Trans-arterial interventions are an increasingly utilized approach for diagnosing and treating a wide range of pathologies, providing superior patient outcomes compared to traditional open surgical methods. Recent advancements in tracking and navigation technologies have significantly refined these interventions, enhancing procedural precision and success. Advanced imaging modalities, such as fluoroscopy, cone beam computed tomography (CBCT), and intravascular ultrasound (IVUS), are frequently used strategies offering critical real-time guidance. Although fluoroscopy remains the primary choice for many procedures, advancements in CBCT and IVUS have enabled the delivery of detailed volumetric and intravascular imaging while reducing radiation exposure. Aiming to enhance imaging capabilities further, image fusion and augmented reality technologies show substantial potential for integrating multiple datasets into cohesive models, improving instrument tracking and spatial orientation during interventions. Innovations in navigation systems, including electromagnetic, fiberoptic, and robotic navigation, provide additional tracking capabilities for enhanced intra-arterial navigation. This article will review the current advancements in imaging, tracking, navigation, and image fusion technologies and explore their utility in enhancing trans-arterial interventions.
Tracking and navigation technologies for image guided trans-arterial interventions
Trans-arterial interventions in interventional radiology leverage advanced imaging technologies to effectively enhance patient outcomes and procedural success. These procedures encompass a diverse array of diagnostic and therapeutic techniques, all of which depend on precise imaging to navigate to the target site accurately. Over time, percutaneous trans-arterial interventions have increasingly supplanted both minimally invasive and traditional open surgical techniques. This shift has led to improved patient outcomes, shorter recovery times, reduced procedural complications, and more refined management of complex diseases. With the growing demand for trans-arterial interventions, there is an increasing need for even more precise tools and techniques to enhance the efficacy of these interventions.
A significant challenge in these interventions is the necessity for accurate image guidance and navigation technologies to identify and track tools within the patient’s vasculature effectively. This requires sophisticated imaging modalities and advanced technology that can provide real-time or near-real-time feedback to the operator. Technologies such as fluoroscopy, computed tomography (CT), and intravascular ultrasound (IVUS) have become widely adopted in clinical practice, each offering unique advantages and facing specific limitations. As the adoption of trans-arterial interventions grows, it has become increasingly vital to select the optimal imaging modality for each intervention and to consider the cumulative effects of ionizing radiation exposure on patients and healthcare staff.
Recent advancements have focused on the development of advanced fusion imaging techniques and more precise navigation systems designed to enhance visualization of the vascular anatomy and facilitate the accurate delivery of instruments and therapeutics to the target site. Innovations such as robotic systems and augmented reality solutions are in progress, promising to standardize procedural workflows and enhance physician capabilities. These technologies are rapidly evolving, with continual improvements in their underlying sophistication.
This review aims to provide a comprehensive overview of recent advancements in tracking and navigation technologies for trans-arterial interventions. We will evaluate the capabilities and limitations of current systems, offer insights into anticipated future developments, and highlight the benefits these technologies offer in managing increasingly complex trans-arterial procedures. Our objective is to deliver a thorough understanding of how these technologies can be integrated into clinical practice to improve patient care and expand the capabilities of interventional radiologists.
Imaging modalities
Selecting the appropriate imaging modality is critical for the success and efficacy of trans-arterial interventions. Each modality brings unique visualization capabilities and inherent limitations, impacting the effectiveness of visual guidance during complex procedures. Understanding these advantages and disadvantages is vital to optimizing procedural outcomes while minimizing the risk to the patient, physician, and operating room staff.
Fluoroscopy
Fluoroscopy remains the foundation in trans-arterial interventions, providing real-time imaging that allows dynamic visualization of catheters and guidewires within the vasculature. Its ability to deliver immediate feedback is indispensable for interventions such as angioplasty, stenting, and embolization, making it the standard of care in such procedures. However, fluoroscopy has limitations, including relying on iodinated contrast agents for adequate visualization. These agents are potentially nephrotoxic, which can be a limiting factor in patients with renal impairment. Additionally, fluoroscopy provides only 2-dimensional images, requiring frequent C-arm repositioning to gain a 3-dimensional perspective. The extended use of fluoroscopy further increases ionizing radiation exposure to both patients and staff. However, advances such as flat-panel detectors have improved image quality while reducing radiation dose.
