Different types of malformations share a dilated vein of Galen, but only one of them is a true vein of Galen aneurysmal malformation (VGAM). The optimal window of opportunity for treatment is between 4 and 5 years of age, because this allows the child to grow and mature. Heart failure and hydrocephalus respond favorably to embolization. Cerebrospinal fluid ventricular shunting, if needed, should be performed after the embolization. The transvenous approach carries significantly elevated morbidity and mortality and is rarely indicated. Anatomic cure of the VGAM is not the main goal of treatment; the ultimate goal is control of the malformation to allow the brain to mature and develop normally.
The first description of a possible vein of Galen aneurysmal malformation (VGAM) was reported in 1895 by Steinheil (cited by Dandy in 1928), but that lesion was, in fact, a cerebral arteriovenous malformation (AVM) of the diencephalon draining into a dilated vein of Galen. The first attempts to treat a VGAM were recorded at the beginning of the last century and described an infant who presented with intracranial hypertension and subsequently underwent bilateral internal carotid artery ligations. In 1946, Jeager reported bilateral arteriovenous communications draining into a dilated vein of Galen, while Boldrey and Miller in 1949 treated two similar patients with arterial ligations. Only the last case seems to correspond to a true VGAM. Litvak in 1960, Raimondi in 1972, Clarisse in 1978, and Diebler in 1981 suggested the possible existence of true and false VGAMs.
More recently, anatomic and embryologic evidence has allowed us to categorize a VGAM as a specific choroidal malformation that is different from a cerebral AVM draining into a dilated but not malformed vein of Galen. Several investigators have established the relationship between VGAM and heart failure in neonates. In 1964 in a review of 34 patients, Gold described the following three consecutive clinical stages in patients with VGAMs: (1) neonates with cardiac insufficiency, (2) infants and young children with hydrocephalus and seizures, and (3) older children or adults with headaches and subarachnoid hemorrhage. In 1978, Amacher added a fourth group that included neonates and infants with macrocephaly and minimal cardiac symptoms. In an excellent review, Johnston and colleagues analyzed in an exhaustive fashion the clinical presentations of VGAMs. In 82 infants, he found the following symptoms: cerebrospinal fluid (CSF) disorders (70%), neurologic deficits (31%), and neurocognitive delay (12%). Among children aged 1 to 5 years, these symptoms occurred in 61%, 33%, and 5%, respectively. These clinical groups do not match those encountered by the authors in their practice. This difference may be due to the inclusion of VGAMs and cerebral AVMs in the previous analysis and the fact that the therapeutic approach did not take into account the window of opportunity for treatment of VGAMs.
Between October 1984 and 2002, 317 children less than 16 years of age with VGAMs were studied at the authors’ center. Of this cohort, 233 patients were treated with endovascular embolization (216 at the authors’ hospital). Of the 216 patients, 23 died despite being treated with embolization or due to procedure-related complications (10.6%). Twenty of the 193 surviving patients were severely retarded (10.4%), 30 were moderately retarded (15.6%), and 143 (74%) were neurologically normal on follow-up. Management of these patients has required the involvement of anesthesia, neurology, and pediatric neurosurgery teams at the authors’ center for the last 20 years. The contributions to the management of VGAM offered by the authors’ approach include the ability to identify the specific type of lesion, to anticipate the natural clinical course of the disease, and to institute treatment within the window of opportunity. The observations detailed in this review are derived from the authors’ experience .
