Cardiac MR imaging and pulmonary MR angiography (MRA) are important clinical tools for the assessment of pulmonary vascular diseases. There are evolving noncontrast and contrast-enhanced techniques to evaluate pulmonary vasculature. Pulmonary MRA is a feasible imaging alternative to CTA in pulmonary embolism detection. Perfusion MR imaging and cardiac MR imaging help diagnose and monitor the treatment response of chronic thromboembolic pulmonary hypertension. Cardiac MR imaging is pivotal in assessing the potential underlying etiology and impact of pulmonary hypertension on the heart. Multiphasic acquisitions and dynamic phase imaging are unique to pulmonary MRA, which aid in diagnosing many pulmonary vascular diseases, including shunts and masses.
Key points
- •
Pulmonary MR angiography (MRA) is a feasible imaging alternative to computed tomography angiography (CTA) in pulmonary embolism detection.
- •
Perfusion MR imaging and cardiac MR imaging help diagnose and monitor the treatment response of chronic thromboembolic pulmonary hypertension.
- •
Cardiac MR imaging is pivotal in assessing the potential underlying etiology and impact of pulmonary hypertension on the heart by providing reference-standard noninvasive cardiac morphology and function assessment and tissue characterization.
- •
Multiphasic acquisitions and dynamic phase imaging are unique to pulmonary MRA, which aid in diagnosing many pulmonary vascular diseases, including shunts and masses.
Video content accompanies this article at http://www.radiologic.theclinics.com .
Introduction
Pulmonary vascular diseases are an important cause of patient morbidity and mortality. Acute pulmonary embolism affects 1 in 1000 people annually and is the third most common cause of acute cardiovascular mortality. , Other pulmonary artery diseases include pulmonary hypertension, vasculitis, aneurysms, congenital diseases, arteriovenous malformations, shunts, and masses. Computed tomographic (CT) pulmonary artery angiography is the reference standard for evaluating pulmonary vasculature with excellent resolution, wide availability, and fast acquisition. Historically, MR pulmonary angiography had limited clinical use in evaluating pulmonary vascular disease. The limitations included low resolution and artifacts from slow flow, respiratory, and cardiac motion; resulting in low sensitivity and specificity. , However, MR pulmonary angiography has become a promising diagnostic tool with newly evolving techniques, wider availability, and faster acquisitions. In patients with moderate-to-severe iodinated contrast allergy and renal failure, MR angiography (MRA) may be a feasible diagnostic alternative in pulmonary embolism detection. In 2022, coronavirus disease 2019-related lockdowns resulted in a worldwide iodinated contrast media shortage where some centers used MR pulmonary angiography as a substitute for CTA to evaluate for pulmonary embolism.
Furthermore, lung perfusion MR imaging is a unique technique for perfusion assessment without the requirement for iodinated contrast or radiation exposure. Cardiac MR imaging is the noninvasive reference standard imaging tool to assess cardiac morphology and function. It has a pivotal role in managing patients with pulmonary hypertension or other pulmonary vascular diseases. Thus, when used alone or combined, MR pulmonary angiography, cardiac MR imaging, and perfusion MR imaging are essential in managing patients with pulmonary vascular disease. , This study reviews technique, limitations, and clinical applications of cardiovascular MR imaging and MRA in patients with pulmonary vascular disease.
Imaging technique
Noncontrast MR Pulmonary Angiography
Balanced steady-state gradient echo sequence
The balanced steady-state free precession (bSSFP) sequences have been commercially available for over 20 years. The steady-state free precession (SSFP) sequence provides high contrast of the blood pool with minimal flow dependency. SSFP sequences obtained at axial, coronal, and sagittal planes may allow evaluation of central pulmonary embolism in fewer than 5 minutes of acquisition. Heredia and colleagues demonstrated that SSFP imaging provides a good image quality in all central and lobar pulmonary artery branches and 90% of segmental pulmonary arteries. Kaya and colleagues demonstrated that the sensitivity of pulmonary embolism detection was 87.9% with SSFP sequences. Unlike spin echo sequences, SSFP is more susceptible to the B0 magnetic field inhomogeneities, resulting in off-resonance banding artifacts and low imaging quality of peripheral branches. Thus, the SSFP sequences should be obtained when combined with subsequent contrast-enhanced sequences to confirm or increase the sensitivity and specificity of pulmonary embolism detection.
A navigator (NAV)-gated 3 dimensional (3D) SSFP imaging can provide rapid 3D imaging with free breathing and take approximately 3 minutes to obtain. Hui and colleagues showed that the NAV-gated SSFP images are comparable to the breath-hold SSFP sequences.