New C-arm imaging platforms aim to provide even more significant reductions in radiation exposure while providing high-quality diagnostic imaging. The x-ray imaging platform AlluraClarity (Philips Healthcare) was shown to result in 66% less radiation exposure during transarterial chemoembolization for live cancer. This reduction was achieved while maintaining the same quality of imaging compared to their previous platform, with no statistically significant difference seen in intra-procedural images evaluated subjectively by 2 independent evaluators.
Cone beam CT (CBCT)
Cone Beam Computed Tomography (CBCT) has addressed many of the limitations inherent in traditional 2-dimensional fluoroscopy by providing detailed volumetric imaging. CBCT technology allows for the acquisition of comprehensive images with a single rotation of the C-arm, leveraging flat-panel detection systems to facilitate intra-procedural CT imaging without repositioning the patient to a conventional scanner. This allows for intra-procedural CT imaging without moving the patient, enabling a comprehensive evaluation of arterial anatomy. This ability to conduct thorough evaluations of the arterial anatomy is particularly beneficial in tumor-directed interventions, where precise visualization of feeding arteries is essential. Automated system capable of detecting tumor feeding arteries have been demonstrated to achieve greater than 90% sensitivity, 29%-50% higher than visual identification on a non-selective digital subtraction angiography (DSA) study.
Advancements in CBCT technology have enabled the creation of complex visualization data, such as 3D angiography, further enhancing its utility in the interventional suite. Modern guidance software that overlays CBCT images with live fluoroscopy provides radiologists with an enhanced anatomical roadmap, increasing the accuracy of catheter and guidewire placement. Additionally, CBCT offers the potential to reduce both radiation exposure and the need for iodinated contrast compared to traditional CT angiography.
Nevertheless, CBCT is not without its limitations. The technology still involves considerable exposure to ionizing radiation. Studies demonstrating significant reductions in radiation exposure typically see the bulk of the reductions coming from 2-dimensional studies and not CBCT acquisitions. Additionally, CBCT images are diminished by factors such as respiratory motion, which can degrade image quality. Such motion artifacts are particularly problematic when patients are under conscious sedation, as they require coordinated breath-holding to achieve optimal image clarity. In instances where patients are unable to comply with breath-holding instructions, the resultant images may be of limited diagnostic value
Angio-CT
Angio-CT marks a transformative advancement in interventional suites, traditionally limited to either CT or fluoroscopic-guided procedures. Developed in 1992 in Japan, this technology integrates multi-detector CT (MDCT) and fluoroscopy within a single suite, offering superior imaging with a wider field of view, higher resolution, reduced artifacts. This streamlined integration enhances procedural efficiency by eliminating patient transfers and optimizing workflow.In addition to its imaging benefits, Angio-CT holds significant operational and financial potential by reducing the time and resources needed for patient treatments. Its versatility supports both diagnostic CT and fluoroscopy, enabling a broad range of complex and non-complex interventions to be performed efficiently in a single session. This capability enhances operational utilization, making it particularly advantageous for interventional radiology practices managing diverse and multi-procedural cases. By optimizing workflows and consolidating procedures, Angio-CT is poised to elevate the efficiency and effectiveness of modern interventional practices. Recent innovations in Angio-CT setups now allow these tools to seamlessly integrate with CT scans performed during procedures, enabling smooth and efficient communication between the two modalities. For example, embolization guidance software enhances catheter navigation, facilitates precise selection of target vessels, and supports focused CT hepatic arteriography (CTHA) to confirm accurate vessel targeting. These advancements streamline workflows and improve procedural accuracy ( Fig. 1 ).