Classification
Vein of galen aneurysmal malformation
A VGAM is a choroidal type of AVM. The lesion is supplied by the choroidal arteries. The choroidal shunt drains into a dilated vein, which Raybaud and colleagues first recognized as an ectatic vein, which is the median vein of the prosencephalon, the embryonic precursor of the vein of Galen. This embryonic vein drains only the choroidal system and does not connect with the deep venous system. It does not become the vein of Galen until communications with the thalamostriate and internal cerebral veins develop. In patients with VGAMs, these latter communications do not form, and the thalamostriate veins drain into the posterior and inferior thalamic (diencephalic) veins and secondarily join the anterior confluence, a subtemporal vein, or (more often) the lateral mesencephalic vein to open into the superior petrosal sinuses, which demonstrate a typical epsilon shape (“epsilon vein”) on the lateral angiogram ( Fig. 1 ) . Persistence of this venous arrangement is identical to that seen early in life before the 12th week of gestation. This pattern allows one to establish the time at which the malformation developed, and one may consider a VGAM to be the result of an error in the early phase of vasculogenesis. Prenatal diagnosis of VGAM may be made as early as the second trimester of intrauterine life by sonography or MR imaging. In the authors’ series of patients, 29.3% of cases were diagnosed in utero.
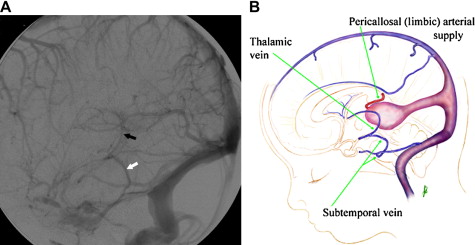
Vein of galen aneurysmal dilatation
Vein of Galen aneurysmal dilatations correspond to AVMs localized in the subpial space, either supra- or infratentorially, which drain into one of the usual tributaries of the vein of Galen and result in its overload and dilatation. This dilatation of the vein of Galen is variable and depends on the outflow obstruction due to stenoses or thrombosis where the vein joins the straight sinus. In this type of lesion, the vein of Galen is fully developed and drains not only the lesion but also the adjacent brain parenchyma. The arteriovenous shunts are located in the cerebellum, brainstem, or in the deep supratentorial territories that normally drain into the vein of Galen. Some tectal AVMs (supplied by transcerebral arteries) may mimic true choroidal VGAMs. Careful analysis of the arterial vascularization of lesions (always choroidal or subependymal in VGAMs) and of venous drainage (absence of connections with deep veins in VGAMs) allows one to arrive at the correct diagnosis. The prenatal diagnosis of a vein of Galen dilatation is uncommon, and the frequency of this lesion in neonates and infants is low. Clinical manifestations of vein of Galen dilatation include neurologic deficits, intracranial hemorrhages, or, in the very young, subsequent delayed psychomotor development. Epilepsy is not common owing to the deep localization of the lesions, and heart failure is also uncommon because these lesions are found in older children.
Vein of galen varix
This lesion refers to a varicose dilatation of the vein of Galen without an underlying arteriovenous shunt and drains normal brain parenchyma ( Fig. 2 ). Two types of vein of Galen varices are encountered in children. The first is a transient dilatation of the vein of Galen in neonates presenting with cardiac failure of a different origin. This venous dilatation persists for a few days postnatally and then disappears on follow-up ultrasound studies. The dilatation does not lead to any symptoms, and its disappearance parallels cardiovascular improvement. The second type of vein of Galen varix is associated with complex developmental venous anomalies draining an entire hemisphere into the deep venous system. This subtype of varix does not result in any specific symptoms, but, over time, some patients may show symptoms of venous ischemia due to insufficient venous drainage.
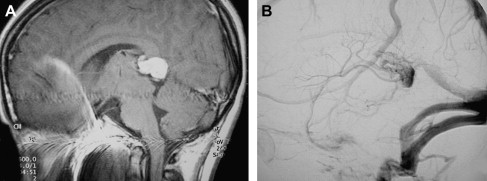
Dural vein of galen dilatation
These malformations belong to a group of acquired dural lesions that develop in the wall of the vein of Galen or at the venous-sinus junction . In nearly all patients, the straight sinus is thrombosed. These lesions occur almost exclusively in adults. In children, similar dural arteriovenous shunts develop secondarily to a complete thrombosis of the vein of Galen or of the falcine sinus. This thrombosis stimulates angiogenesis. At this level, angiogenesis is favored by pre-existing vasa vasorum in the vein of Galen wall.