Three dimensional fresh blood imaging using 3 dimensional fast spin echo-based sequence
The 3D fast spin echo (FSE)-based sequence could provide 3D non-enhanced MRA or MR venography (MRV) imaging using electrocardiogram (ECG) gating. The sequence depends on differences in the blood flow during systole and diastole of the cardiac cycle. ECG-triggered imaging during diastole provides signals from arterial structures that have a relatively slow flow. MR imaging of the venous structures could be obtained similarly due to their constant slow flow. The images are moderate-to-heavily T2 weighted with this sequence.
Contrast-enhanced MR Pulmonary Angiography
High-resolution 3 dimensional T1-weighted spoiled gradient echo
Contrast-enhanced MR angiography is the most accurate MRA technique in evaluating pulmonary arteries. In this technique, the gadolinium-based contrast agent results in T1 shortening and high signal of the vasculature. The images are obtained with a very short relaxation time (TR) of less than 5 msec and an echo time (TE) of less than 2 msec; using a fast low-angle single shot (FLASH) technique. A short TE prevents signal loss from inhomogeneous magnetic susceptibility from background lung parenchyma and reduces artifacts. A short TR enables imaging with shorter breath holds. The acquisition time can be further decreased by parallel imaging. The images are usually acquired in coronal plane to reduce the required number of slices for coverage. The arms-up position prevents wrap-around artifacts. ,
The gadolinium-based contrast media is injected via a peripheral vein as a bolus with an automated power injector. A standard dose of gadolinium-based contrast (0.1 mmol/kg) is used with a flow rate of 2 to 5 mL/s to obtain an optimal bolus, which is followed by a 20 mL saline flush. Acquisition at the optimal timing during the maximized pulmonary vascular signal intensity is critical. The contrast media concentration is adjusted to the central k-space acquisition timing. Nonoptimized imaging may preclude pulmonary arterial assessment due to suboptimal opacification of the pulmonary artery branches and opacification of the pulmonary veins. The timing could be determined by care bolus/MR fluoroscopy technique or test bolus examination. A series of delayed phase images can often be helpful in problem-solving, especially for bolus timing-related suboptimal opacification or artifacts.
Time-resolved MR Angiography
Time-resolved MRA (TR-MRA) provides rapid multiphasic imaging of the pulmonary vasculature, which benefits patients with severe dyspnea. With parallel imaging, the MRA images could be obtained in less than 10 seconds with a temporal resolution of 1 second. , Optimized bolus timing is not required as injection and image acquisition occur simultaneously. Furthermore, pulmonary venous and aortic imaging could be obtained during the same session. TR-MRA is used to evaluate pulmonary venous anatomy for pre-procedural assessment of atrial fibrillation ablation, vascular shunts, arteriovenous malformation, and vascular anomalies. During TR-MRA acquisition, there is relatively more frequent sampling of the center of the k-space than the periphery. , Thus, although the imaging has good contrast resolution, the evaluation of the peripheral branches may be degraded due to low spatial resolution. A standard dose of gadolinium-based contrast (0.1 mL/kg) should be used with a high flow rate to obtain an optimal bolus.
Pitfalls, limitations, artifacts, and how to solve them
Pulmonary MR imaging is difficult due to the heavily T2 ∗ -weighted signal of air (0.5 msec T2 ∗ at 1.5 T and 2 msec T2 ∗ at 3T). Increased susceptibility limits the delineation of small peripheral vessels. These effects could be decreased by using a 1.5 T scanner, employing short TE GRE sequences, scanning during expiration, and parallel imaging.
Maki artifact occurs due to inadequate timing of the contrast bolus relative to k-space sampling. If the contrast bolus arrives in the middle of the acquisition, the center of the k-space is obtained with minimal contrast in the pulmonary arteries, and the edges are obtained when contrast is present. This results in transient enhancement of the periphery of the vessel and signal drop of the center. The artifact could be prevented by diluting the intravenous contrast and ensuring diluted contrast administration throughout the acquisition. Obtaining a delayed phase in addition to the arterial phase can help distinguish artifact from a true embolism ( Fig. 1 A–C ).
