ABSTRACT
Objective
The rupture of vulnerable plaques in the carotid artery is a leading cause of strokes. While magnetic resonance imaging (MRI) is the standard for quantifying plaque composition, its high costs and lengthy procedure times limit large-scale use. Compound ultrasound strain imaging (CUSI) ultrasound offers a non-invasively alternative by assessing tissue deformation/strain within the arterial wall. Previous studies have demonstrated the relationship between strain values and plaque composition, primarily focusing on longitudinal acquisitions, which only image the proximal and distal parts of the wall. This study examines CUSI in transverse imaging planes, which enables visualization of the entire cross-section of the vessel wall. CUSI has already been validated on a symptomatic population. Therefore, the aim is to determine whether CUSI can differentiate plaque composition in asymptomatic individuals with an increased cardiovascular risk profile, validated by MRI.
Methods
This cross-sectional study included 42 plaques in 28 participants. Ultrasound data were acquired with a Samsung Medison Accuvix V10 with an L5-13IS transducer and RF-interface. An experienced neuroradiologist classified plaques with MRI using the Siemens 3.0T MAGNETOM Skyra MRI (Erlangen, Germany) with a dedicated coil. Strain differences were compared across four plaque categories (calcified, lipid, hemorrhagic, fibrous/aspecific) and for vulnerable versus stable plaques.
Results
A difference in strain values was found between lipid and calcified plaques (Kruskal–Wallis test, p < 0.05). Additionally, vulnerable plaques exhibited higher strain values than stable plaques (independent samples test, p < 0.05).
Conclusion
CUSI values differ between lipid and calcified and between stable and vulnerable plaques. Minor differences were found between the other plaque types.
Introduction
Between 1990 and 2019, the absolute number of incident strokes increased by 70.0% reaching 12.2 million strokes worldwide [ ]. Due to the increasing prevalence of obesity and cardiovascular diseases (CD), this trend is anticipated to escalate substantially in the coming decades [ ]. CD render carotid plaques more vulnerable, thereby increasing the risk for a stroke [ ]. There are several types of strokes, where ischemic stroke predominates and constitute 62% of all occurrences [ ]. Atherosclerotic plaques initially manifest without apparent symptoms. As plaque accumulates within arterial walls, an arterial remodeling process is initiated until the stenosis reaches approximately 40%. Beyond this point, further enlargement becomes unfeasible which result in progression of plaque-induced stenosis [ , ]. When an atherosclerotic plaque ruptures, the thrombogenic material forms a blood clot, which is transported downstream toward the brain. This clot has the potential to occlude cerebral arteries, resulting in an ischemic stroke [ ].
A plaque can comprise one or multiple components, with lipid, hemorrhage, calcifications and fibrous material being the most prevalent [ ]. Existing evidence indicates a higher incidence of cerebrovascular events in high-risk plaques (vulnerable) compared to low-risk plaques (stable) [ ]. Vulnerable plaques are characterized by a substantial lipid pool with high concentration of macrophages that is covered by a thin fibrous cap. In contrast, stable plaques present a core with small or absent lipid pool and, few macrophages, that is shielded from the bloodstream by a thick fibrous cap [ ]. However, the literature presents some ambiguity regarding the role of calcifications in plaques as indicators of stability or vulnerability. Studies have concluded that the location, number, size and shape of calcifications play a role in plaque vulnerability and the risk of rupture [ , ].
The optimal therapeutic approach for asymptomatic plaques remains a subject of discussion. Due to uncertainty surrounding the identification of plaques at high risk of rupture, a generalized treatment is currently administered to all patients [ , ]. This approach is further complicated by the historical lack of determination of plaque compositions, making it hard to demonstrate effectiveness of the treatment. At present, generalized treatment is based on the grade of luminal stenosis [ , ]. Recent insights suggest that plaque vulnerability provides more information about rupture risk compared to the grade of stenosis [ ]. Preventive and more specific treatments for vulnerable plaques may become viable if plaque composition imaging becomes standard practice in clinical care [ ].