Vein of galen aneurysmal malformation
Architecture and pathophysiology
The arterial supply of a VGAM usually involves all of the choroidal arteries, including anterior choroidal contributions ( Fig. 3 ). It may also receive significant contributions from the subependymal network ( Fig. 4 ) originating from the posterior circle of Willis. These arteries should be differentiated from transmesencephalic ones, because the involvement of the latter excludes the diagnosis of VGAM and indicates a tectal and not a choroidal AVM. The subependymal arteries pierce the floor of the third ventricle and run under the ependyma to join the choroid fissure, where they contribute to the blood supply of the lesion. Subependymal and thalamo-perforating contributions supply the shunt as accessories that are recruited by the sump effect of the venous drainage, and they usually disappear following proper occlusion of the most prominent shunts. Cerebellar arteries do not supply a VGAM except indirectly through their dural branches, which can be enlarged because they may participate in the supply to the vasa vasorum at the veno-dural junction. A persistent limbic arterial arch, which bridges the cortical branches of the anterior choroidal artery initially ( Fig. 5 ) and the posterior cerebral artery, is seen in nearly one half of neonatal VGAM cases. This limbic arch should be distinguished from subcallosal and subforniceal supply to the choroidal shunts. The arch regresses (matures) after obliteration of the VGAM by means of embolization.
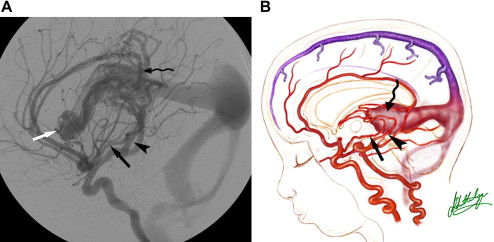
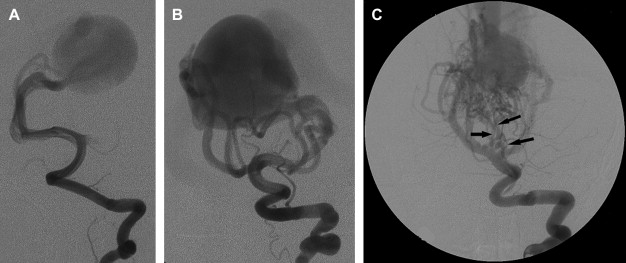
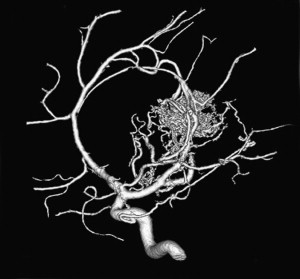
The nidus of a VGAM is usually located in the midline and receives bilateral supply. Generally, two types of angioarchitecture are encountered: choroidal and mural (see Fig. 4 ). The former corresponds to a primitive condition involving all of the choroidal arteries and an interposed network before opening into the large venous pouch. This condition is encountered in most neonates with severe symptoms. The latter type corresponds to direct arteriovenous fistulas within the wall of the median vein of the prosencephalon. The fistulas may be single or, more often, multiple and converge into a single venous chamber or into multiple venous chambers located along the anterior aspect of a venous pouch or along one dilated choroidal vein before reaching the venous pouch. The mural form is better tolerated clinically than the choroidal form. Mixed forms may occur, such as those with a choroidal nidus and high-flow fistulas located in the wall of the venous pouch.
The venous drainage of the choroidal shunt is always via a median prosencephalic vein and has no communication with the deep venous system. The choroidal veins are the embryonic tributaries of the median vein, and, potentially, the choroidal nidus may drain into the choroidal veins before they join the median pontomesencephalic vein. The connection between the medial pontomesencephalic vein and the choroidal veins may result in reflux into the pial venous system due to communications between the choroidal and striate veins ( Fig. 6 ). This arrangement is found in untreated older children with advanced VGAMs or in those who have undergone occlusion of their venous drainage without complete embolization of the arterial components. A connection with the choroidal veins must be sought out by angiography; their visualization is a contraindication to embolization by the venous route. Intraventricular bleeds and cerebral venous infarctions may occur after complete occlusion of a VGAM via the venous route if the lesion drained into the choroidal, subependymal, or striate venous systems. Reflux into the pial venous system leads to risks of seizures and bleeds similar to those associated with brain AVMs, which need urgent treatment, and alters the standard algorithm of management for VGAMs.