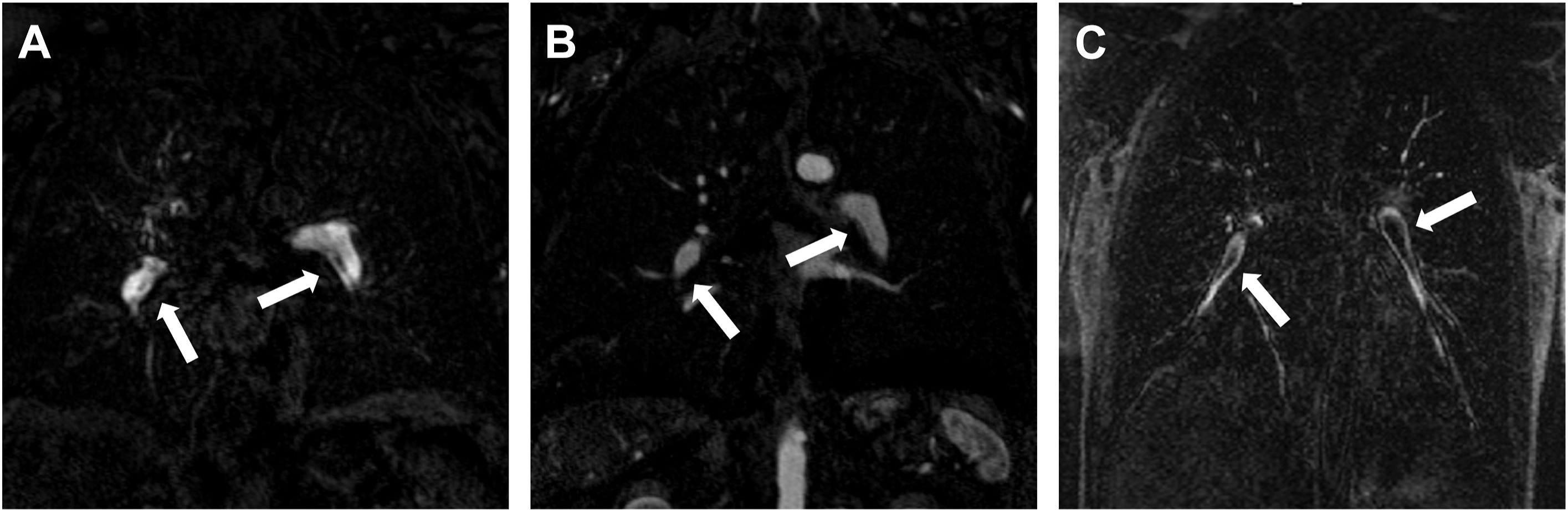
Truncation artifact (Gibbs or ringing artifact) is seen near the abrupt transitions between regions of high and low signal intensity. The artifact results in central low signal intensity in pulmonary arteries 3 to 5 pixels in diameter, such as lobar or segmental pulmonary artery branches. Identification of this artifact is usually straightforward with experienced readers; however, it may be difficult for inexperienced radiologists. Bannas and colleagues demonstrated that a signal drop with a cut-off value greater than 51% on the arterial phase and >47% on the delayed phase could distinguish artifact from pulmonary embolism with high sensitivity (100%) and specificity (>90%).
Additional MR imaging artifacts, such as motion and wrap around (aliasing), can occur. Careful selection of patients who can hold their breath is essential to avoid motion artifacts with pulmonary MRA. Having the patient raise their arms can avoid the wrap-around artifacts. The aliasing artifacts can be avoided by increasing the field of view, although this results in a longer breath-hold acquisition.
Additional techniques
Ferumoxytol as a Contrast Agent
Ferumoxytol (Feraheme, AMAG) is an ultrasmall superparamagnetic iron oxide particle approved by Food and Drug Administration for parenteral iron deficiency anemia treatment. It causes T1 shortening and can be an alternative MR contrast agent in patients with moderate-to-severe gadolinium contrast allergy. It has a relatively prolonged intravascular half-life of 15 hours, allowing repeat imaging in technically inadequate studies and same-session lower extremity MRV, as needed. The dose for MRA (3–4 mg/kg) is lower than for anemia treatment and requires a slow infusion rather than rapid bolus administration as it may cause side effects such as hypotension and death. , The acquisition time is short and requires a few breath holds. The limitations of ferumoxytol are longer preparation time, requirement for slow infusion and monitoring after the infusion, transient signal alteration on subsequent MR imaging studies, and relatively high cost. Starekova and colleagues. showed that ferumoxytol-enhanced MRA provided a good or excellent image quality MR pulmonary angiography in pregnant patients in most cases.
MR Perfusion
Noncontrast MR pulmonary perfusion
Fourier decomposition techniques provide ventilation and perfusion images using differences in signal changes during inspiration and exhalation and cardiac systole and diastole. Arterial spin labeling provides perfusion maps by labeling the intrinsic contrast of magnetized inflowing flow. Currently, these methods do not have any clinical application due to artifacts, low spatial resolution, and low signal-to-noise ratio.
Contrast-enhanced MR pulmonary perfusion
Dynamic time-resolved MR sequence is obtained with subtracted first-pass dynamic postcontrast T1-weighted imaging with high temporal resolution. A lower quantity of gadolinium contrast such as 0.05 to 0.1 mmol/kg is used. Perfusion imaging has higher temporal but lower spatial resolution than MR angiography. The perfusion images could be obtained with breath holds or free breathing. A 3D FLASH MR imaging is frequently employed. The perfusion abnormalities identified with this technique can be qualitatively and quantitatively assessed.