Histopathological analysis remains the ultimate gold standard for analyzing plaque components [ ]. However, since plaque removal is not performed in asymptomatic patients, magnetic resonance imaging (MRI) imaging is considered the gold standard for non-invasive plaque characterization [ ]. MRI classifies plaque composition into four types: lipid, calcium, hemorrhaged and fibrous—with notable sensitivity and specificity [ ]. Although MRI is highly accurate, it is often regarded as complex because calcified components appear hypointense, complicating the evaluation of adjacent structures [ ]. Additionally, distinguishing between lipid and hemorrhagic components can be challenging due to their similar appearances on MRI images. Moreover, many plaques are composed of multiple components, making it difficult to classify them within a single category, particularly given the limited resolution of the technique. Additionally, the main limitations of MRI include its relatively high costs and the time-consuming nature of the process.
Ultrasound strain imaging can address these issues due to its shorter scan time, cost-effectiveness and superior temporal and spatial resolution in assessing the carotid arteries. Strain imaging quantifies tissue deformation resulting from fluctuating intraluminal pressure. Calcified and fibrous plaques tend to deform less because of their stiffer composition compared to lipid and hemorrhagic plaques, enabling strain imaging to potentially differentiate between these plaque types. Existing studies have demonstrated the effectiveness of strain imaging for plaque composition analysis, but they mainly focused on longitudinal acquisitions [ ]. Longitudinal acquisitions, which only visualize the anterior and posterior vessel walls, may underdiagnose or miss carotid plaques located on the lateral sides of the vessel, which is a limitation.
To address the limitations of longitudinal acquisitions, a technique called compound ultrasound strain imaging (CUSI) has been developed [ ]. This method enables the visualization of the entire vessel wall in a cross-section and can estimate the plaque stiffness across its circumference. CUSI operates within transverse planes with three ultrasound beams at different angles [ ]. Phantom studies have validated the efficacy of this technique [ , ]. Furthermore, a study by Hansen et al. [ , ] reported high sensitivity and specificity of CUSI in discriminating lipid and fibrous tissue in a symptomatic population, compared to histology following an endarterectomy procedure. It remains unclear whether CUSI can effectively differentiate asymptomatic plaques in the transverse plane. If CUSI can demonstrate this capability, it could play a prominent role in the diagnosis, large-scale screening and preventive treatments of asymptomatic patients. Since an asymptomatic population does not undergo an endarterectomy procedure, this study employs the non-invasive golden standard (MRI) to classify the plaques. The purpose of this study is to explore whether CUSI can differentiate plaque composition in asymptomatic individuals with an increased cardiovascular risk profile, validated by MRI.
Method
Study design
This study is a cross-sectional study. All measurements were conducted at Radboudumc Nijmegen.
Study population
The study population was drawn from the Nijmegen Biomedical Study—Non-Invasive Markers of Atherosclerosis 3 (NBS-NIMA 3) database, an extension of the NBS-NIMA 1 cohort, comprising a random sample of 10.000 inhabitants of Nijmegen, the Netherlands [ ]. From NBS-NIMA 1, 619 subjects had a body mass index (BMI) above 27 kg/m 2 , with 54% showing metabolic disorders or cardiac vascular disease, while 46% were apparently healthy. A total of 500 potential participants were invited from the 619 possible participants for the NBS-NIMA 3 study, divided into two groups: 250 subjects with metabolic syndromes (MetS) and/or CD, and 250 subjects without these conditions. Therefore, the 250 participants without those conditions were eligible for this study. The inclusion criteria included being a participant within the NBS-NIMA 1 study, elevated cardiovascular risk profile without the presence of MetS and/or CD, defined as individuals aged between 50 and 70 y, with a BMI exceeding 27 kg/m 2 . Exclusion criteria included a recent cardiovascular event, not having a minimal plaque thickness above 2 millimeters, inability to measure a diameter curve for at least one cardiac cycle within the strain imaging analysis, and artifacts within the MRI or CUSI imaging. In total, 28 participants encompassing a total of 42 carotid plaques, were included in this study.
Prior to participation, written informed consent was obtained from all participants, who retained the freedom to withdraw from the study at any point without providing a reason. The research protocol adhered to the ethical principles outlined in the Declaration of Helsinki and received approval from the Ethics Review Board (CMO) in the Arnhem-Nijmegen region.
Study procedures
If included, participants underwent both CUSI and MRI of the carotid arteries. Initially, participants received a CUSI ultrasound of the carotid arteries, followed by an MRI scan within 4 wk due to logistical reasons.
