Abstract
Objectives
The enormous burden that cardiovascular diseases put on individuals and societies warrants reliable biomarkers of disease risk to optimize disease prevention. We studied longitudinal movement (LMov) in arterial walls using ultrasound of the common carotid artery (CCA). We believe that LMov could be a sensitive biomarker of cardiovascular health and in this study, we evaluate the intra-observer repeatability and inter-observer precision of our method. We also present a novel parameter ratio.
Method
Four independent researchers, called “observers”, analyzed CINE-loops of two separate ultrasound recordings of the CCA in 20 healthy individuals using an in-house developed program (ARTIC) based on MATLAB. Vessel wall displacement was measured in two dimensions and results between repeated measurements for one research subject and between observers were compared. We also present and evaluate a novel parameter ratio, the notch ratio (NR) which by combining velocities of phases B and X reduces variability and improves comparability of LMov analysis in late systole.
Results
Most LMov parameters showed good repeatability with coefficient of variation (CV) values 10%–26%. CV values for radial and IMT measures were excellent between 1% and 7%. Intra-class correlation coefficients (ICC) for inter-observer precision of single measures showed excellent values ICC > 0.9. NR improved LMov analysis in late systole and showed homogenous values of 1.36–1.45 between observers and good CV values of 9%–20%.
Conclusion
We demonstrate good intra-observer repeatability and excellent inter-observer precision of LMov analysis using ARTIC. NR displays similar consistency. This promotes further research on larger study populations using the same method focusing on the possible pathophysiological link between LMov and cardiovascular disease.
Introduction
Cardiovascular disease accounts for a tremendous disease burden on individuals and societies globally. The World Health Organisation points out cardiovascular disease as the leading cause of mortality and morbidity in the whole world [ ]. Reliable biomarkers can help optimize treatment and are instrumental in disease prevention. Optimal biomarkers are minimally invasive, easily interpretable, robust in function, and consistent across subjects and observers. Carotid intimal medial thickness (IMT) is a predictor of atherosclerotic disease and coronary heart disease [ ] as well as cardiovascular disease in general [ ]. Carotid artery wall stiffness is believed to cause exaggerated rises in systolic blood pressure and pulse pressure [ ] and the association between carotid wall stiffness, carotid stenosis and stroke is well described [ ]. There is also increasing evidence for a two-way interaction with hemodynamic forces acting on the vessel walls in maintaining vascular health [ ]. Overall, changes in the elasticity of arterial walls are known to occur in cardiovascular disease and as such could be a predicting factor of future risk [ , ]. A surrogate marker of arterial wall stiffness is the overall diameter change of the lumen in relation to blood pressure during a cardiac cycle [ , ].
Longitudinal arterial wall movement (LMov) – was first described invasively in canine aortas [ ] and then noninvasively with ultrasonography in porcine models [ ] and humans [ ]. Several different methods of measuring this motion have since been developed and are reviewed by Rizi et al [ ]. The common carotid artery (CCA) has mostly been the vessel of choice for investigating LMov due to its accessibility for high-resolution ultrasound examination. LMov is still incompletely understood both in its physiological and potential pathophysiological role. The pattern of LMov in the CCA shows great variation between individuals but the movement pattern within individuals is well consistent over a 4-month period in adults [ ], 12-month period in children [ ], and after regular physical exercise over three months in healthy adults [ ]. LMov consists of five distinct phases (A, B, C, W, X) [ ] as shown in Figure 1 , where the underlying mechanisms are only partially understood. Suggested acting forces include myocardial contraction through cardiac-vascular coupling [ , ], blood pressure [ , ], smooth muscle cell activation in the vessel wall [ , , , ] and lumen diameter change with associated elastic recoil [ , ]. There is data supporting a central energy source (i.e., the myocardium) for LMov [ ] both as a pulling force during ventricular contraction and as a pulse pressure generator. A central source of energy would suggest effect attenuation (i.e., decrease in LMov) with increasing distance from the source which has been observed [ , ]. A difference in magnitude of some LMov phases between the right and left CCA has been observed and can support the notion of a central energy source by the same logic. The right CCA branches off the brachiocephalic trunk whereas the left CCA comes directly off the aortic arch with fewer vascular bifurcations and shorter total distance from the heart. The right CCA seems to show lower amplitudes of some LMov phases compared to the left CCA [ ].
