PART II Brain Arteriovenous Malformations A 42-year-old woman presents with multiple episodes of generalized tonic-clonic seizures that started recently. Despite medication, she experiences up to two seizures per week. Given the adult onset of her seizures, enhanced CT is performed, followed by MRI and angiography. Fig. 15.1 (A) Contrast-enhanced axial CT demonstrates a dilated vessel in the left parietal region. (B) Axial T1-weighted contrast-enhanced and (C) T2-weighted MR images confirm the presence of an abnormal vascular structure at the left parietal region, seen as flow voids without evidence of a classic caput medusae. Dynamic CTA in (D) early arterial, (E) late arterial, and (F) venous phases show early filling of a cortical vein, indicating an AV shunt. CT AND MRI Outside contrast-enhanced CT of the brain demonstrated a dilated vessel in the left parietal region that was also shown by MRI. Because no classic caput medusae was present to suggest a possible developmental venous anomaly, a micro-arteriovenous malformation (AVM) was suspected. CTA demonstrated filling of a cortical vein during the arterial phase, indicating an AV shunt (Fig. 15.1). Following a complete six-vessel angiographic evaluation, a single AVM was identified in the left parietal region fed solely by the anterior parietal branch of the middle cerebral artery (MCA) and draining into two superficial cortical veins (central and parietal veins) with a long pial course along the surface of the brain. There was evidence of venous ectasia in the central vein proximal to a focal stenosis as the vein reached the superior sagittal sinus. The nidus of the AVM was small (Fig. 15.2). Small pial AVM with venous ectasia and stenosis Following the diagnostic angiography, a 5F multipurpose catheter was placed in the distal infrapetrous internal carotid artery (ICA) and continuously flushed. A flow-directed microcatheter with a steam-shaped 90-degree curve was introduced into the MCA and advanced into the anterior parietal branch of the MCA. Given the distal tortuosity, a guidewire was introduced into the microcatheter and advanced without exiting the microcatheter to make it easier to push the system and position the microcatheter far distally, just proximal to the nidus as verified by a subsequent microcatheter injection. After the microcatheter had been flushed with the glucose solution, a mixture of 1 mL of glue with 1 mL of Lipiodol was injected that allowed complete occlusion of the nidus without significant venous penetration. The microcatheter was removed, and post-embolization angiogram demonstrated complete occlusion of the AVM without evidence of a missing arterial branch or compromise of the venous flow (Fig. 15.3). Immediately after the procedure, 4 mg of dexamethasone was administered. Fig. 15.2 DSA. Left ICA angiogram in (A) AP and (B) lateral views in arterial phase reveals a small AVM in the left parietal region, supplied by the anterior parietal branch of the left MCA, draining into two superficial cortical veins. A long pial course of the draining veins with a focal stenosis before entry into the superior sagittal sinus is noted. Although there is little discussion about the necessity of treating pial AVMs that have bled because of the significant risk for rebleeding, pial AVMs that have not bled must be further analyzed to select those patients in whom therapy is indicated (i.e., in whom the therapeutic risk is lower than the natural history risk). In our practice, we first classify unruptured AVMs according to their pathologic mechanism and angioarchitecture because hemorrhage is not the only way an AVM may become symptomatic. Second, we try to determine the natural history risk by a careful angioarchitectonic evaluation of the AVM in relation to risk factors for future hemorrhage. Epilepsy is one of the more common presenting symptoms of AVMs, and it may be related to (1) venous congestion (due to a long pial course of a superficial draining vein, as in this case), (2) mass effect of a venous pouch, or (3) perinidal gliosis. Venous outflow obstructions (especially if already associated with venous ectasia, as in this case) are regarded in our practice as a possible risk factor for future hemorrhage related to an increase in intranidal pressure. Fig. 15.3 (A) Superselective microcatheter injection and (B) plain radiography in lateral view demonstrate deposition of the glue cast within the AVM nidus. (C) Left ICA angiogram in lateral view after embolization shows complete occlusion of the AVM. MRI/MRA CONSERVATIVE OR MEDICAL MANAGEMENT Published Literature on Treatment Options The treatment of incidentally discovered pial AVMs of the brain is controversial. Little is yet known about their natural history, their pathologic mechanisms, and the efficacy and risks of some of the proposed treatments (e.g., Onyx). The annual hemorrhagic risk for unruptured brain AVMs varies between 1 and 5%. It is well-known that only complete exclusion of an AVM can eliminate the risk for hemorrhage, and that the rates of curative endovascular embolization of AVMs with an acceptable periprocedural risk are ~20 to 50%. However, these values do not take into account the individual patient for whom the treatment plan has to be tailored. In the present example, a defined pathologic mechanism could be linked to the patient’s symptoms and explained by the