The investigation and application of PET modalities for the evaluation and treatment of patients with central nervous system (CNS) tumors continues to evolve, with anticipated increased uptake in the United States for both benign and malignant CNS tumors in the decade to come.
Key points
- •
PET is increasingly used for brain tumors to aid in diagnosis, prognostication, evaluation of extent of disease, treatment planning, and response assessment.
- •
Somatostatin receptor PET has established a role in assessment and treatment planning for meningioma, while amino acid PET continues to be evaluated for glioma.
- •
Histology-specific PET tracers may help guide care of patients with brain metastases, while other tracers may help differentiate progressive tumor from necrosis and treatment effects.
CNS | central nervous system |
DCE | dynamic contrast-enhanced |
DW | diffusion weighted |
FDG | fluorodeoxyglucose |
FLAIR | fluid-attenuated inversion recovery |
GBM | glioblastoma |
GTR | gross total resection |
MTV | metabolic tumor volume |
PCNSL | primary CNS lymphoma |
PFS | progression-free survival |
RANO | Response Assessment in Neuro-Oncology |
SSTR | somatostatin receptor |
SUV max | maximum standardized uptake value |
TLG | total lesion glycolysis |
Introduction
The role of PET imaging in the management of patients with central nervous system (CNS) tumors has reached a promising juncture. Beyond its diagnostic utility, PET imaging is emerging as a tool to guide multidisciplinary management and next-generation precision radiotherapy. Its prognostic and predictive capabilities have the potential to inform the timing, sequencing, and intensification of local therapy platforms across benign and malignant CNS tumors. Increased utilization of PET imaging for CNS tumors in the United States in both routine care and clinical trials attests to its growing role in neuro-oncology, across the spectrum of diagnosis, treatment, and surveillance.
In this review, we overview the diagnostic roles of PET modalities for CNS tumors, and the evidence of its prognostic and predictive significance among the most common as well as rarer malignant and benign CNS tumors. We compare PET modalities with MR imaging in identifying and treating biologically relevant tumor in glioblastoma (GBM), the most aggressive primary CNS malignancy, as well as recent clinical trials assessing the role of PET as an integral imaging biomarker to guide patient-specific radiotherapy. We review its potential role in enhancing response assessment to multimodality therapy and to guide earlier and more accurate decision-making regarding adjuvant or salvage treatment of patients with CNS tumors. Finally, we discuss promising future directions of research aimed at maximizing the potential applications for advanced PET modalities in the multidisciplinary care of the neuro-oncology patient.
Diagnostic role of PET/CT
PET imaging of brain tumors has been an active area of research for nearly 50 years. Despite this fact, PET imaging is only now beginning to be regularly used for brain tumor assessment in the United States. In coming years, it is anticipated that PET imaging will continue to find greater use in a wide variety of brain tumors, including gliomas, meningiomas, and intracranial metastatic disease.
Fluorodeoxyglucose (FDG) is the prototypical PET agent and is widely used both for CNS imaging of benign processes such as neurodegenerative disease and epilepsy, as well as for a broad range of extracranial neoplasms. However, FDG is a suboptimal imaging agent for the evaluation of brain tumors given a high degree of background glucose uptake in normal brain, limited sensitivity for tumor identification, and poor specificity to differentiate tumors from other disease processes. A variety of techniques to optimize brain tumor evaluation by FDG PET have been explored, such as multi-timepoint delayed imaging, but the question of whether FDG PET has any useful role in brain tumor imaging remains open to debate.
The primary innovation that has brought PET imaging of brain tumors to the forefront is the development of non-FDG PET radiotracers that offer greater sensitivity, specificity, and higher tumor-to-background ratios. Amino acid PET agents are the class currently of greatest interest for the evaluation of infiltrating gliomas and metastatic disease. In the context of glioma, these agents are able to cross the blood brain barrier and identify nonenhancing disease, leading to a range of potential uses from initial diagnosis to treatment planning to longitudinal response assessment. Amino acid PET is commonly used for these purposes in European centers, but lack of regulatory approval in the United States has necessarily limited the tool to research applications, though this circumstance may change in the near future. With respect to meningiomas, PET radiotracers targeting somatostatin receptors can detect meningioma tissue with high sensitivity and specificity. In many centers, off label use of somatostatin receptor PET is frequently employed for surgical and radiation planning. , The PET imaging agents of greatest interest in brain tumor imaging are summarized in Table 1 . A more complete account of emerging radiotracers of interest in neuro-oncology is beyond the scope of this review and has been previously published.