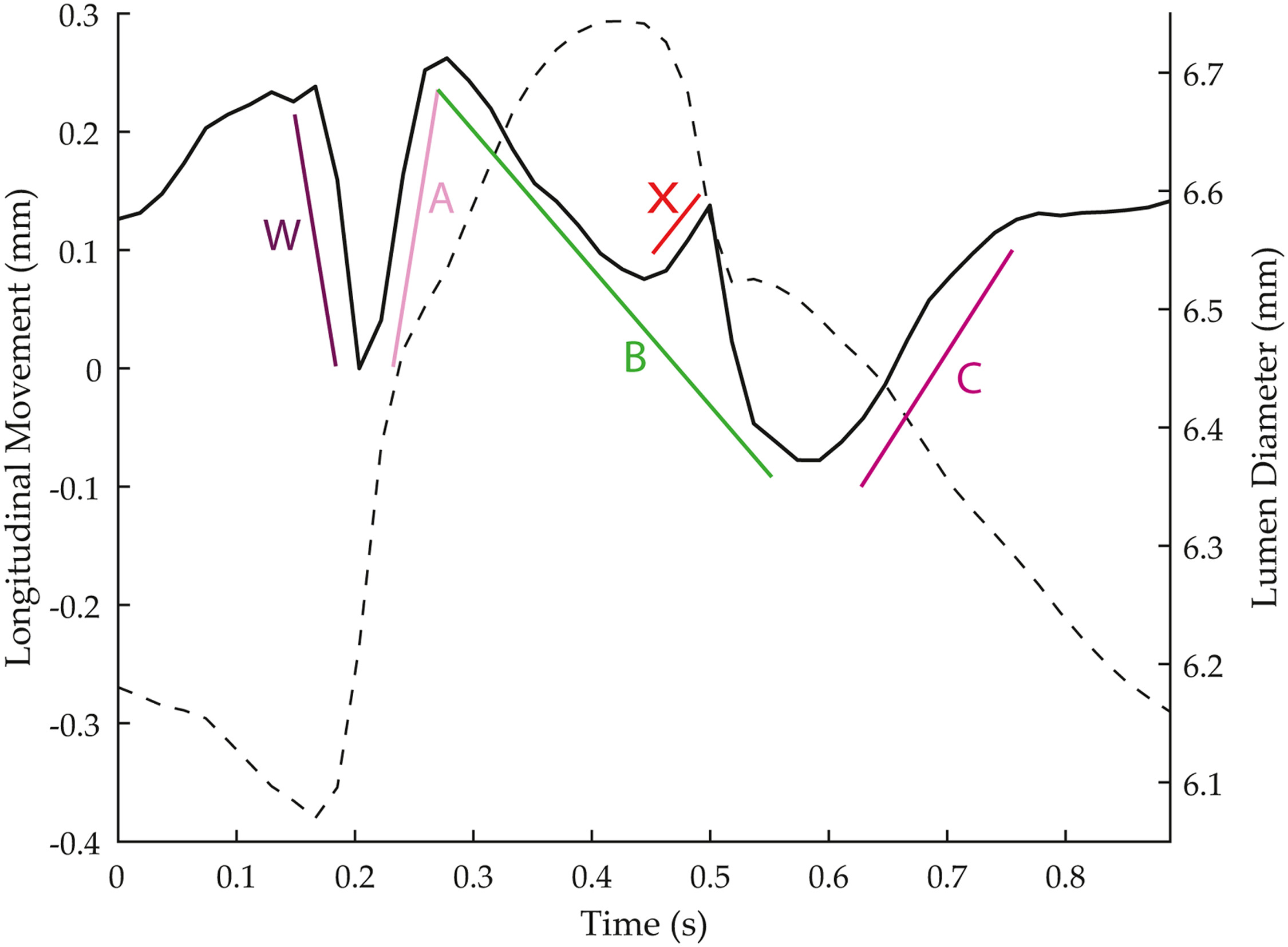
LMov also differs in magnitude across the different layers of the vascular wall ( intima, media, adventitia ) which results in intramural shearing across the layers [ ]. The largest strain occurs across the adventitia-media junction (external elastic lamina) [ ] potentially affecting the vasa vasorum [ ] which lies in this interface. Intramural shearing forces due to LMov could, therefore, affect vascular wall health through vascular parenchymal perfusion and as such contribute to vessel wall pathology [ ]. LMov seems to be reduced in patients with carotid artery atherosclerosis, suspected coronary artery disease, type II diabetes [ ] and periodontal disease [ ]. The combined data suggests that LMov, can play a significant role in vascular physiology and pathology possibly serving as a biomarker of cardiovascular health.