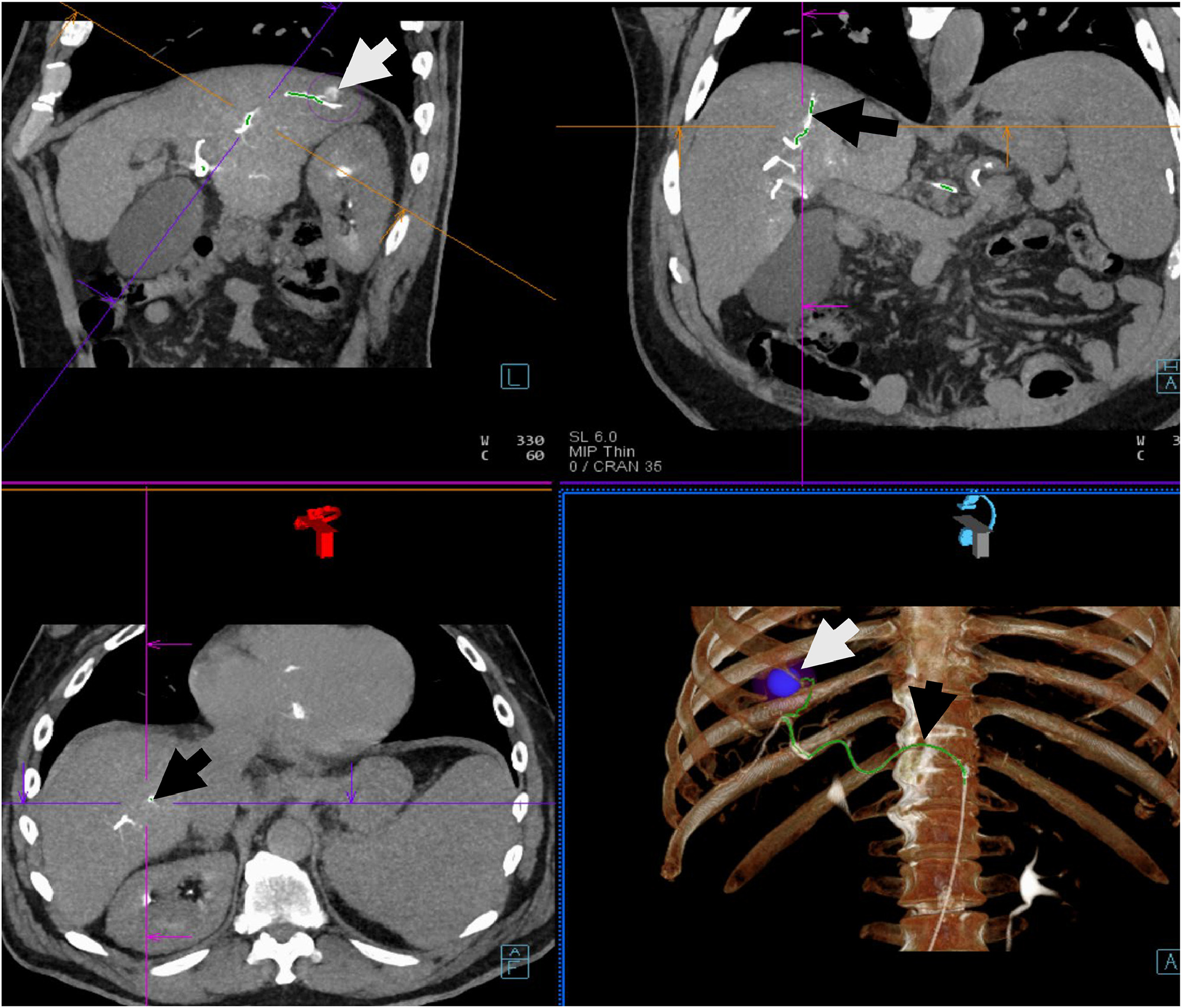
Intravascular ultrasound (IVUS)
Intravascular ultrasound (IVUS) provides a valuable radiation-free imaging modality, complementing existing technologies by offering precise imaging guidance from within the vascular system. The IVUS catheter can be introduced through an arterial sheath allowing for an intravascular view of the vessel walls and surrounding tissue. IVUS is particularly beneficial in situations where reducing radiation exposure and contrast use is a priority. IVUS has been shown to enhance the accuracy of intravascular navigation, thereby diminishing the dependence on fluoroscopy and nephrotoxic contrast agents. For arterial interventions, IVUS can be an invaluable tool for determining vessel morphology, which can assist in planning when placing arterial stents.
IVUS technology has been further advanced by incorporating image fusion techniques. For instance, the fusion of IVUS with optical coherence tomography (OCT) can provide cross-sectional views of blood vessels, enhancing image guidance in recanalization procedures. Innovations combining fiber-optic navigation (FORS) with IVUS have also demonstrated reductions in radiation and contrast use. However, despite these improvements, IVUS remains limited by its spatial resolution, and its effectiveness is highly dependent on the operator’s skill and expertise.
Navigation systems
In the realm of trans-arterial interventions, navigation systems hold significant promise by enhancing spatial orientation and enabling precise instrument tracking. These technologies can facilitate more accurate targeting of lesions and reduce procedural complications, overcoming the limitations of traditional imaging alone. By providing interventional radiologists with advanced tools, these systems allow for more efficient navigation through the complex vascular landscape. Typical tracking and navigation systems include electromagnetic navigation, optical tracking, laser guidance, magnetic guidance, and fiberoptic navigation. However, most commercially available systems are primarily designed for percutaneous needle interventions, with few options explicitly tailored for trans-arterial interventions. Despite this, ongoing advancements have improved their applicability and effectiveness in trans-arterial navigation.
