Abstract
Objective
Transcranial magnetic resonance imaging (MRI)-guided histotripsy has been demonstrated to treat various locations in in vivo swine brain through a human skull. To ensure that the histotripsy treatment is delivered to the intended target location, accurate pre-treatment targeting is necessary. In this work, we investigate the feasibility of MR-thermometry and MR-acoustic radiation force imaging (MR-ARFI) to perform pre-treatment targeting of histotripsy in ex vivo bovine brain through a human skull.
Methods
A 700 kHz, 128-element MR-compatible histotripsy array was used to generate histotripsy and tone-burst sonications. The array’s electronic drivers were modified to also generate low-amplitude tone-burst sonications to perform MR-thermometry and MR-ARFI–based targeting. Twelve ex vivo bovine brains were treated with histotripsy at 35 MPa, 75 MPa and through a skull at 36 MPa. Before treating the tissue, both MR-ARFI and MR-thermometry were used to estimate the lesion location. Finally, the location of the histotripsy lesion was compared with the focus estimated by MR-thermometry and MR-ARFI.
Results
MR-thermometry and MR-ARFI were able to successfully perform pre-treatment targeting of histotripsy using the modified histotripsy array driver. Histotripsy focus was estimated with mean absolute errors along the transverse/longitudinal axis of 2.06/2.95 mm and 2.13/2.51 mm for MR-ARFI and MR-thermometry, respectively. The presence of the human skull reduced the pressure at the focal region, but it did not compromise the targeting accuracy of either of the two methods with a mean absolute error of 1.10/2.91 mm and 1.29/2.91 mm for MR-ARFI and MR-thermometry, respectively.
Conclusion
This study demonstrated that transcranial histotripsy pre-treatment targeting is feasible with MR-thermometry and MR-ARFI.
Introduction
Histotripsy is a non-invasive, focused ultrasound treatment method that uses high-pressure (> 20 MPa), short (1–2 cycles) ultrasound pulses at low duty cycle (≤1%) to disrupt the target soft tissues via acoustic cavitation mechanically [ ]. It has been proven effective in various tissue types in preclinical studies [ ] and was recently approved by the U.S. Food and Drug Administration for the non-invasive treatment of liver tumors. Histotripsy has also been shown to stimulate local and systemic immunological response in rodent tumor models and abscopal effect (reduction of off-target tumors) in human case reports [ , , ]. Transcranial histotripsy has been shown to treat the target brain tissues through an excised human skull without causing significant heating of the skull [ , ]. Recent studies have demonstrated magnetic resonance imaging (MRI) to be a viable tool for guiding and monitoring transcranial histotripsy treatments [ ].
Treatment planning for histotripsy in the abdomen region, such as liver and kidney, involves taking pre-treatment computed tomography and MRI scans to locate the treatment region [ ]. During treatment, an ultrasound imaging probe co-aligned with a histotripsy array is used to monitor the histotripsy treatment in real time because cavitation bubble clouds show hyperintense contrast in B-mode ultrasound images [ ]. MRI guidance is being investigated currently for histotripsy targeting and monitoring because ultrasound imaging is not ideal for imaging targets in the brain and does not provide good contrast between various tissue types in the brain. Transcranial MR-guided histotripsy uses MRI co-registered with the histotripsy transducer focal region to guide and monitor transcranial histotripsy for brain treatment. This work aims to assess the use of MR-thermometry [ ] and MR-acoustic radiation force imaging (MR-ARFI) [ ] to visualize the histotripsy transducer focal zone and the accuracy of these two MRI-based pre-treatment targeting methods for histotripsy.
MR-thermometry and MR-ARFI are routinely utilized in high-intensity focused ultrasound thermal ablation and blood–brain barrier opening treatment studies [ ]. MR-thermometry is used with low-temperature ultrasound heating to perform pre-treatment guidance for thermal ablation. This works well because the treatment and targeting methods use the same physical mechanism (ultrasound absorption causing temperature change). Alternatively, MR-ARFI uses milliseconds-long ultrasound bursts to displace the tissue a few microns, which is characterized by MRI to perform targeting for both thermal ablation and blood–brain barrier opening treatment studies.