We have incorporated our intensity phase tracking algorithm (IPT) [ ] into our graphical user interface for arterial characterization (ARTIC) [ ]. The aim of this study was to assess the intra-subject repeatability and interobserver precision of measurements of LMov, IMT, lumen diameter, lumen diameter changes and strain (the ratio between lumen diameter change and lumen diameter) using ARTIC on human subjects. Many different methods for evaluating longitudinal arterial wall motion have been developed but no consensus exists on what the optimal method is. Besides evaluating ARTIC against itself we also compared it to our previous best in-house developed 2D block-matching method [ ] to see if either one is superior. In addition, due to significant age-related variation of phase X [ ], we believe that it may be a good candidate for a potential biomarker of vascular health. However, in some individuals it can be a challenging phase to visualize and measure because of the overlapping phase B. Because of this, we sought to establish a new parameter combining information from both phases B and X to improve the analysis of phase X. The primary endpoints were thus:
- a).
To evaluate the repeatability of measurements in the same subject from two separate ultrasonographic recordings (intra-subject repeatability) using ARTIC
- b).
To evaluate the precision of measurements from the same subjects between four independent observers (inter-observer precision) using ARTIC
- c).
Establish a new parameter for estimating phase X in proportion to phase B
- d).
Compare the IPT algorithm with an older in-house 2D block-matching method
Materials and method
Subjects
The study population consisted of 20 healthy adult individuals (12 males, mean age 48, range 23–69, and 8 females, mean age 49, range 22-65) with no history of cardiovascular disease. All participants were non-smokers and instructed to abstain from alcohol and caffeine two hours prior to the ultrasound examination. All participants gave informed written consent in accordance with the Helsinki Declaration. The study was approved by the Ethics Committee of Lund University.
Ultrasound recordings
A total of 40 (two per subject) 60 Hz recordings were obtained using a commercial ultrasound scanner (Philips Medical System, Bothell, WA, USA) with a 38 mm 5–12MHz linear array transducer (L12-3). The right CCA was scanned in the longitudinal direction 2 cm proximal to the carotid bifurcation. The entire thickness of the artery had to be well visible during the entire cardiac cycle when recording. All measurements were performed with the subject in a supine position in a quiet room following 10 minutes of rest. To avoid motion artifacts from respiratory muscle movement [ ] the subjects were asked to gently hold their breath in mid-respiration (i.e., not maximal inspiration or expiration) while vessel wall movement during 4–6 cardiac cycles was recorded. Two such recordings ( r 1 & r 2 ) were performed for each subject and then exported in DICOM format. To minimize the effect of physiological variation on measurements r 1 and r 2 were taken only a few minutes apart with the subject retaining the same resting supine position. To minimize variation in recording technique the same experienced sonographer performed all the measurements.
Ultrasound recording analysis
The exported DICOM files (CINE-loops) were analysed in ARTIC, a tailored user interface for automatic measurements of vessel lumen diameter, diameter change, IMT and LMov parameters ( Fig. 2 ) that has been earlier used in two studies [ , ]. The operator manually chooses which segment (region of interest [ROI]) of the arterial wall the program analyses. The vessel wall boundaries are automatically identified by the program and presented as a set of markers in the specified ROI, but the operator still has the possibility of manually adjusting the markers. After analysis, a summarising graph of average LMov for the recorded cardiac cycles is produced. On the graph, the operator manually marks the start of phase A (or phase W if A is not visible), after which the program automatically identifies the remaining phases by detecting the minimums and maximums in the motion, velocity, and acceleration curves, respectively. Even here there is the possibility of manually correcting the position of the different phase markers should disagreement between the operator and program algorithm arise. Once the phases are identified, their magnitude and velocity are automatically calculated as the total displacement and maximum gradient for a given phase respectively. The method is highly sensitive and has the capacity of estimating dynamic processes on a sub-pixel level corresponding to changes in arterial wall motion and lumen diameter on a µm scale [ ]. Specifically, the LMov parameters were the maximal longitudinal displacement (Max) during a cardiac cycle, the magnitudes of different phases of LMov (A mag , B mag , C mag , W mag , X mag ) and the phase velocities (A vel , B vel , C vel , W vel , X vel ). Max was defined as the difference between the maximum and minimum point of LMov.