angioarchitecture, which could be easily treated with a high probability of complete cure, given the type of feeding artery (terminal feeder), the size of the nidus, and its accessibility. Therefore, treatment strategies, including conservative management as well as all three treatment options and combinations thereof, are discussed in our practice within a multidisciplinary team and tailored to the individual patient. PEARLS AND PITFALLS__________________________________________________ Krings T, Geibprasert S, Terbrugge K. Interventional therapy of brain and spinal arteriovenous malformations. In: Mast et al, eds. Stroke. Philadelphia, PA: Lippincott Williams & Wilkins. In press. Ledezma CJ, Hoh BL, Carter BS, Pryor JC, Putman CM, Ogilvy CS. Complications of cerebral arteriovenous malformation embolization: multivariate analysis of predictive factors. Neurosurgery 2006;58(4):602–611, discussion 602–611 Willinsky RA, Goyal M, terBrugge K, Montanera W, Wallace MC, Tymiansky M. Embolization of small (< 3cm) brain arteriovenous malformations. Correlation of angiographic results to a proposed angioarchitecture grading system. Interv Neuroradiol 2001;7:19–27 A 21-year-old student presents with increasing memory problems, a decreased attention span, and progressive left hemiparesis and clumsiness of his left hand. On physical examination, he has difficulty performing voluntary motor acts with his left arm and leg, and a hemiapraxia is observed, although the muscle tone is maintained. The function of his left side is not affected in serial automatic motor activities (dressing and walking). A supplementary motor syndrome is therefore diagnosed. In addition, his short-term memory is reduced. MRI and CT perfusion are performed. Fig. 16.1 (A) T2-weighted MRI demonstrates an abnormal tangle of flow-void structures at the right parasagittal region, corresponding to an AVM. (B) Perfusion CTA in a parasagittal view shows a decreased MTT (blue) within the AVM caused by rapid shunting and a markedly increased MTT (yellow and orange) within the SMA and anterior cingulate gyrus region. MRI demonstrated a right parasagittal central arteriovenous malformation (AVM) with leptomeningeal recruitment of middle cerebral artery (MCA) branches. No evidence of recent or remote hemorrhages was found, and no perilesional gliosis was visualized. CT perfusion demonstrated a decreased mean transit time (MTT) within the brain AVM due to a rapid shunt. Most interestingly, a marked increase in the MTT within the supplementary motor area (SMA) and anterior cingulate gyrus region was noted, indicating a prolonged AV transit time due to delayed outflow that resulted in venous congestion remote from the AVM (Fig. 16.1). Injection in the right internal carotid artery (ICA) demonstrated an AVM that was fed by the pericallosal and callosomarginal arteries. In addition, significant leptomeningeal indirect collaterals were visualized that reconstituted the distal anterior cerebral artery (ACA) territory as well as the AVM, indicating a significant sump effect of the AVM (which suggests a high-flow shunt within the AVM nidus). Delayed venous return was visualized in the anterior midline brain parenchyma, indicating venous congestion of the SMA and anterior cingulate gyrus (Fig. 16.2). Large pial AVM with high-flow shunt and venous congestion associated with progressive neurologic deficits Fig. 16.2 DSA. Left ICA angiogram in (A) AP and (B) lateral views reveals an AVM supplied mainly by the pericallosal and callosomarginal arteries of the ACA, with significant indirect leptomeningeal collaterals from the MCA branches. Following diagnostic angiography, a 5F multipurpose catheter was placed in the distal infrapetrous ICA and continuously flushed. A flow-directed microcatheter with a steam-shaped 90-degree curve was introduced into the ACA and advanced into the proximal A2 segment, where microcatheter injections revealed the anticipated fistulous component of the AVM. Because of the high flow in the lesion, no guidewire was required. The microcatheter was placed ~5 mm proximal to the fistula, as verified by a subsequent microcatheter injection with the tip of the catheter pointing against the wall of the artery. After the microcatheter had been flushed with the glucose solution, a mixture of 2 mL of glue with 0.2 mL of Lipiodol and half of a vial of tantalum powder was injected that allowed complete occlusion of the fistulous component of the AVM nidus. Following microcatheter removal, control runs demonstrated significantly altered hemodynamics, with reduced flow through the AVM. Immediately after the procedure, 4 mg of dexamethasone was administered. Follow-up CT perfusion demonstrated improvement in the perfusion of the SMA and anterior cingulate gyrus (Fig. 16.3). The patient’s neurologic deficits decreased significantly, and he was scheduled for subsequent radiosurgery. Fig. 16.3 (A) Superselective microcatheter injection reveals a fistula within the AVM nidus. (B) Plain radiography demonstrates the location of the glue cast, and left ICA angiogram in (C) AP and (D) lateral views after embolization shows significantly decreased flow through the AVM. There is better perfusion of the SMA and anterior cingulate gyrus region on the follow-up CT perfusion (E). Venous congestion may be related to decreased outflow (in the case of venous stenoses) or increased