In this study, CUSI data were compared between the four plaque categories: calcified, lipid, hemorrhagic, fibrous/aspecific. MRI was used to categorize plaques into these four categories. A radiologist with 11 y of experience, blinded to the CUSI data, classified the plaques based on MRI features on T1- and T2-weighted (T2w) images. For example, a plaque hyperintense on both images was categorized as a lipid plaque, Table 1 .
Plaque Components | T1w-Image | T2w-Image |
---|---|---|
Lipid | Hyperintense | Hyperintense |
Hemorrhagic | Hyperintense | Hypo- or isointense |
Calcified | Hypointense | Hypointense |
Aspecific/fibrous | Hypo- or isointense (heterogenous) | Hyperintense |
Given the ambiguity in the literature regarding the vulnerability of calcified plaque composition, caution was exercised when categorizing plaques with calcified components . For this reason, in this study, calcification was identified as a stable factor. However, if vulnerable components (such as lipid or hemorrhagic) were present within a plaque containing calcifications, the plaque was classified as vulnerable.
Ultrasound acquisition
The participants underwent ultrasound (CUSI) of the carotid arteries in accordance with the protocol of Hansen et al. [ ]. The Samsung Medison Accuvix V10 ultrasound system (Seoul, South Korea), equipped with the Samsung L5-13IS linear array transducer ( f center = 8.5 MHz) was employed for this purpose. An experienced sonographer initially examined the common carotid artery, bifurcation and internal carotid artery in a longitudinal plane. Luminal narrowing was visually assessed, the transducer was subsequently rotated to the transverse plane to image the radial vessel wall at the plaque site with the highest stenosis rate.
A dedicated imaging mode, called radial zone, was activated to record the raw radiofrequency data ( f sample = 61.6 MHz) for 3 s, capturing insonification angles of 0°, + θ , and − θ (20° ≤ θ ≤ 30°), at a frame rate of at least 129 Hz (43 Hz/angle). Angle switching occurred automatically, eliminating the need for probe repositioning. Additionally, a duplex mode image was acquired to facilitate differentiation between the lumen and plaque during subsequent analysis.
Blood pressure was measured twice on the right arm, before and after the ultrasound procedure, utilizing the manual Welch Allyn sphygmomanometer monitor (Hechingen, Germany). The recorded blood pressure was used to standardize the difference between systole and diastole to a 40-mm Hg difference for each participant. Because the amount of deformation is not only related to tissue stiffness but also to the force applied by the pulsating blood pressure to the tissue, all strains were normalized to a blood pressure difference (40-mm Hg) as this diastolic-systolic pressure differential is observed in healthy young individuals. The normalization process was integrated into the CUSI analysis. The complete protocol lasted approximately 15 min.
MRI scan
The MRI scan was conducted using a Siemens Healthcare 3.0T MAGNETOM Skyra MRI scanner (Erlangen, Germany) along with a Machnet Phased Array Carotid Coil (Eelde, Netherlands). Participants were positioned in a headfirst supine orientation. The scan protocol was based on the article of Saam et al. [ ], see Appendix I for the used scanning parameters.
Parameters
The participant characteristics age, gender and plaque location were recorded. Vulnerable plaques typically exhibit greater deformability compared to stable plaques, and this degree of deformation is quantified as strain. More deformation results in a higher percentage of strain. In the visual representation, higher strain values appear in yellow, while lower strain values are shown in red/black. Figure 1 provides a visual comparison of the strain distribution for each plaque composition group, alongside the corresponding MRI images.