MR-thermometry has been tested for histotripsy pre-treatment targeting, but not through the skull [ ]. MR-ARFI has not been tested for histotripsy pre-treatment targeting. This article evaluates MR-thermometry and MR-ARFI for the pre-treatment targeting of histotripsy with and without the skull.
The driving electronics of the histotripsy array were modified to generate low-amplitude tone-burst sonications along with high-amplitude, short histotripsy pulses. The accuracy of the two methods is compared at different pressure levels with and without trans-skull sonication. Even though tone-burst–based sonications and histotripsy both use ultrasound, histotripsy uses much higher acoustic pressures with substantial non-linear effects. Therefore, the accuracy of MR-thermometry/MR-ARFI for pre-treatment targeting of histotripsy treatments under high pressures and non-linear propagation is unknown, especially in the presence of the skull.
Materials and methods
Experimental setup
All experiments used a focused, 700 kHz, 128 element MR-compatible circular-shaped histotripsy array with an f-number of 0.74 (focal distance/aperture diameter: 15 cm/20.3 cm), full-width at half-maximum of the focal beam profile: 2 × 2 × 7 mm 3 . The same array has been used previously for our transcranial in vivo histotripsy experiments on pigs [ , ]. The array’s electronic drivers were modified to generate the low-amplitude tone-burst sonications required for performing low temperature heating and imparting ARF in addition to the single-cycle high-amplitude pulses required for histotripsy. This was done by optimizing the gate resistance of the high-speed insulated gate bipolar transistor to improve the electrical efficiency required for the tone-burst pulses while minimizing radio-frequency (RF) noise emissions during the high-amplitude pulses.