Electromagnetic navigation
Electromagnetic navigation systems have emerged as a promising technology, providing enhanced guidance for trans-arterial interventions. These systems utilize electromagnetic fields to track the position and movement of endovascular instruments in real time. One of the key advantages of these systems is their ability to function without a direct line of sight—a crucial requirement for trans-arterial navigation. Several electromagnetic systems are available commercially including the StealthStation™ (Medtronic) and AxiEM™ (Medtronic).
In-vitro studies, such as those involving the StealthStation TREON plus, have demonstrated significant reductions in fluoroscopy times when electromagnetic navigation is employed, regardless of the operator’s level of expertise. However, there is a gap between in-vitro results and clinical practice; reports indicate variable procedural times when these systems are introduced into real-world settings. This may be attributable to the operators’ unfamiliarity with the technology, highlighting the learning curve associated with integrating such systems effectively.
However, initial data from studies examining the efficacy of electromagnetic navigation systems is promising. EM tracking systems alone, using no intra-procedural images, have been used to successfully position and deploy thoracic aortic endografts in swine with a deployment deviation error of less than 5 mm. This result mirrors phantom studies for endovascular abdominal aortic aneurysm repair, which resulted in a mean registration error 1.3 mm. Current clinical practice pairs electromagnetic navigation with traditional fluoroscopy. However, as this technology continues to progress the need for additional intra-procedural imaging is expected to decrease.
Fiberoptic navigation
Fiberoptic RealShape (FORS) technology (Phillips) represents another notable advancement in navigation systems for trans-arterial interventions. FORS employs optical fibers embedded within instruments to provide a real-time 3D reconstruction of their position and shape. With the device tethered to the table at the bedside, it can be used alongside traditional catheters and guidewires, offering real-time visualization displayed on a bedside monitor. By analyzing the changes in the light spectrum caused by bends and twists in the optical fibers, FORS provides a detailed spatial map that diminishes reliance on ionizing radiation for instrument position determination. These systems allow for 3D visualization of the device, including simultaneous bi-plane viewing modes.
Initial clinical feasibility studies using FORS technology have been encouraging. FORS has been successfully used in a variety of endovascular navigational tasks such as catheterization of the thoracic aorta from the groin, both renal arteries, crossing occluded stends, and recanalization procedures. The usefulness of FORs based imaging guidance during these tasks was subjectively rated as better than standard guidance in 76% of procedures, and never worsen than standard guidance. In preclinical validation studies the mean difference in catheter tip position as displayed on the FORS monitor compared to fluoroscopy has been reported as 2.2 mm. However, the error in catheter tip positioning in significantly impacted by factors such as vessel wall deformation and patient movement, and differences in positions as great as 4-11 mm have been reported.
FORS offers the potential for navigation with reduced radiation exposure for patients and operators. However, the need for compatible high-end Phillips systems and the steep learning curve hinder its adoption in routine clinical practice. , Although this system reduces radiation, FORS does not entirely eliminate the need for fluoroscopy. Additional limitations include the limited working length of available catheters and guidewires and the necessity for tethering the system to the table, making the guidewire exclusively front-loadable. , The displayed positioning of the device is also subject to error induced by different patient positioning, movement, and vessel wall deformation from instrumentation. ,
Robotic navigation
Robotic navigation systems and steerable catheters have introduced a new level of precision in intravascular procedures. They allow operators to manipulate instruments with increased dexterity, facilitating complex interventions requiring nuanced maneuvers. Steerable catheters are more widely utilized in clinical practice than robotic systems, enabling operators to adjust the distal end of the catheter. Several commercially available options exist, including the Magellan Robotic System (Hansen Medical) and CorPath GRX (Corindus Vascular Robotics), with ongoing research focusing on novel designs to further improve positioning and dexterity.
Although not as prevalent in clinical settings, robotic navigation systems could herald a new era of precision in trans-arterial interventions. Robotic systems can be classified into magnetic and electro-mechanical categories. , Among various available systems, the Magellan and CorPath GRX have shown significant promise in trans-arterial interventions. The Niobe magnetic navigation system is notable for its advancements in coronary artery procedures. , The CorPath system, particularly, has demonstrated success in robotic renal artery stent implantation and uterine artery embolization. ,
While offering substantial benefits, emerging navigation technologies, including robotic systems, are not without limitations. The high costs of these systems and the need for extensive training are significant barriers to widespread adoption. Furthermore, certain procedures necessitate manual intervention, especially when navigating sensitive or highly variable anatomical regions.