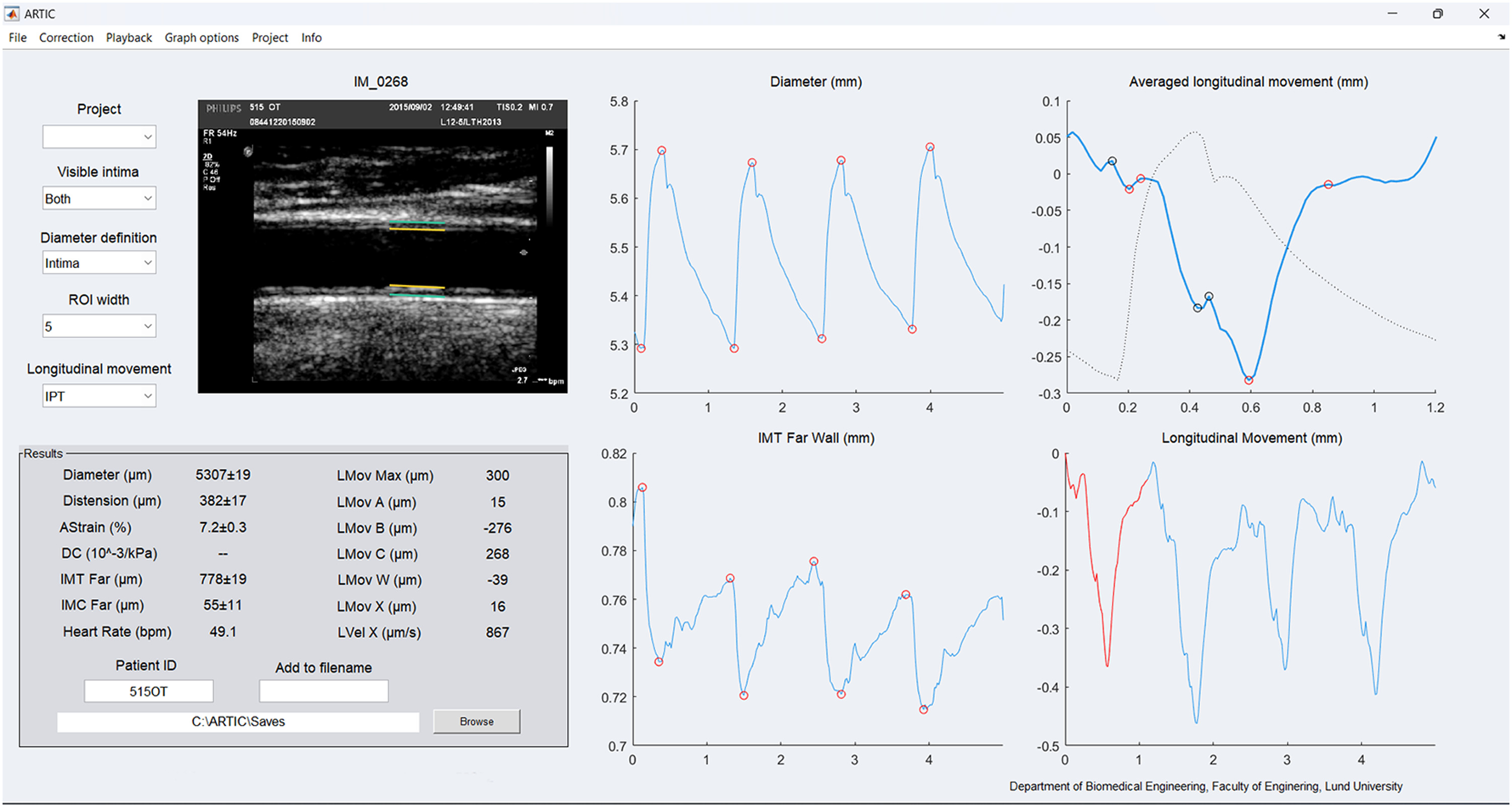
Echoes of the different arterial wall layers (lumen-intima [LI] and media-adventitia [MA]) are automatically tracked during all the frames. LMov is measured using an intensity phase tracking (IPT) algorithm [ , ]. The IPT method is described in detail in [ ]. In short, IPT is based on the local phase variation of the amplitude information (amplitude converted to phase information). By combining phase differences between frames with the estimated local phase gradients total displacement and velocity of the motion can be estimated. To obtain an average cardiac cycle of the motion, ARTIC applies a frequency-domain approach that also reduces the impact of global motion, such as breathing artifacts [ ].