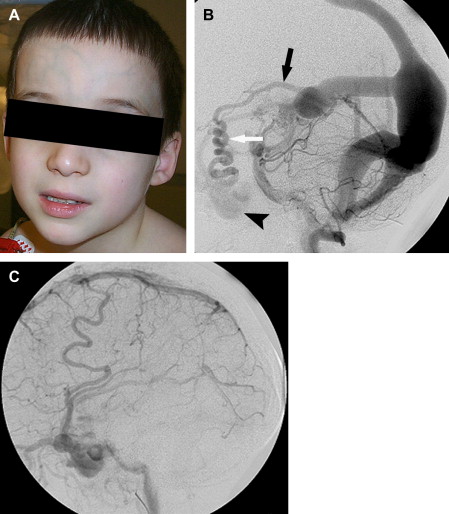
Dilatation of the median pontomesencephalic vein is variable and not related to the architecture of a choroidal or mural VGAM. This vein opens most commonly into a large falcine sinus; stenoses at the veno-sinus junction are occasionally encountered. These stenoses may increase the cerebral venous pressure, but, conversely, they improve heart function by decreasing overload.
The VGAM drains into the straight sinus and uses the posterior sinuses as a route of drainage. This implies that the deep cerebral venous system is drained by alternative pathways. As mentioned previously, an alternate route of drainage is via thalamic and subtemporal or lateromesencephalic veins, demonstrating typical epsilon shapes on the lateral angiogram . Visualization of the epsilon vein following contrast injection into the internal carotid arteries is a favorable sign and predicts a good neurologic prognosis.
The cavernous sinuses are another alternative route of venous cerebral drainage in patients who have a VGAM. During the prenatal period and the first months of life, the cavernous sinuses do not connect with the cerebral veins. At a later time, the cavernous sinuses mature and become able to “capture” blood from the sylvian veins, offering the brain a potential drainage pathway through the orbits, pterygoid plexuses, or inferior petrosal sinuses ( Fig. 7 ). Cerebral venous drainage via the ophthalmic veins increases the clinical visibility of the facial and scalp veins (see Fig 6 ). Dilatation of the facial veins in infants is an indication that cerebral venous drainage is occurring via the cavernous sinuses and is associated with a good prognosis. Rarely, facial venous congestion may result in nasal mucosal swelling and mild epistaxis.
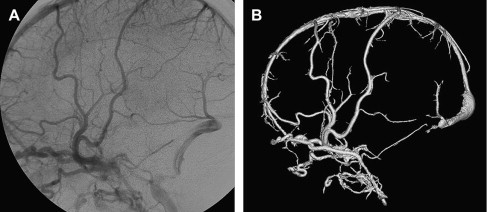
Maturation of the jugular bulbs and of the venous sinuses at the base of the skull occurs during the peri- and postnatal periods. This process of maturation encompasses remodeling of the torcular, regression of the embryonic occipital and marginal sinuses, and definite formation of the jugular bulbs . These maturation processes may be disturbed by the presence of high-flow shunts or by macrocephaly, which alters the normal development of the skull base. Processes that interfere with venous maturation at the base of the skull are also seen with high-flow pial arteriovenous fistulas in newborns and infants.
