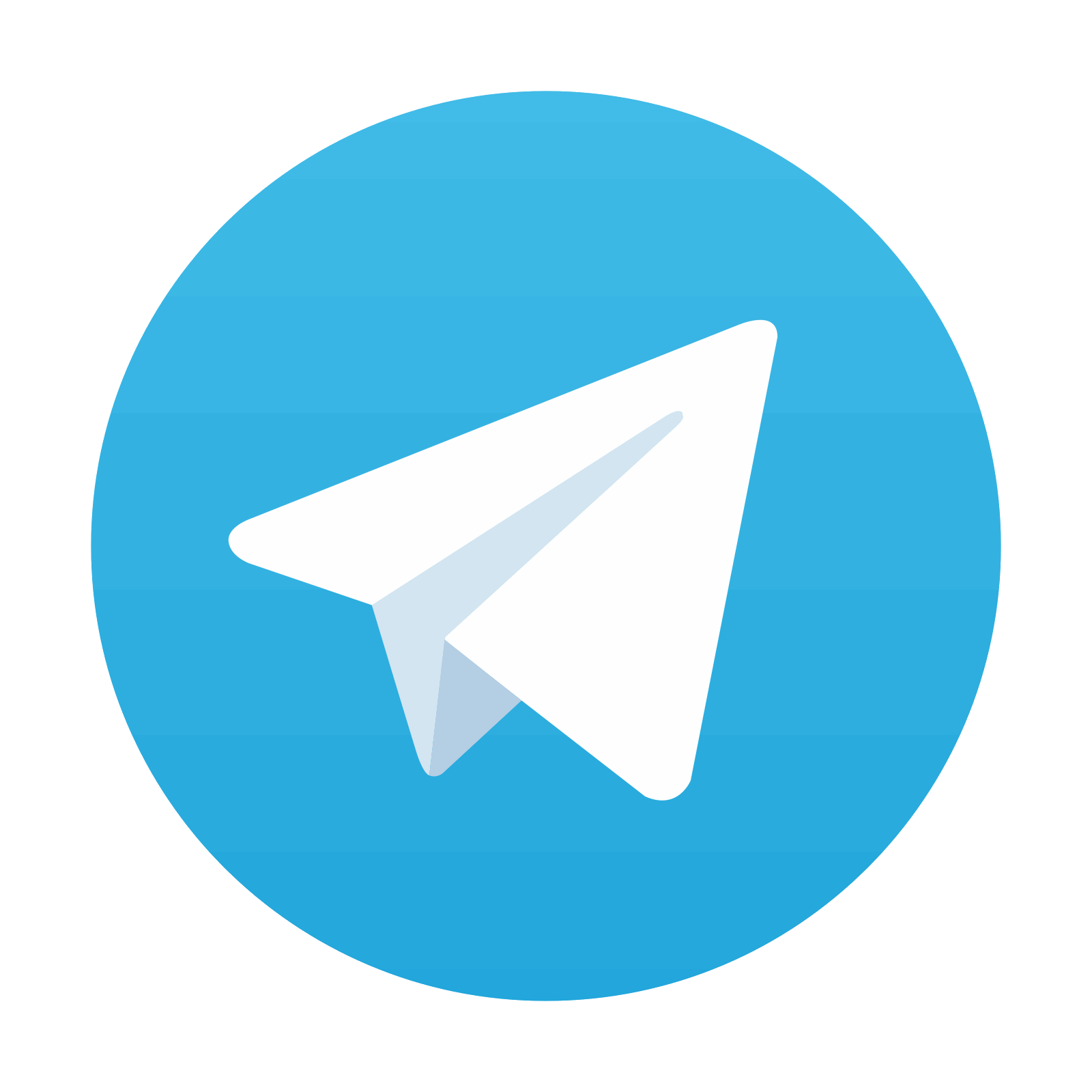
Stay updated, free articles. Join our Telegram channel

Full access? Get Clinical Tree
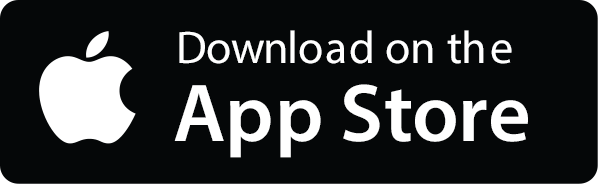
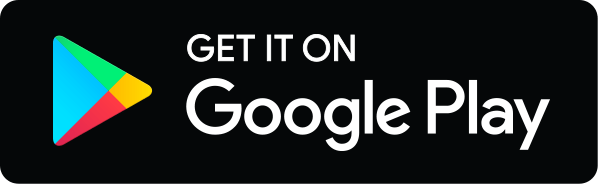