Lumen diameter was defined as the distance between the near and far LI-layers at the end of diastole which corresponded to the minimum diameter during a cardiac cycle. Lumen diameter change denoted the difference between the maximum and minimum values of lumen diameter during a cardiac cycle. IMT was defined as the distance between lumen-intimal- and media-adventitial junctions of the far wall at the end of diastole. The different layers were segmented by a combination of peak and threshold detection [ ]. Sub-pixel resolution was achieved using linear interpolation [ ].
Data analysis
We investigated the repeatability in measurement of lumen diameter, diameter change, IMT and LMov parameters between r 1 and r 2 for each subject and the precision between four different observers (O 1-4 ). Of the four observers one ( O 1 ) was experienced in using the program while the rest ( O 2 -O 4 ) were previously unacquainted with ARTIC and performed their analysis after a short introduction. Each observer analyzed the same recordings using ARTIC independently choosing the ROI of the vessel wall to analyze and retain the possibility of manually correcting program-determined LMov phases. To assess intra-subject repeatability, coefficients of variation (CV %) were calculated for all different parameters for each observer. Intra-class correlation coefficients (ICC) calculated using SPSS (IBM) were used to assess the inter-observer variation. Since all observers analyzed the same CINE-loop using the same analysis program (ARTIC) it was reasonable to apply a two-way mixed effects model where observer effects are variable, and measure effects are fixed. Similar ICC values were calculated when comparing the results between the old 2D block-matching method and ARTIC for observer 1. In this calculation the different methods were regarded as “observers” and observer 1 as “the method” with regard to ICC. Degrees of freedom were n – 1 where n is the number of study subjects. Level of significance was p < .05.
Notch ratio
Phase X is often small, especially in younger individuals in whom no apparent positive deflection can be visually detected but a temporary change in phase B velocity can be measured. All our observations so far indicate that phase X causes a decrease in phase B velocity (phase B being retrograde) suggesting that the driving force for phase X is antegrade in direction and the vector component of phase X is correspondingly also positive. When apparent, phase X is an antegrade deflection and the magnitude of phase X (X mag )> 0, but in cases where only a slowing down of phase B can be noted X mag = 0. In these cases, the slowing down of phase B indicates that there is a positive antegrade force (phase X) occurring “under” phase B even if it is not visually as apparent on the graph. The exact cause of the antegrade motion of phase X is unknown but close temporal proximity with the dicrotic notch in the lumen diameter curve suggests a relationship with closure of the aortic valve. A possible mechanism could be an antegrade longitudinal elastic wave that propagates through the vascular wall, created by motion arising at the closure of the aortic valve.