The prognosis for infants who have a VGAM depends largely on the evolution of the maturation of both sino-jugular junctions. Partial restriction of venous outflow into a jugular bulb together with drainage into the cavernous sinuses allows for normal cerebral venous drainage through the ophthalmic veins or the veins of the foramen ovale. If the stenosis of a jugular bulb is more pronounced, or if the jugular bulb is thrombosed, the VGAM and the brain use the cavernous sinuses to drain (see Fig. 6 ), leading to cerebral venous hypertension. This situation may be further aggravated in patients with bilateral sigmoid sinus thrombosis ( Fig. 8 ) Cerebral venous drainage via the cavernous sinuses may be uni- or bilateral. In some patients, bi-hemispheric drainage is accomplished via the sylvian veins draining into only one cavernous sinus. If this occurs, unilateral exophthalmos may develop if the venous drainage recruits the ophthalmic systems. Similarly, epistaxis may develop owing to engorgement of the nasal mucosa and the facial veins. Obstructed venous drainage, particularly a lack of recruitment of the cavernous sinuses, may lead to a “pseudophlebitic” appearance of the cortical veins on angiography ( Fig. 9 ). The combination of jugular bulb occlusion and venous pial reflux may exist for a few years, and some children with undiagnosed VGAM may present late with intracerebral hematomas or subdural or subarachnoid hemorrhage.
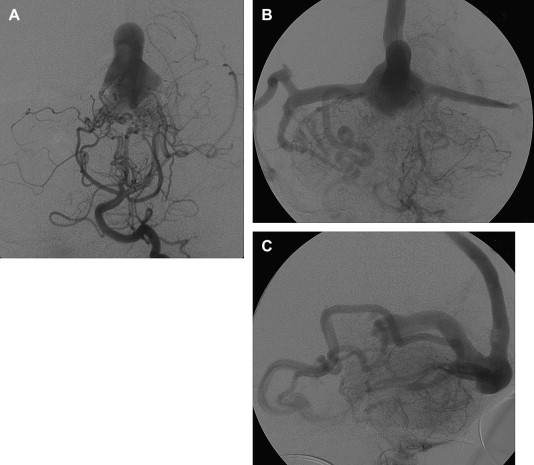
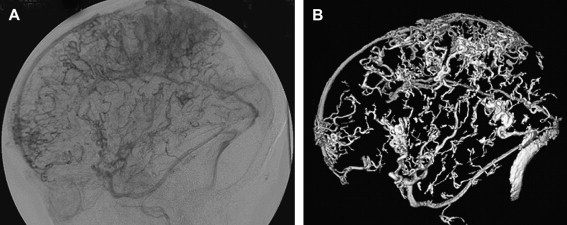
The infratentorial consequence of sinus occlusion is cerebral tonsillar herniation . Tonsillar herniation is secondary to cerebellar pial congestion and is only seen in its presence. It may disappear with correction of the arteriovenous shunts provided that the herniation has not existed for a long time ( Fig. 10 ).
Another fundamental aspect of neonatal and infant anatomy and CSF physiology is that the pacchionian granulations are not functional during the first months of life; therefore, CSF must be absorbed, at least partly, via the medullary veins . The association of this immaturity of the pacchionian granulations and venous hypertension arising from stenoses at the skull base results in an unbalanced hydrovenous state, leading eventually to hydrocephalus. Mechanical compression of the mesencephalic aqueduct should not be considered to be the primary cause of the hydrodynamic disorders at this age in VGAM patients; in reality, the aqueduct is patent in almost patients .
Untreated VGAMs evolve toward chronic venous ischemia manifested by the development of subcortical white matter calcifications and subependymal atrophy with ventricular dilatation ( Fig. 11 ). These calcifications reflect deep hydrovenous watershed failure, and they occur when the compliance of the medullary veins loses its normal ventricular-cortical gradient. These calcifications are usually bilateral and symmetrical, but they may be asymmetrical and mostly unilateral in shunted children (often on the side opposite to the shunt). Subependymal atrophy is primarily seen in the occipital regions. It may be dramatic, and it seems to be at least partially related to an abnormal postnatal development of the corpus callosum. Spontaneous thromboses of isolated cortical veins are also possible in untreated patients with VGAM.
Clinical manifestations
Clinical manifestations of patients who have VGAMs are mainly divided into those related to high output cardiac failure and those involving neurologic symptoms due to venous congestion and abnormal CSF flow. Their severity and tolerability are variable and are related to the angioarchitecture of the VGAM and the age of the child.