Tumor Use | Agent | Mechanism | FDA Approved a | Half-Life |
---|---|---|---|---|
Meningioma | 68 Ga-DOTATATE | Somatostatin receptor binding | X | 1.1 h |
68 Ga-DOTATOC | Somatostatin receptor binding | X | 1.1 h | |
64 Cu-DOTATATE | Somatostatin receptor binding | X | 12.7 h | |
Glioma | O -(2-[ 18 F]fluoroethyl)-L-tyrosine (FET) | LAT1 and LAT2 amino acid transporter uptake | 1.8 h | |
3,4-dihydroxy-6-[ 18 F]fluoro-L-phenylalanine (FDOPA) | LAT1 and LAT2 amino acid transporter uptake | X | 1.8 h | |
11 C-methyl-methionine (MET) | LAT1 and LAT2 amino acid transporter uptake | 0.3 h | ||
18 F-fluciclovine | LAT1 and ASCT1 amino acid transporter uptake | X | 1.8 h | |
18 F-fluorodeoxyglucose (FDG) | Glucose transporter uptake | X | 1.8 h |
Prognostic and predictive role of PET/CT, and evaluation of disease extent
Gliomas
Well-established predictors of outcomes for patients with glioma include tumor histology, molecular tumor characteristics, and patient characteristics. , More recently, the volume of tumor as measured by PET imaging has demonstrated prognostic value. , For patients with high-grade glioma, studies of directed biopsy and surgical resection demonstrate tumor extension outside of visualized regions on anatomic T1-weighted contrast-enhanced MR imaging. The biologic relevance of this disease is evidenced by systematic patterns of failure analyses demonstrating that inadequate resection or radiotherapy coverage of the “biologic” or “metabolic” tumor volume among patients treated using only standard of care anatomic MR imaging is not only associated with worse progression-free survival (PFS) but also corresponds spatially to where tumors eventually recur. , In a phase I/II study utilizing anatomic MR imaging to dose-intensify radiation therapy, correlative 11C-methionine PET (11C-MET PET) in 26 patients identified 19 who had greater than 1 cc of increased 11C-MET PET activity prior to radiation. Inadequate coverage of disease identified by 11C-MET PET was associated with noncentral tumor progression ( P <.01). These studies form the basis for using PET as integral imaging biomarker to guide personalized radiation treatment (see section on tumor target delineation).
Meningiomas
MR imaging with gadolinium contrast is the current standard for evaluating meningioma disease extent. , However, challenging locations (eg, sagittal sinus, parafalcine, or skull base), bony involvement, or ambiguous postoperative changes can complicate recognition of tumor extent using anatomic imaging alone. Because somatostatin receptor (SSTR) expression is many magnitudes higher in meningioma than normal brain or other brain tumors, SSTR ligands such as 68 Ga-DOTATATE and 68 Ga-DOTATOC are highly sensitive and specific for meningioma, and can aid with tumor recognition. , , The false positive gross total resection (GTR) rate as assessed by intraoperative and MR imaging evaluation alone may reach 20%, suggesting the importance of detecting a subtotal resection upfront ( Fig. 1 ).