Image fusion
Image fusion technologies have become an indispensable aspect of image-guided procedures, offering enhanced visualization capabilities by integrating data from multiple imaging modalities. By synthesizing these varied datasets, image fusion provides a more comprehensive understanding of complex anatomical structures, significantly improving the precision of image-guided interventions. Initial steps in image fusion involve image registration, which aligns images from different modalities to achieve spatial coherence. Once registered, the images are fused into a cohesive dataset that serves as a detailed map for clinicians to follow.
The adoption of image fusion techniques have resulted in significant reductions in radiation exposure, procedural times, and constrast usage. Nonetheless, challenges such as image distortion due to patient motion can affect accuracy. , Efforts to further optimize registration algorithms have improved in recent years, aiming to provide more robust and automated solutions that can adapt to the patient’s natural variability and dynamics during the procedure.
CT fusion with fluoroscopy exemplifies the utility of integrating static and real-time imaging. By combining dynamic fluoroscopic images with traditional CT or Cone-Beam CT (CBCT) datasets, clinicians gain access to a detailed anatomical roadmap, which significantly enhances procedural guidance. The integration offers notable advantages, including reduced radiation exposure and contrast usage, as well as shorter procedural times—factors that collectively improve both patient safety and operator efficiency.
Although not as widely deployed as CT-based methods, MRI fluoroscopic fusion offers a blend of MRI’s high-resolution and superior soft-tissue contrast capabilities with the real-time benefits of fluoroscopy. This type of fusion has been applied successfully in procedures like uterine artery embolization, where it provides a detailed roadmap and reduces the reliance on potentially nephrotoxic iodinated contrasts. Despite these advantages, there are certain phases in procedures where additional CBCT images might be necessary, and the fused images can suffer from misalignment issues due to vessel wall deformation and patient movement during the procedure.
The integration of augmented reality (AR) with image fusion is being actively explored as a way to enhance procedural navigation and expand physician capabilities. By projecting spatially registered 3-dimensional images onto the surgical field, AR technology can offer intuitive visual guidance for navigating complex anatomical structures, thereby reducing the cognitive load on the practitioner. Furthermore, these systems hold the potential to be combined with other navigation technologies like electromagnetic tracking, which could significantly decrease procedural times without compromising positional accuracy. These advancements represent an exciting frontier in the evolution of image fusion technology. Although currently in the early stages of development, augmented reality and advanced image fusion are expected to mature in sophistication and accuracy in the coming years, promising to redefine the operational landscape of interventional radiology.
Conclusion
The ongoing advancements in imaging and navigation technologies have significantly transformed trans-arterial interventions, enhancing both procedural success and patient outcomes. By incorporating a diverse range of imaging modalities—from fluoroscopy to magnetic resonance imaging, and augmented by innovative technologies such as Cone Beam CT and intravascular ultrasound—interventional radiology has effectively shifted from traditional open and minimally invasive surgeries to precise, image-guided interventions. This evolution not only results in improved clinical outcomes and reduced recovery times but also advances the management of complex diseases. Given the growth in demand for such interventions, the necessity for highly accurate, real-time imaging tools and techniques has become more pressing than ever.
Navigation systems and image fusion technologies further refine these capabilities by providing enhanced spatial orientation and integrating multiple datasets into cohesive models that facilitate precise targeting and delivery of therapies. Innovations such as electromagnetic and fiberoptic navigation systems, along with robotic navigation and augmented reality, promise to push the boundaries of what is achievable in interventional radiology. These technologies offer flexible platforms for enhancing the accuracy and safety of procedures, minimizing complications while maximizing efficacy. However, they are not without challenges, including high costs, learning curves, and the need for integration with existing systems. As these technologies continue to evolve, their potential to further revolutionize patient care within interventional radiology is immense, and their integration into clinical practice will be a crucial step in expanding the possibilities of what can be achieved through image-guided interventions.
Conflicts of interest
Dr. Ozen is consultant for Boston Sci, Cook Medical, Varian.