While X mag can be difficult to measure when very small, often producing 0-values, the velocity of phase X (X vel ) which can take both positive and negative values is a more sensitive parameter when addressing phase X. Phase X shows no correlation to phase B, and we assume them to be independent phenomena despite their temporal overlap. Any distension or longitudinal movement of the vessel wall bears a natural relation to vessel elasticity. Since phases B and X are assumed to have different physiological causes, their relationship to vessel elasticity can also be expected to differ. Thus, any effect that phase B could have on the measurement of phase X occurs independently of phase X and, therefore, promotes the need of taking phase B into account when measuring phase X. Hence, we propose a “Notch ratio” (NR) using:
NR=(Bvel−Xvel)Bvel
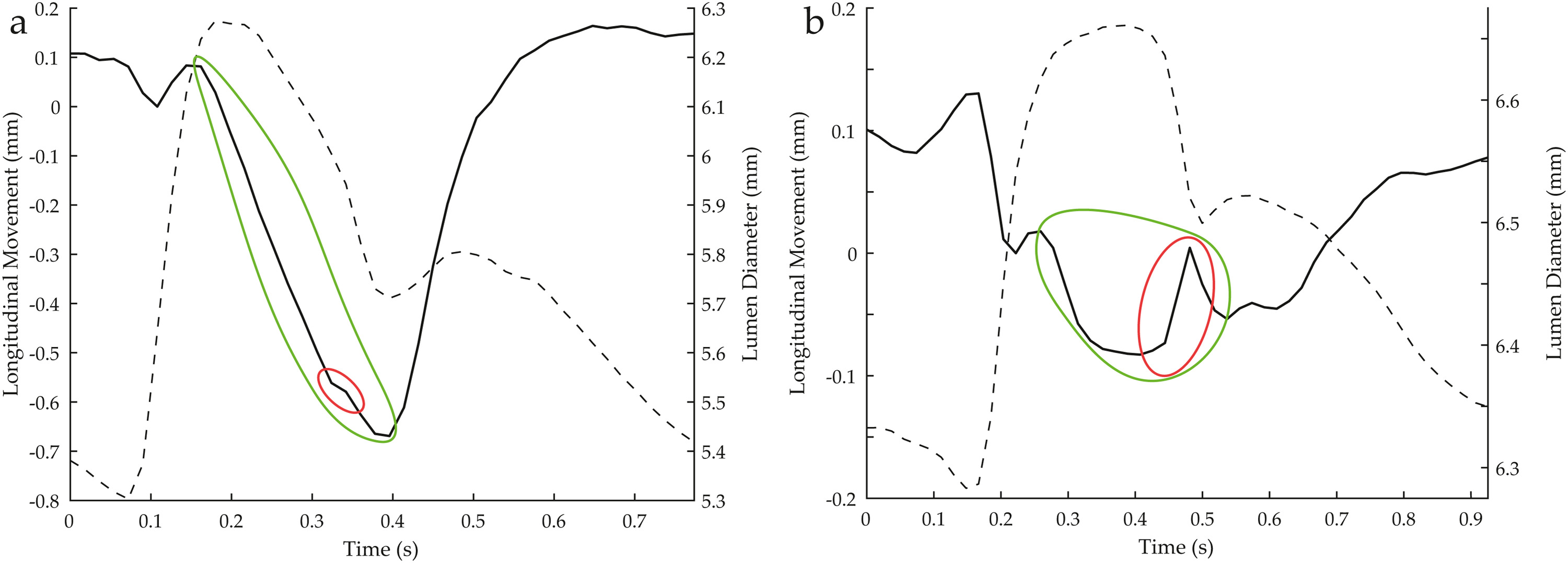
X vel | NR |
---|---|
X vel ∼ B vel | ∼ 0 |
X vel < 0 | < 1 |
X vel ∼ 0 | ∼ 1 |
X vel > 0 | > 1 |
Comparison of the novel method with an in-house block-matching method
ARTIC was compared to our earlier in-house developed 2D block-matching method that utilizes unbiased block-matching [ ] and block expansion in the time domain [ ], combined with a modified version of the Woody average [ ] on a partly overlapping study population of 20 healthy adult individuals. The block-matching method was previously used in our study [ ], where the data files were analyzed by observer (O 1 ). Now 20 of the same files were also analyzed using ARTIC. As far as it was possible, the same wall position was used for both methods. Mean difference, standard deviation of the differences, and ICC were used as comparison methods.
Results
To understand the measured parameters, it aids keeping in mind the graphical presentation of the typical LMov phases ( Fig. 4 ). Positive values imply antegrade (in direction of blood flow) movement and are illustrated with an upward deflection on the graph. Negative values imply the opposite. The velocity of the different phases that is, the velocity of the movement in the arterial wall is illustrated by the angle of incline or decline on the graph. Averages of observer averages with (ranges) for the different parameters were as follows; Max 393 µm (94–1070 µm), A mag 91 µm (0 to 331 µm), B mag -310 µm (-926 to -70 µm), C mag 279 µm (11 to 1070 µm), W mag -95 µm (-335 to -0.1 µm), X mag 34 µm (0–203 µm), A vel 2.2 mms -1 (0 to 7.8 mms -1 ), B vel -3.3 mms -1 (-7.6 to -1.2 mms -1 ), C vel 2.7 mms -1 (0.5 to 10.4 mms -1 ), W vel -2.8 mms -1 (-8.9 to 0 mms -1 ), X vel 1.0 mms -1 (-2.8 to 5.4 mms -1 ), diameter 5662 µm (4807–6980 µm), distension 605 µm (306–1025µm), strain 10.1% (5.1%–19.2%), IMT 662 µm (457–1019 µm) and for NR (0.37–3.80). In general, intra-subject variability was low among all four observers. For LMov parameters CV values ranged mostly between 10-26% except for A vel and X vel which showed larger heterogeneity. CV values for radial measures (lumen diameter, lumen distension and IMT) were excellent between 0.7% and 7.2% ( Table 2 ). ICC analysis of inter-observer precision for single measures showed excellent values ranging between 0.873 and 0.994 where all but one parameter showed ICC > 0.9 ( Table 3 ). The averaged notch ratio (NR) showed little dispersion ranging from 1.36 to 1.45 between the four observers and displayed low intra-subject variability with CV values between 9% and 20% ( Table 4 ).