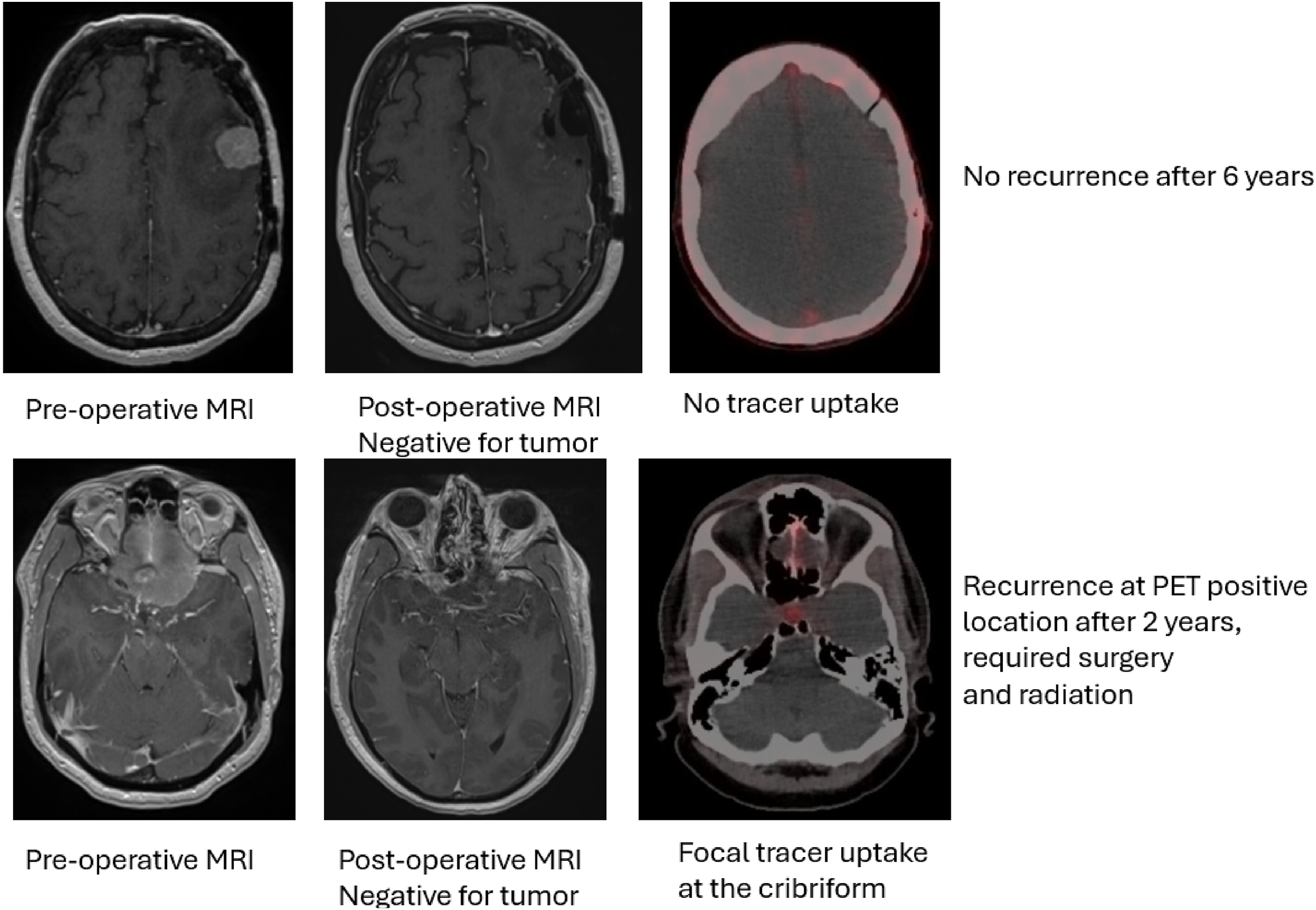
Somatostatin receptors 1 and 2 are expressed at significantly higher levels in brain tumors such as meningioma in comparison to normal tissue. , MR imaging scans are usually fused to radiation simulation scans to assist with target delineation. In many instances, the primary target of both primary and recurrent meningiomas can be difficult to delineate due to residual scar tissue or tumor infiltration, even with MR imaging. Utilizing a combined PET and MR imaging technique to assess tumors may improve treatment planning in these circumstances. For example, when 68 Ga-DOTATOC-PET-fused radiation volumes were compared with volumes planned solely using MR imaging or CT, significant alterations in target volume were made in 3-quarters of plans.
There is a strong positive correlation between maximum standardized uptake value (SUV max ) of SSTR2 radiotracer 68 Ga-DOTATATE and SSTR2 expression in both primary and recurrent meningiomas, highlighting its utility in tumor detection even in patients with complex anatomic changes due to previous treatment. , 68 Ga-DOTATATE PET scans are more effective in detecting osseous involvement than MR imaging. 68 Ga-DOTATATE PET can even be used to screen for metastatic meningioma. 68 Ga-DOTATATE PET has a potential benefit for improving local control and PFS for patients diagnosed with an intermediate or high-risk meningioma due its potential efficacy in assisting radiation oncologists with more precise target delineation, which has yet to be evaluated and validated in prospective clinical trials. A retrospective study evaluated deidentified and blinded gross tumor volume contour delineation with 7 CNS specialists (4 CNS radiation oncologists and 3 neuroradiologists) for 25 patients diagnosed with a meningioma who received both a 68 Ga-DOTATATE PET and an MR imaging for radiation treatment planning. Both the MR imaging and the PET were nonsequentially contoured by each physician for each patient. The median MR imaging volume for each physician ranged from 16.94 to 25.53 cc. The median PET volume for each physician ranged from 2.09 to 8.36 cc. There were greater levels of physician agreement between PET-based contours compared with MR imaging-based contours. In this study, 7 of 25 (28%) patients had new nonadjacent areas contoured on PET by at least 6 of the 7 physicians that were not contoured by these physicians on the corresponding MR imaging. In addition, PET-based plans had dose reductions to organs at risk including the brain, brainstem, and optic structures when comparing PET-based plans to the MR imaging-based plans. Given the current evidence for Ga-DOTATATE PET imaging to differentiate meningioma tissue from nonmeningioma tissue, it has been added to the National Comprehensive Caner Network (NCCN) guidelines for meningioma.