The array was placed in a 3T GE UltraHigh Performance human MRI scanner (GE HealthCare, Chicago, IL, USA), and 21 channel GE AIR coils were used for receiving MR signals. Treatments were performed on 12 ex vivo bovine brains sourced from a nearby slaughterhouse within 48 h of resection and refrigerated under degassed saline solution until the day of the experiments. On the day of the experiment, the tissues were degassed and set in 1.5% agarose gel at room temperature for up to 3 h before the experiment. The water bath was used as the acoustic coupling between the histotripsy array and the ex vivo brain tissue embedded in the agarose gel. Using a custom 3-D–printed insert, the brains were placed centered at the array’s geometric focus. The experimental setup inside the MRI scanner is shown in Figure 1 .
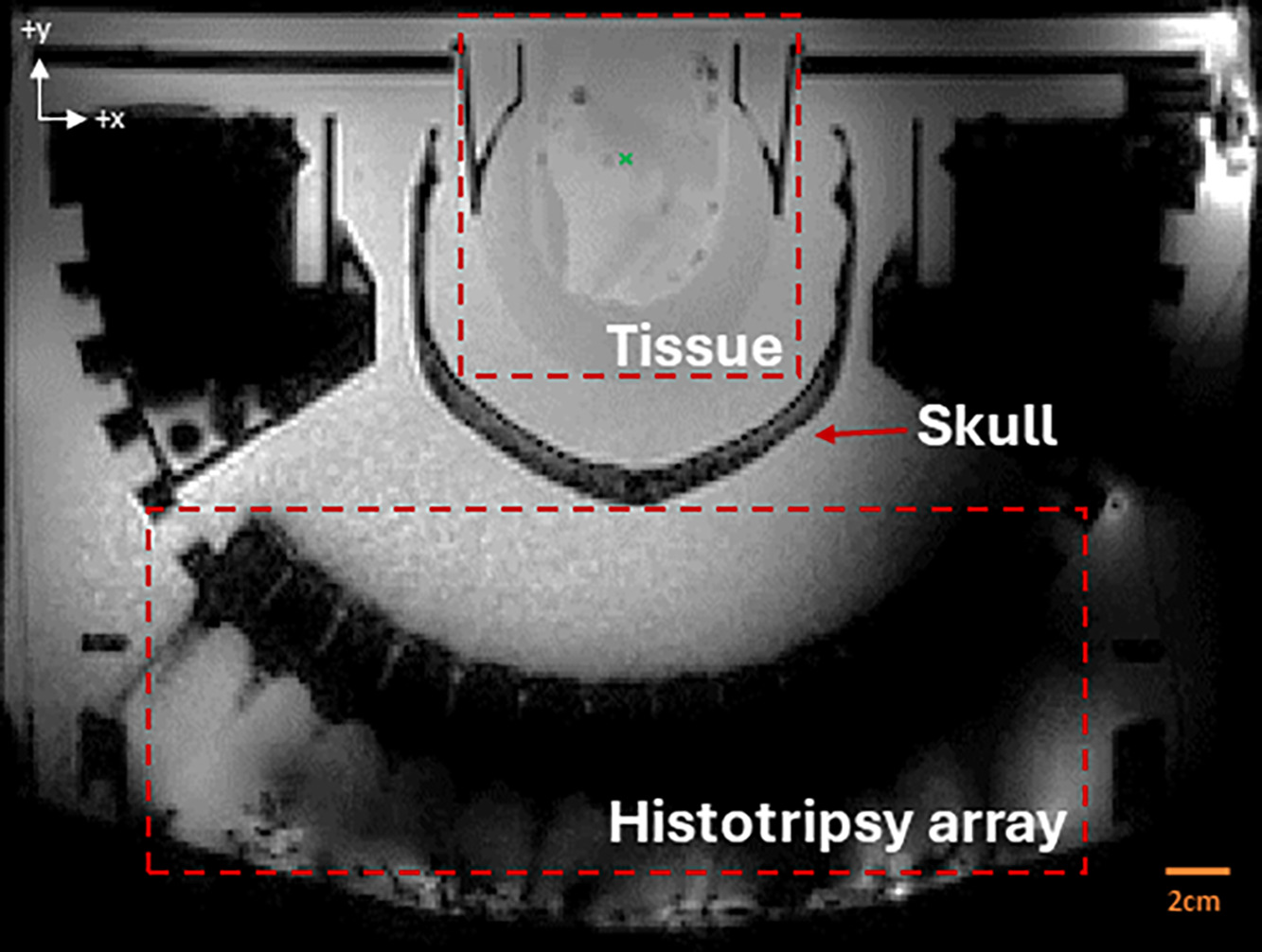
For the transcranial histotripsy treatment, two human skulls were acquired from the University of Michigan Anatomical Donations Program and were stored under degassed saline. The skulls were degassed under a vacuum chamber for 24 h before the experiment and held in place using screws mounted on the array scaffold. Since the skull significantly aberrates the ultrasound field, a hydrophone (CA-1135, Dynasen Inc, Goleta, CA, USA) was placed at the focus of the histotripsy array to measure the phase delays from each array element, which was then used to perform aberration correction.
Pre-treatment targeting using MR-thermometry
For pre-treatment targeting using MR-thermometry, focused heating was generated using continuous wave ultrasound (100% duty cycle) for 30 s. For experiments without the skull, the peak negative pressure (P − ) of 4 MPa was measured in the free field ( Fig. 2 ) by capturing the pressure waveform while firing the entire array using a fiber-optic hydrophone (Onda HFO-60, Sunnyvale, CA, USA). Through an excised human skull, the in situ P − of 1.2 MPa without was estimated, with approximately 70% ultrasound attenuation through the human skull with aberration correction measured previously. This resulted in spatial-peak temporal-average intensity of 437 W/cm 2 and 40 W/cm 2 for the cases without and with the skull, respectively.