Although the perfusion MR imaging technique has been studied for more than 20 years and significant advancements have been made, the clinical translation of these improvements has been relatively slow. This is primarily due to high feasibility of other studies such as single-photon emission computed tomography (SPECT) and spectral CT. However, MR imaging may have a potential role in pregnant and pediatric patients and patients requiring serial imaging. Dynamic contrast-enhanced lung perfusion MR imaging has similar sensitivity and specificity to SPECT and planar scintigraphy for screening for chronic thromboembolic pulmonary hypertension. ,
Imaging protocol
In our institution, MR pulmonary angiography examinations are performed using at least 1.5 T field magnetic strength. The chest imaging begins with axial and coronal 2 dimensional (2D) bSSFP and axial and coronal fat-saturated T1-weighted spoiled gradient echo sequences. Subsequently, an initial mask of a high-resolution (HR) 3D T1 gradient echo data set is acquired during breath hold at end expiration. Before contrast administration, the technologists check the images for wrap-around artifacts and coach the patient on breath-hold instructions. A single dose of gadolinium-based contrast (0.1 mmol/kg) is prepared and diluted with saline at a 1:1 ratio. Coronal TR-MRA is acquired with a temporal resolution of 2 seconds, which begins immediately before the contrast injection. Initially, 5 to 8 mL of the diluted contrast (⁓25%–30% of the total contrast amount) is administered at a rate of 2 mL/sec. Subsequently, the remainder of the contrast is injected at a rate of 2 mL/sec, and the coronal HR MRA images are acquired, which is timed to pulmonary arteries. After HR MRA images, a coronal whole-heart 3D Nav inversion revocery (IR) cardiac-gated and respiratory-gated images are obtained. Then, postcontrast axial and coronal fat-saturated T1-weighted spoiled gradient echo sequences are acquired ( Table 1 ). Total table time is 30 to 45 minutes. Post-processing is performed at the scanner, which includes maximum intensity project images and axial-plane multiplanar reformation of the HR-MRA, 3D Nav-IR MRA, and TR-MRA (during the maximum opacification of the pulmonary artery) and images are sent to picture archiving and communication system. It is possible to create a significantly abbreviated protocol to allow for more rapid evaluation for acute pulmonary embolism. In this setting, an HR-MRA can be timed to the pulmonary artery phase of enhancement, followed by 2 to 3 additional delayed-phased HR-MRA acquisitions ( Table 2 ).
Preliminary Imaging | Three-plane Localizer Scout Imaging |
Precontrast Sequences in Order | Axial bSSFP single-shot, breath hold |
Coronal bSSFP single-shot, breath hold | |
Axial fat-saturated T1W spoiled GRE, breath hold | |
Coronal fat-saturated T1W spoiled GRE, breath hold | |
Coronal high-resolution precontrast 3D T1W GRE MRA, breath hold | |
Contrast Agent Injection | Intravenous single dose of gadolinium-based contrast 0.1 mmol/kg which is diluted with saline at 1:1 ratio |
Postcontrast Sequences in Order | Coronal time-resolved MRA (temporal resolution of 2 s), 5–8 mL bolus at 2 mL/sec rate, scan starts immediately prior to injection |
Coronal high-resolution 3D T1W GRE MRA, breath hold, injection of the remainder of contrast at 2 mL/sec, timed to pulmonary artery | |
Coronal whole heart 3D Navigator IR cardiac and respiratory gated | |
Axial fat-saturated T1W spoiled GRE, breath hold | |
Coronal fat-saturated T1W spoiled GRE, breath hold |
Preliminary Imaging | Three-Plane Localizer Scout Imaging |
Precontrast Sequence | Coronal precontrast 3D T1W spoiled GRE MRA, breath hold |
Contrast Agent Injection | Intravenous single dose of gadolinium-based contrast that is diluted with saline to a total volume of 30 mL and injected at 1.5 mL/s rate |
Postcontrast Sequences in Order | Coronal 3D T1W spoiled GRE MRA, breath hold, timed to pulmonary artery |
Coronal 3D T1W spoiled GRE MRA, breath hold, immediate delay | |
Low flip angle T1W 3D spoiled GRE MRA | |
T1W 2D axial or 3D spoiled GRE with fat saturation |
Imaging findings in pulmonary embolism
The imaging findings of pulmonary embolism with MRA are similar to those observed with CTA. Direct findings include filling defects, T1 hyperintense clot in the pulmonary arteries, occlusion of the pulmonary artery, and arterial cut-off sign ( Figs. 2 A–E and 3 A–C ). Indirect signs include main pulmonary artery enlargement, dilated right heart chambers, peripheral wedge-shaped intensities representing pulmonary infarcts, pleural effusion, atelectasis, and perfusion defects on MR imaging perfusion. ,