Brain Metastases
PET imaging is not routinely used for diagnosis or radiation treatment given higher spatial resolution of MR imaging required for treatment with stereotactic radiosurgery for most patients. However, there are data demonstrating dural-based brain metastasis can be differentiated from meningiomas for prostate cancer using prostate-specific membrane antigen (PSMA)-PET and breast cancer using 16α-[18F]fluoro-17β-estradiol ([18F]-FES) PET imaging. , PET imaging has developed a more prominent role in the differentiation of radiation necrosis from tumor progression (see response assessment in brain metastases section). ,
Primary Central Nervous System Lymphoma
In contrast to other intracranial tumors, FDG PET may provide more value in assessment of primary CNS lymphoma (PCNSL). Over 90% of PCNSL demonstrates diffuse large B-cell lymphoma histology which has high FDG uptake. Furthermore, CNS lymphoma commonly involves periventricular white matter and other deep brain structures that have less physiologic glucose uptake than cortical regions. In the contexts of pretreatment PET and PET during ibrutinib therapy, significant associations have been demonstrated between increased metabolic tumor volume (MTV), total lesion glycolysis (TLG), and SUV max with overall survival and PFS in CNS lymphoma patients. , It should be noted that while measures such as MTV and TLG provide the promise of quantitative and reproducible measure of lesions, at many centers these values must still be calculated manually and are therefore not typically reported in routine clinical practice, thereby limiting their value. Still, FDG-PET appears to carry more prognostic and potentially predictor power in CNS lymphoma than other intracranial tumors.
The optimal role of FDG PET for radiation target delineation for CNS lymphoma is not defined. While a fused PET scan could serve as complementary data in defining targets, gadolinium contrast-enhancement will likely remain the mainstay for definition of gross tumor in the setting of focal treatment or tumor boost. Future molecularly targeted PET tracers, such as those targeting CD20, may provide additional value in assessment and radiation planning for CNS lymphoma. ,
Role of PET/CT in guiding radiation treatment for glioma
The implementation of advanced PET imaging for tumor targeting with radiation therapy among patients with newly diagnosed GBM has been successfully undertaken in recent phase I and II clinical trials. Across these studies, a consistent finding is the extension of disease outside of tumor identified using anatomic MR imaging alone. In studies incorporating advanced MR imaging techniques such as perfusion and diffusion MR imaging, the advanced PET and advanced MR imaging are complementary, identifying both overlapping as well as disparate tumor regions, potentially reflective of the underlying biologic and resultantly, phenotypic heterogeneity of the disease. In a recent prospective phase II trial of patients with newly diagnosed GBM targeting adversely prognostic hyperperfused and hypercellular tumor regions using dynamic contrast-enhanced (DCE) and high b-value diffusion weighted (DW) MR imaging, median MTV was 7 cc (IQR 1–18). Targeting disease identified with advanced perfusion and diffusion MR imaging also targeted a significant component of disease identified using 11C-MET PET imaging (median fraction receiving 95% of the boost radiation dose was 93% [IQR 59–100]), with a broad range of dose coverage reflective of the PET-identified tumor regions outside of that observed using even advanced MR imaging techniques.
A single institution, single-arm prospective phase II trial utilize 18F-DOPA PET to guide treatment intensification for glioblastoma met its primary endpoint of improving PFS for patients with O 6 -methylguanine-DNA methyltransferase (MGMT) unmethylated tumors and also demonstrated an overall survival benefit in patients with MGMT methylated tumors. A recently published clinical trial using 18F-DOPA PET to guide hypofractionated radiation for elderly patients met its primary endpoint of improving overall survival compared with historical controls. Another prospective study utilized 18-fluoride-fluoro-ethyl-tyrosine (FET) PET to guide radiation intensification, also demonstrating promising outcomes with a median survival of 24 months. Larger, randomized trials are needed to more definitely prove the benefit of adding amino acid PET to standard MR imaging for radiation treatment planning for glioblastoma.