Cardiac manifestations
The cerebral arteriovenous shunts result in a larger volume of venous return to the right side of the heart and lead to dilatation of the right-sided chambers . At the same time, there is increased pulmonary blood flow and resultant pulmonary hypertension. All of this leads to failure of the left chambers (due to increased preload). Prenatal development of congestive heart failure is sonographically diagnosed by supraventricular extrasystole, tricuspid valve insufficiency, and tachycardia (over 200 beats per minute) and portrays a poor prognosis. In its most severe form, it may be associated with multiorgan failure and irreversible brain damage (“melting” brain). Termination of pregnancy should be considered in this scenario (performed in 3 of 93 of fetuses in the authors’ series). In the authors’ experience, 22% of children with a prenatal diagnosis have irreversible cerebral damage at birth and die soon thereafter. The remaining infants should undergo embolization at a time dependent on their individual response to medical cardiac treatment. Antenatal diagnosis is not an indication to perform early delivery, interruption of pregnancy, or cesarian section at term .
In the neonatal period, cardiac symptoms vary from mild overload to cardiogenic shock . In the mildest form, only cardiomegaly is present. During the neonatal period, there is a brief time (approximately the first 3 days of life) of clinical stabilization, but, shortly afterward, congestive heart failure worsens, then stabilizes, and finally improves with appropriate medical management. At birth, the transition between fetal and adult circulations imposes great demands on the myocardium, which already has a limited function because it contains a relatively high proportion of noncontractile fibers that, in combination with the relative immaturity of the sympathetic nervous system, explain the worsening of heart function during the first week of life. Symptoms depend on the volume of the cerebral arteriovenous shunts but, for the most part, are a reflection of the state of maturation of the cardiopulmonary system. Mild symptoms include feeding difficulties, lack of weight gain, and tachycardia. Chest radiographs show cardiomegaly, especially involving the right side. If the cardiac failure is mild, medical treatment generally suffices. Diuretics are initiated to reduce cardiac preload. One of the most important goals of medical treatment is to permit normal oral feeding, which ensures weight gain and allows the child to go home and to return at 5 months of age for embolization.
Not uncommonly, infections may lead to decompensation of the cardiac status, necessitating immediate treatment of the VGAM. Echocardiography is the best method to evaluate the cardiac consequences of the VGAM. In patients with severe heart failure, mechanical ventilation is needed a few hours after birth. This ventilation reduces oxygen needs and improves myocardial performance. Adjustments in the inspired fractional oxygen concentration are necessary to obtain an adequate arterial saturation. Diuretics and fluid restriction may also be used to decrease preload. Inotropic agents such as digoxin are used to increase cardiac contractility, but their benefits are not clear if the indices of myocardial function are normal. Theoretically, when left ventricular dysfunction owing to chronic volume overload occurs, digoxin should be beneficial. Dobutamine and dopamine may be used in severe situations to improve cardiac output. If heart failure cannot be controlled with these measures, embolization of the cerebral arteriovenous shunts should be performed in the neonatal period.
Recently, the authors have identified a group of VGAM patients with severe heart failure and suprasystemic pulmonary hypertension who are resistant to nitric oxide and also have a lack of a clear-cut response to embolization. The exact treatment algorithm in these patients is yet to be determined. Recent studies suggest that management from the time of birth in the intensive care unit with mechanical ventilation and oxygen administration interferes with postnatal vascular lung maturation. This lack of normal pulmonary vascular remodeling may persist even after correction of the increased preload. Before starting treatment of the arteriovenous shunts, the patient’s neurologic status needs to be assessed, and the cranial circumference, an electroencephalogram, and brain MR imaging need to be obtained.