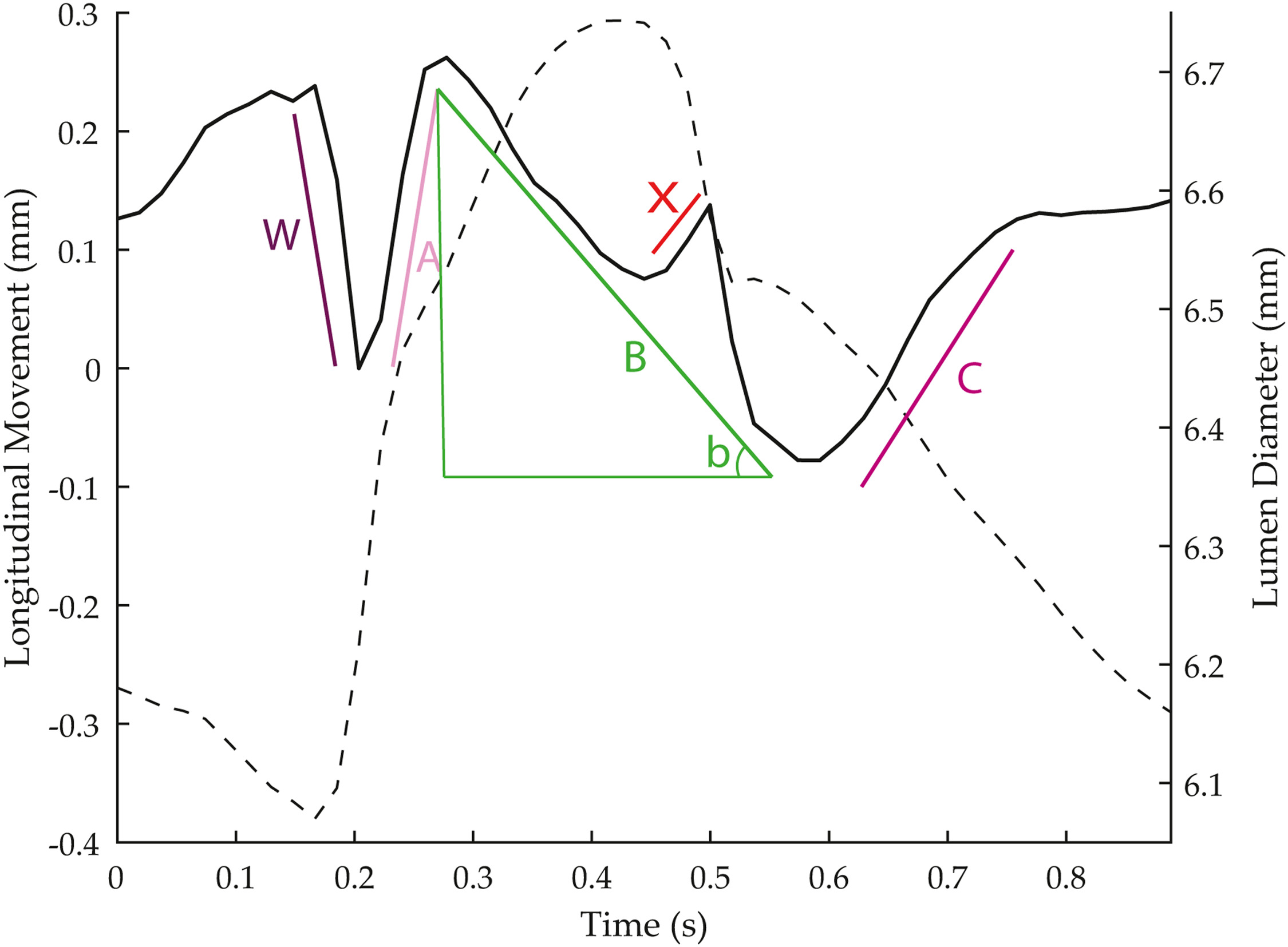