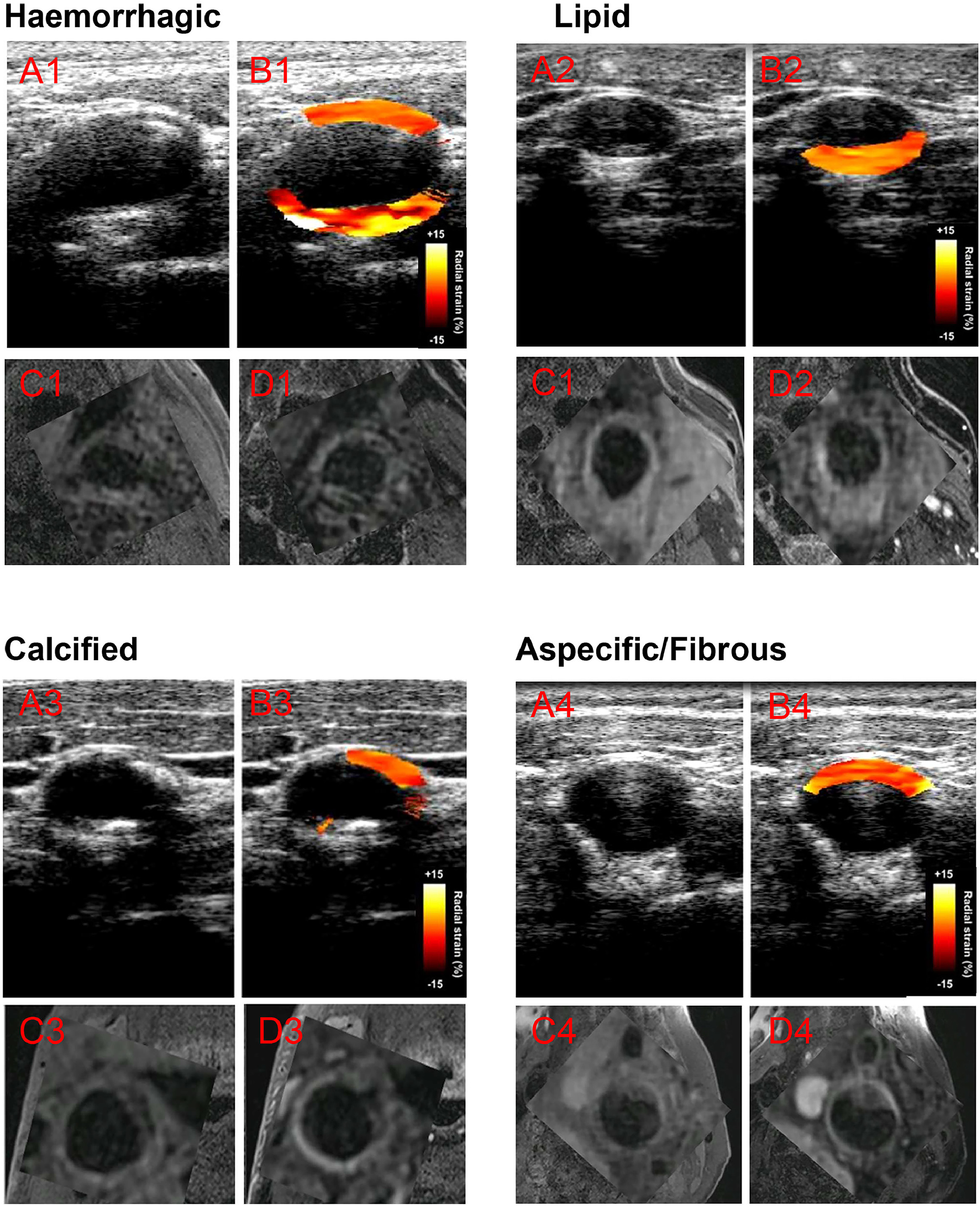
Differences in strain will be assessed using two distinct parameters: Strain med and Area high . Strain med represents the median value of deformation within a plaque. Area high denotes the percentage of strain values (representing an area) exceeding a strain level of 0.5%. The 0.5% threshold emerged after testing multiple values in a preceding study, wherein 0.5% scored the highest correlation with plaque phenotype [ ].
Compound ultrasound strain imaging analysis
The CUSI analysis was performed using a custom offline software developed at Radboudumc, utilizing MATLAB version R2015B from MathWorks (Natick, Massachusetts, USA). Firstly, ultrasound frames were identified that corresponded with the systolic and diastolic phases. In the second step, the pressure cycle (systole to diastole) was measured three times within an diameter curve on an m-mode image, and the cycle which visually showed the least motion, was selected for strain analysis, Figure 2 . The large global motion causes displacement estimates to be affected, which in turn negatively impacts the strain estimate. Therefore, the pressure cycle with the least motion was selected. The ROI was drawn manually, Figure 3 . The final step involved quantifying deformation of plaques within the pressure cycle, from systole to diastole, using an estimation algorithm, displacement compounding, tracking, rotation and 2-dimensional least-squares strain estimation. A comprehensive guideline for this analysis is provided in the article by Hansen et al. [ ].