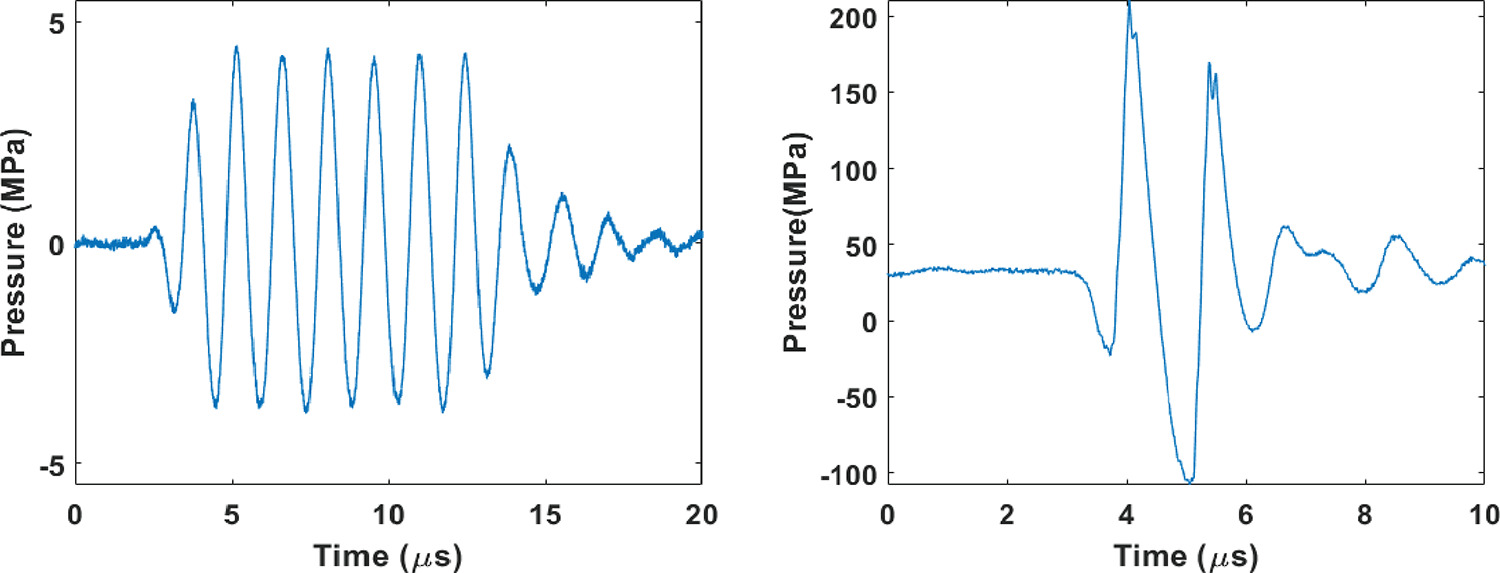
Proton resonance frequency-based MR-thermometry was performed simultaneously during ultrasound heating using a 3-D RF-spoiled gradient recalled echo scan using a stack of spirals acquisition with 8 shots/kz encode. The scan parameters were echo time (TE), 33 ms; repetition time (TR); 39 ms, flip angle (FA), 13°; field of view (FOV), 40 × 40 × 4.5 cm 3 ; matrix, 256 × 256 × 15; voxel size, 1.56 × 1.56 × 3 mm 3 ; and 4.68 s per acquisition. A field map-informed conjugate-gradient sensitivity map encoded (CG-SENSE) reconstruction was done using a field map and sensitivity map acquired from a separate scan [ ]. Phase difference images were calculated by subtracting the angle of the pre-heating image from the subsequent heating images. Zeroth-order phase correction was done to correct for scanner drift-induced phase changes before estimating the final temperature changes. To avoid a shift in the estimated focus owing to heat diffusion for MR-thermometry images, the earliest timepoint where the heating spot was visible was used to estimate the focus.
Pre-treatment targeting using MR-ARFI
Ultrasound ARF was applied using the 20 ms length pulses at the P − of 5 MPa without the skull and 1.5 MPa without through the skull with aberration correction. The pressure corresponds with a spatial peak pulsed average intensity of 780 W/cm 2 and 70 W/cm 2 with and without the skull. MR-ARFI scan was done using a 3-D spin-echo pulse sequence with a stack of spiral acquisition with 8 shots/platter and a pair of bipolar gradients on either side of the 180° pulse, similar to the method described in [ ]. The scan parameters were TE, 38 ms; TR, 500 ms; FOV, 40 × 40 × 4.5 cm 3 ; matrix, 256 × 256 × 15; voxel size, 1.56 × 1.56 × 3 mm 3 ; and 60 s per acquisition. Each gradient in the bipolar gradient had a first moment of 24 G · ms/cm and was oriented along the array’s longitudinal axis (scanner y axis).