References
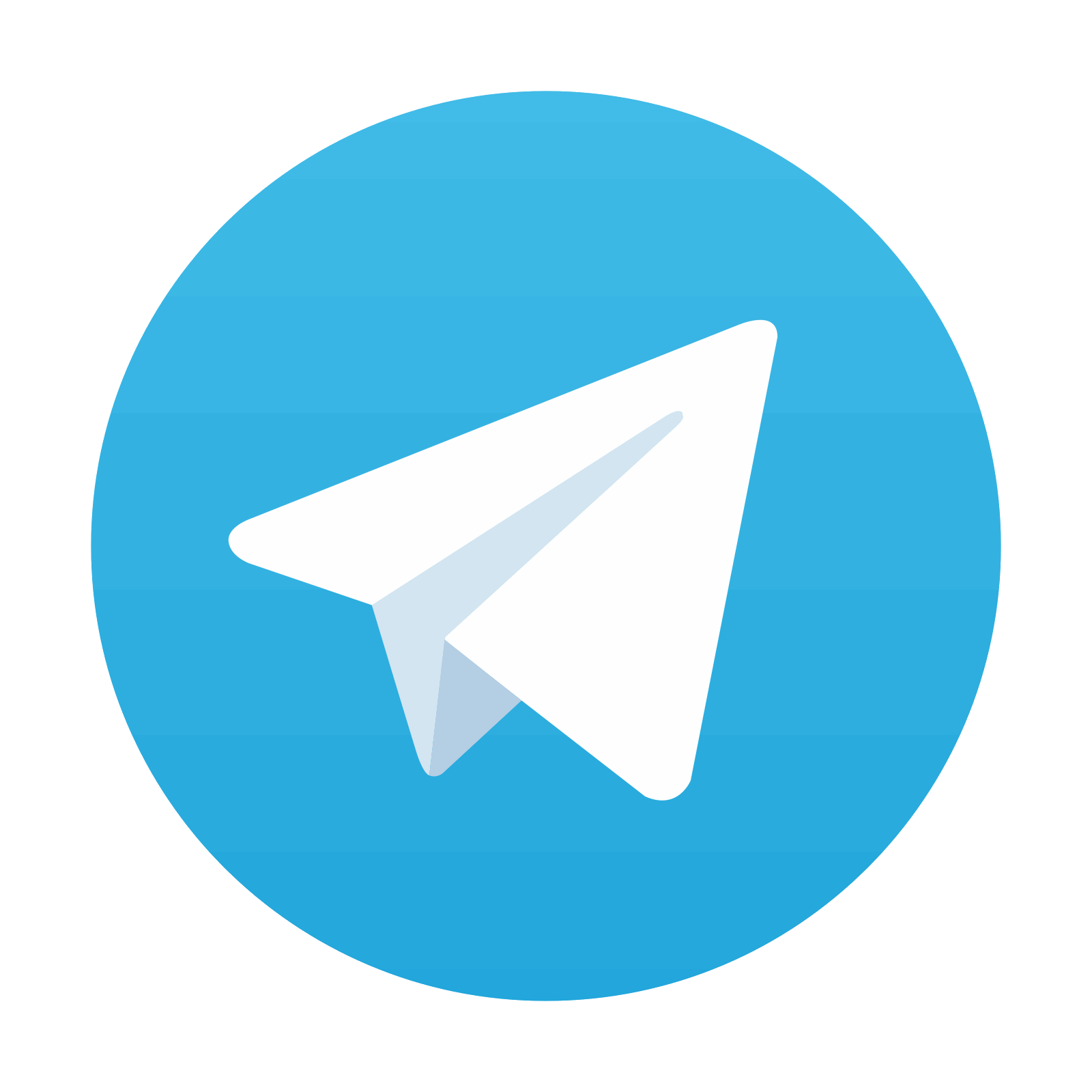
Stay updated, free articles. Join our Telegram channel

Full access? Get Clinical Tree
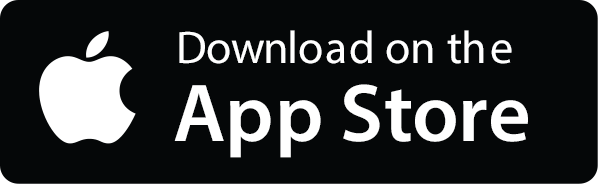
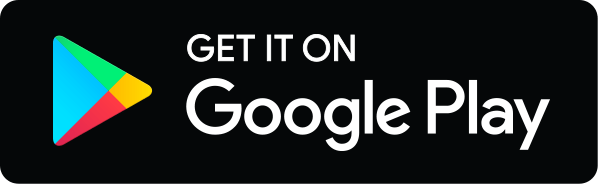