The authors have developed a specific scoring system that takes into account neurologic, cardiac, respiratory, renal, and hepatic functions ( Table 1 ). A total score of less than 8 is evidence of a poor systemic or neurologic outcome and may be an indication to withhold therapy. In the authors’ series of patients, 25 of 140 newborns were not treated owing to severe brain damage, and 17 of 140 were not treated owing to multiorgan failure. Together, both groups of patients represent 17% of all infants and 30% of newborns managed at the authors’ center. A score between 8 and 12 indicates a normal neurologic status but heart failure that is not responsive to medical treatment and the need for mechanical ventilation. Emergency embolization of the VGAM is mandatory in these patients. The goal of embolization is to reduce the arteriovenous shunting by one third, a proportion that results in improvement of systemic symptoms. Post embolization Doppler imaging reveals rapid improvement of cardiac and pulmonary functions, which allows for decreases in sedation and extubation of the infant. Mortality remains high, and neurologic scores are lower than in infants treated at 5 months of age. Neurologic assessment is difficult in neonates. Current methods to evaluate brain morphology and function do not permit identification of some injuries that may occur pre- or postnatally and do not become clinically evident until later. In the authors’ series of over 140 neonates (including prenatally diagnosed cases), only 23 (among the 95 patients who were thought to have an acceptable neurologic prognosis) needed to be embolized during the neonatal age. One half of these patients died despite treatment.
Points | Cardiac function | Cerebral function | Respiratory function | Hepatic function | Renal function |
---|---|---|---|---|---|
5 | Normal | Normal | Normal | — | — |
4 | Overload, no medical treatment | Subclinical isolated EEG abnormalities | Tachypnea, finishes bottle | — | — |
3 | Failure, stable with medical treatment | Nonconvulsive intermittent neurologic signs | Tachypnea, does not finish bottle | No hepatomegaly, normal function | Normal |
2 | Failure, not stable with medical treatment | Isolated convulsion | Assisted ventilation, normal saturation FiO 2 <25% | Hepatomegaly, normal function | Transient anuria |
1 | Ventilation necessary | Seizures | Assisted ventilation, normal saturation FiO 2 >25% | Moderate or transient hepatic insufficiency | Unstable diuresis with treatment |
0 | Resistant to medical treatment | Permanent neurologic signs | Assisted ventilation, desaturation | Abnormal coagulation, elevated enzymes | Anuria |
A neonatal score between 13 and 21 allows one to delay the embolization of the malformation until the infant is 5 months of age. During the waiting period, the infant is followed up clinically, with special attention to cranial circumference because an increase may be the first indication of an unbalanced intracranial fluid state. Likewise, close neurologic and cardiac assessments are indicated to detect early symptoms arising in these organs. The authors suggest that MR imaging studies be obtained at birth and then at 3 months of age to evaluate myelination and morphologic cerebral status. Two thirds of patients referred to the authors did not need to be treated immediately but were able to wait until the optimal therapeutic window (5 months of age).
Unlike in prenatal patients and neonates, congestive heart failure is almost never the presenting symptom in infants, nor does it worsens at any age if already present. An increased cardiac index is often noted when the diagnosis of VGAM is made because of macrocrania. Most children need continuous treatment with diuretics until the time of embolization. After the first embolization, weaning from these medications is nearly always possible.
Neurologic manifestations
Intracranial venous hypertension resulting from the VGAM in combination with anatomic (the status of the cavernous sinuses and the degree of development of the base of the skull) and physiologic features (nonfunctioning pacchionian granulations) of the neonatal brain are the cause of neurologic deterioration . Cerebral maturation requires a normal venous system capable of maintaining a fluid balance among the intracellular, extracellular, intraventricular, and intravascular spaces. Symptoms in fetuses or neonates are generally the result of intracranial venous congestion or hemodynamic alterations owing to heart failure during intrauterine life and reflect early brain damage. MR imaging ( Fig. 12 ) may show focal white matter lesions or diffuse destruction of the brain (the melting brain), which is generally associated with severe cardiac insufficiency. These patients have low clinical scores, which is further evidence that aggressive treatment may not be indicated. In the authors’ series, 25 of 140 patients showed zones of encephalomalacia, and 17 had low scores, both leading to a decision not to treat.