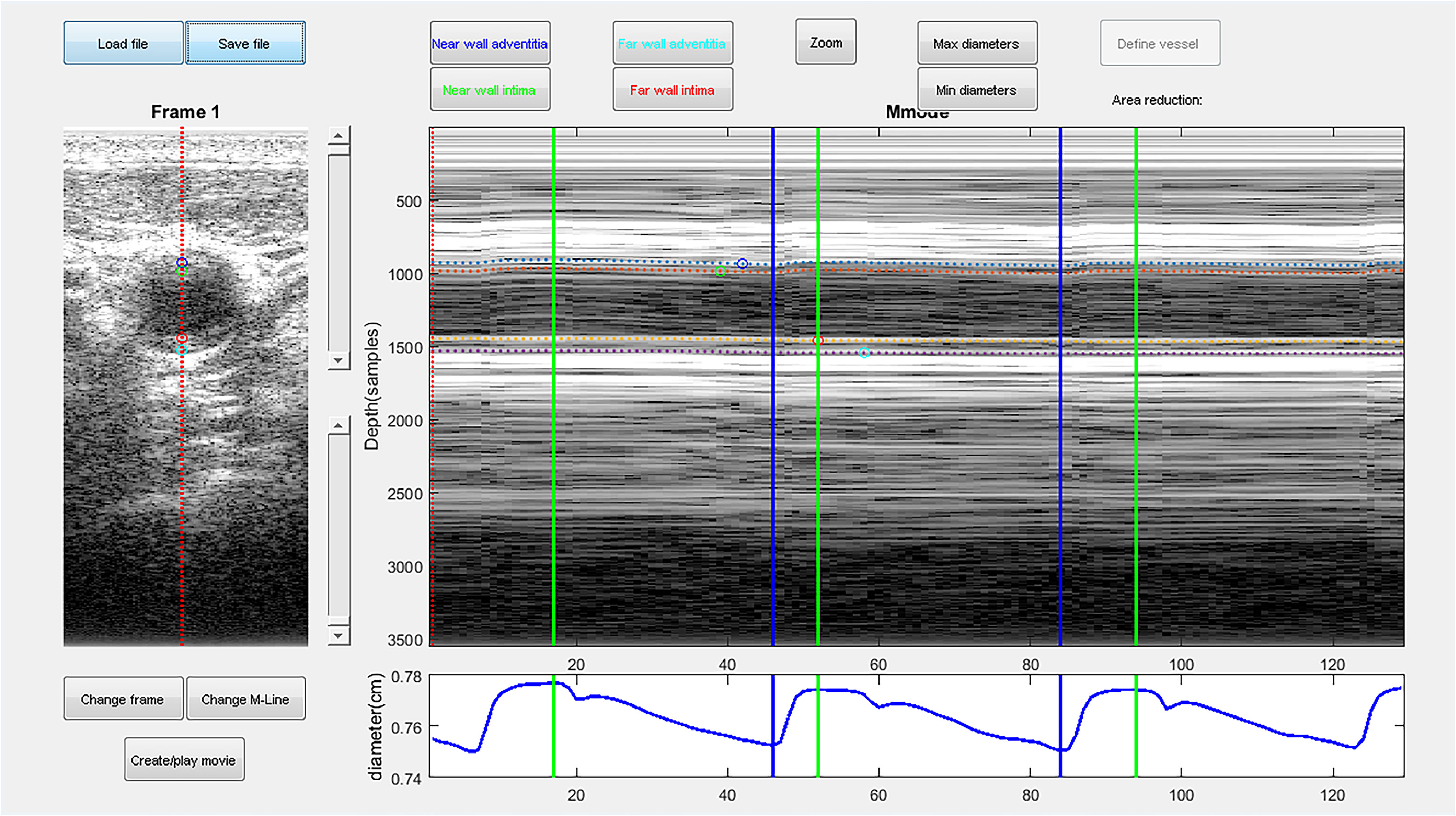
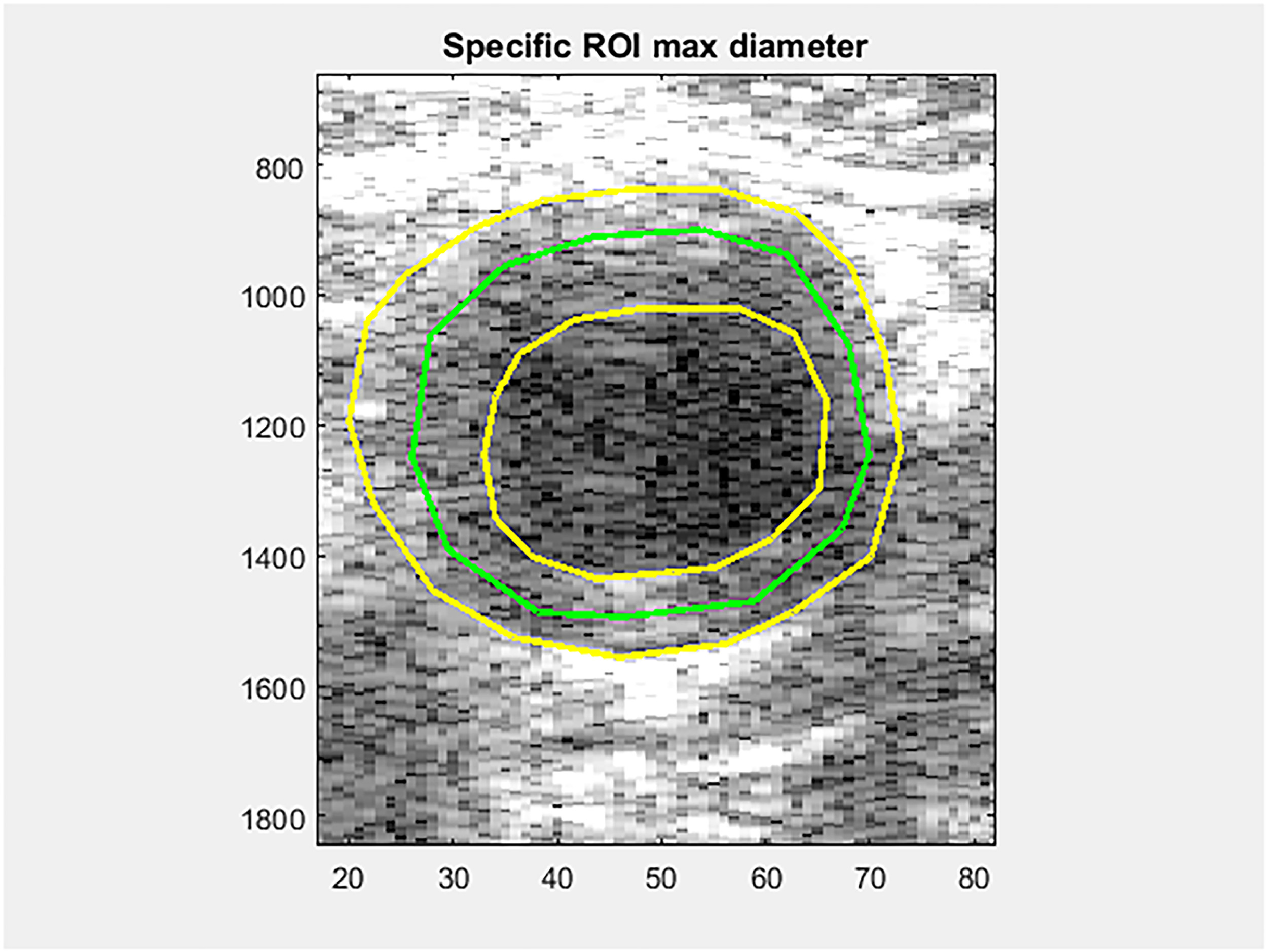
Statistical analysis
The data were analyzed using IBM SPSS Statistics (Version 29.0.0.0) [ ]. Descriptive statistics were presented. To compare Strain med and Area high among the four plaque categories, a Kruskal–Wallis was conducted. A Bonferroni correction was executed and a p value below 0.05 was considered statistically significant. The data were also dichotomized into stable and vulnerable categories, Figure 4 , and an independent samples test was performed, a p < 0.05 was considered significant.