Role of PET for glioma response assessment in neuro-oncology
Post-treatment surveillance for patients with GBM relies on radiographic response assessment using conventional, anatomic imaging techniques. The updated Response Assessment in Neuro-Oncology (RANO) criteria have been widely used in contemporary clinical trials to enable comparison of outcomes between studies. These criteria rely on conventional T1-weighted contrast enhanced and T2-weighted/fluid-attenuated inversion recovery (FLAIR) MR images, and specifically recognize the limitations of using this response assessment strategy for patients within 3 months after completing chemoradiation, because of the nonspecific increases in contrast enhancement unrelated to tumor status (inflammation, seizures, postsurgical changes, ischemia, subacute radiation effects) seen during this period. While numerous response assessment strategies based on anatomic, conventional MR imaging have been evaluated, including subtraction maps and volumetric measurements, no strategy has been shown to reliably evaluate early tumor response in standard practice. Furthermore, none of these response assessment criteria have been validated as predictive of survival in patients with GBM, and are therefore not utilized in routine clinical care.
Although not incorporated in the RANO criteria, a number of studies have demonstrated the potential utility of advanced PET techniques for early response assessment after chemoradiation. , Many have sought to distinguish true tumor recurrence from pseudoprogression (transient increases in contrast enhancement after chemoradiation which eventually subsides without change in cancer-directed therapy), which is a perennial challenge among a significant subset of patients and is the subject of an ongoing cooperative group trial. The recently launched GABLE study (A Phase II Glioblastoma Accelerated Biomarkers Learning Environment Trial) seeks to evaluate imaging biomarkers, including 18F-Fluciclovine PET, that may effectively help stratify patients with distinct survival outcomes who exhibit nonspecific enhancement on standard anatomic MR imaging within 3 months post-radiaition therapy (RT). To date, this is the first multi-institutional cooperative study to systematically undertake imaging biomarker evaluations with this approach.
Several studies have specifically assessed whether early tumor response itself may be identified using advanced imaging including PET techniques, and whether this response is associated with survival and/or tumor progression. In an analysis of 37 patients enrolled on a phase I/II trial assessing the safety and benefit of dose-escalated chemoradiation using anatomic MR imaging, 25 patients underwent correlative 11C-MET scans prior to and 3 months postchemoradiation. Patients with no residual MTV at 3 months had superior PFS (18.2 vs 10.2 months, P =.03). On multivariate analysis, larger 3-month MTV (hazard ratio [HR] 2.4, 95% confidence interval [CI] 1.4–4.3, P =.03) was significantly associated with worse PFS, and the enhancing tumor volume at recurrence encompassed 97% of the MTV that persisted from prechemoradiation to 3 months postchemoradiation. In another prospective study, 25 patients with glioblastoma underwent serial 18F-FET PET after surgery, 7 to 10 days (early) postchemoradiation, and 6 to 8 weeks later. Early decrease in both maximum and mean tumor-to-brain ratios was significantly associated with both PFS and overall survival (OS), whereas changes on anatomic contrast-enhanced MR imaging were not. Recent guidelines codifying PET-based response (PET RANO 1.0) in diffuse gliomas may now enable the structured implementation of PET-based imaging into neuro-oncology clinical research, and provide a uniform language that will enable consistency and comparability of future investigations.
Response assessment for brain metastases
A significant challenge in the management of patients with brain metastasis is the differentiation of adverse radiation effects and true tumor progression. Standard anatomic MR imaging and reliance on volumetric changes is inaccurate and insufficient in determining tumor progression. Advanced imaging techniques such as dynamic susceptibility contrast MR imaging, DCE MR imaging, and MR spectroscopy are additional imaging modalities that have been used to improve the sensitivity and specificity of differentiating tumor necrosis from tumor progression. , More recently, prospective trials utilizing 18F-Fluciclovine PET imaging have evaluated the diagnostic performance of this imaging agent, and its potential utility in addition to standard MR imaging in determining pseudoprogression from true progressive tumor. , An SUV max threshold of 4.8 conferred a sensitivity of 80% with a specificity of 85% (area under the curve [AUC] 0.87). Results from the multicenter phase 3 trial are awaited. A case example of a patient not treated on clinical trial for whom 18F-Fluciclovine was acquired postradiation is shown in Fig. 2 .