Each ultrasound pulse was triggered by sending a trigger from the MR scanner to the array’s electronic drivers at the beginning of the second gradient from the first bipolar encoding gradient, resulting in a 4% duty cycle. The ultrasound array was turned on for 20 ms each TR to cover half of both the bipolar gradients along with the 180° pulse [ ]. Like MR-thermometry, field map-informed CG-SENSE was also used for reconstructing MR-ARFI images. Three MR-ARFI image volumes were acquired: two with the ultrasound array on and one with the ultrasound array off. For the acquisitions with the ultrasound array on, the ARF encoding gradients had opposite polarities. The phase images from the two ultrasound-on volumes were combined to increase the signal-to-noise ratio (SNR) of displacement images [ ]. Phase difference images were calculated by subtracting the averaged ultrasound-on images from the ultrasound-off images to calculate the MR-ARFI displacement maps. Phase unwrapping was performed on the phase difference images using the Goldstein method [ ].
Targeting accuracy evaluation
To evaluate the targeting accuracy, the array focus location based on pre-treatment MR-thermometry and MR-ARFI images was compared with the estimated centroid location of the post-histotripsy lesion determined by diffusion-weighted imaging (DWI). Single-point histotripsy lesions were generated using 1.5 cycle pulses and 100 Hz pulse repetition frequency (0.02% duty cycle) until the tissue was homogenized completely with a treatment time of 2 min. The histotripsy ultrasound pulse waveform is shown in Figure 2 .
Pressure waveforms from individual transducer elements were acquired using the fiber-optic hydrophone and summed to estimate the total pressure output from the transducer. Three experimental conditions were tested to examine the impact of pressure levels and skull presence on the targeting accuracy: (1) estimated in situ peak negative pressure P − of 35 MPa without a skull, estimated in situ P − of 75 MPa without a skull and estimated in situ P − of 36 MPa through the human skulls. The peak negative pressure of 35 MPa is slightly above the cavitation intrinsic threshold in the brain using 1 cycle pulses (28 MPa), whereas 1 cycle pulses and intrinsic threshold histotripsy (also known as microtripsy) was used to ensure the highest treatment accuracy. The peak negative pressure of 70 MPa was the maximum pressure achievable using the current setup. These pressure values cover the full range of pressures used in the prior transcranial histotripsy studies [ , ].
Because histotripsy causes cell membrane disruption, lesions show well on DWI images owing to the increased diffusion coefficient inside the lesion compared with untreated tissue [ , , ]. The lesions were imaged using a DWI scan (b = 0, 1000 s/mm 2 ) with echo-planar imaging readout. The scan parameters were TE, 52.6 ms; TR, 2 s; FOV, 40 × 40 × 4.5 cm 3 ; matrix, 256 × 256 × 15; voxel size, 1.56 × 1.56 × 3 mm 3 ; and 4 shots [ ]. Vendor-provided distortion correction was used to correct for echo-planar imaging image warping. Finally, the DWI images were aligned with the magnitude of the MR-thermometry and MR-ARFI images using MATLAB’s imregister function using a mutual information cost function to correct for rigid shifts between the three scans. The image registration was performed on a region containing only the brain tissues to avoid minimizing the objective closer to the ultrasound array and the water-air interface space, which are prone to high field-inhomogeneity. The lesion was identified by comparing the pre- and post-treatment apparent diffusion coefficient images and the lesion voxel with the maximum increase in the apparent diffusion coefficient was then found. Then all neighboring voxels with a 50% apparent diffusion coefficient of the peak were identified to be the treatment region. The threshold of 50% was made to reduce the contribution of voxels with partial volume effect owing to histotripsy.
To quantify the targeting accuracy of MR-thermometry and MR-ARFI, the center of mass of the marked DWI lesion was designated as the actual histotripsy target and compared with the center of mass focus identified by the MR-thermometry and MR-ARFI. The difference between the focus coordinates estimated from the two methods, and the estimated histotripsy focus was calculated as the targeting error. A negative error in the longitudinal and transverse axis corresponded to the estimated focus being more distal (+y) and right (+x) of the histotripsy focus, respectively.
Results
Pre-treatment MR-thermometry and MR-ARFI
MR-thermometry, MR-ARFI and DWI images could visualize heating, radiation force and histotripsy lesions in 12 tissue samples. Heating and ARFI focal regions were seen as oblong, elliptical-shaped regions with a tight focal spot ( Fig. 3 ). The peak temperature change and the peak displacement without the skull were approximately 8°C and 20 pm, respectively. Heating temperature uncertainty was estimated to be approximately 0.2°C. The estimated temperature increase generated by the MR-ARFI experiment was approximately 1.2°C.