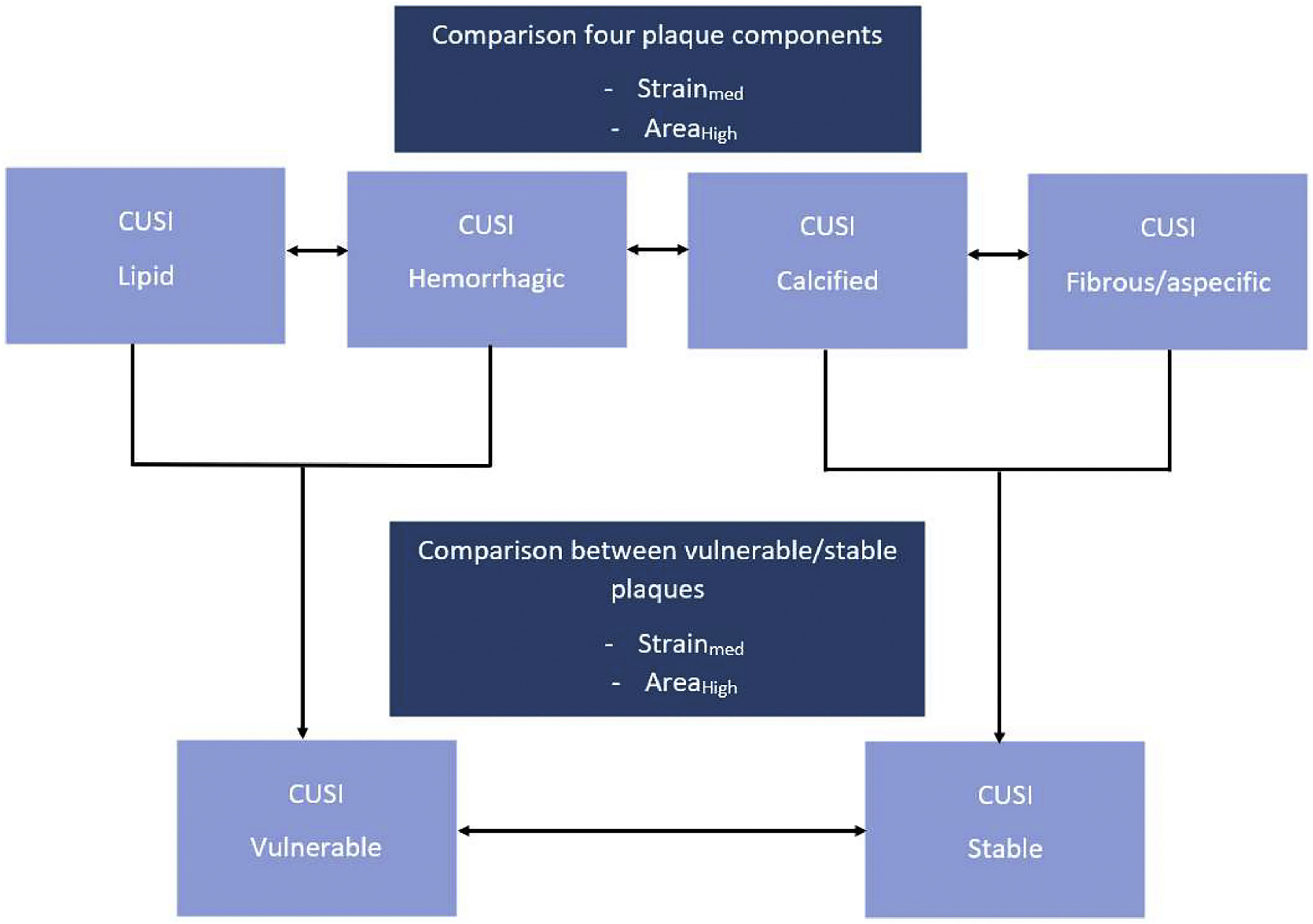
Results
A total of 76 plaques met the inclusion criteria and were eligible for inclusion in this study. Sixteen plaques were excluded due to a maximum plaque diameter of less than 2.0 mm. In two cases, a diameter curve could not be determined, and three plaques were not scanned using MRI. An additional four plaques were excluded due to inadequate image quality in either ultrasound or MRI, resulting from artifacts. Furthermore, incorporating a ring to exclude the lumen-plaque border led to the exclusion of another 9 plaques, Figure 5 . Ultimately, 42 plaques from 28 participants with a mean age of 70.6 y and a mean maximum plaque thickness of 3.2 millimeters were included in the study, see Table 2 for demographic information. According to the MRI classification, 18 plaques were fibrous/aspecific, 13 were calcified, seven were lipid and 4 were hemorrhagic. The majority of the plaques were located at the carotid bifurcation [ ], with 22 plaques in the left carotid artery and 20 in the right.