inflow (in the case of fistulous AVMs) and can be associated with progressive neurologic symptoms. Even if treatment is not complete following the first embolization session, endovascular treatment may play a role in reducing the shunt to relieve clinical symptoms before definitive treatment of the AVM. The strategy of defining a target (i.e., a pathologic mechanism that is related to a clinical symptom as verified by imaging of the angioarchitecture) before treatment has been termed partial targeted embolization. The role of endovascular treatment is therefore to “secure” the AVM, stabilize or ameliorate the symptoms, or reduce the size of the AVM so that additional therapies that may take longer to be effective are made safer. In the present case, the perfusion data, the recruitment of leptomeningeal collaterals from a different vascular territory (MCA) to reconstitute the distal ACA territory, and the progressive neurologic symptoms strongly suggested a fistulous compartment within the AVM, which could then be regarded as the target of embolization. See also Case 15. See also Case 15. CONSERVATIVE OR MEDICAL MANAGEMENT Published Literature on Treatment Options Management strategies will be influenced by local preferences and capabilities; however, results presented in the literature suggest the following therapeutic strategies. Small, superficially located AVMs (nidus volume < 10 mL) are best operated on; however, a presurgical attempt to cure by embolization may be warranted unless the angioarchitecture is unfavorable. In single feeder-single compartment AVMs or AVMs with large fistulous components, embolization should be the first therapy of choice. Deep-seated, small AVMs should be treated by radiosurgery unless they are suitable for cure by embolization or have angioarchitectonic risk factors (fistulae, intranidal aneurysms, venous stenoses). Large AVMs (nidus volume > 10 mL) may benefit from partial embolization followed by radiosurgery or surgery, depending on the location and angioarchitecture. Finally, very large AVMs (nidus volume > 20 mL) will present a high treatment risk with all modalities and are therefore best managed conservatively. As in the present case, multimodality treatment with endovascular therapy preceding either surgery or radiosurgery is in most centers the therapy of choice. Although the risks of two treatment modalities are combined, there is consensus in the literature that in specific and selected cases, the risks of two treatment modalities are smaller for an individual patient than the risks of a single-modality treatment. PEARLS AND PITFALL__________________________________________________ Back AG, Vollmer D, Zeck O, Shkedy C, Shedden PM. Retrospective analysis of unstaged and staged gamma knife surgery with and without preceding embolization for the treatment of arteriovenous malformations. J Neurosurg 2008;109(Suppl):57–64 Hartmann A, Mast H, Mohr JP, et al. Determinants of staged endovascular and surgical treatment outcome of brain arteriovenous malformations. Stroke 2005;36(11):2431–2435 Krings T, Hans FJ, Geibprasert S, Terbrugge K. Partial targeted embolization of brain arteriovenous malformations. Eur Radiol June 11, 2010 [Epub ahead of print] Ledezma CJ, Hoh BL, Carter BS, Pryor JC, Putman CM, Ogilvy CS. Complications of cerebral arteriovenous malformation embolization: multivariate analysis of predictive factors. Neurosurgery 2006; 58(4):602–611, discussion 602–611 Natarajan SK, Ghodke B, Britz GW, Born DE, Sekhar LN. Multimodality treatment of brain arteriovenous malformations with microsurgery after embolization with onyx: single-center experience and technical nuances. Neurosurgery 2008;62(6):1213–1225, discussion 1225–1226 Söderman M, Andersson T, Karlsson B, Wallace MC, Edner G. Management of patients with brain arteriovenous malformations. Eur J Radiol 2003;46(3):195–205 Yuki I, Kim RH, Duckwiler G, et al. Treatment of brain arteriovenous malformations with high-flow arteriovenous fistulas: risk and complications associated with endovascular embolization in multimodality treatment. J Neurosurg Oct 16, 2009 [Epub ahead of print] A 28-year-old right-handed woman has had migraine headaches for 3 months, which have progressed in intensity over the last weeks. In addition, she experiences transient ischemic attack (TIA)-like symptoms (motor aphasia) that are not related to the headaches and are not associated with a loss of consciousness. Outside imaging reveals a right parietooccipital arteriovenous malformation (AVM). Functional MRI and CT perfusion are performed. Fig. 17.1 (A,B) Functional MRI during speech production. (C,D) Perfusion MRI in axial cuts. MRI, MR PERFUSION, AND FUNCTIONAL MRI MRI demonstrated a large right parieto-occipital AVM without evidence of remote hemorrhage or perilesional gliosis. Functional MRI during speech production revealed bilateral representation of the patient’s speech areas in the opercular portion of the inferior frontal gyrus (Broca’s area). Perfusion MRI revealed a decreased mean transit time not only within the parieto-occipital AVM location, indicating a shunt, but also remote from the AVM in the previously identified speech areas, suggesting arterial steal as the most likely explanation of the patient’s recurrent TIA-like symptoms (Fig. 17.1). njection in the right ICA demonstrated an AVM that was fed by the angular and the temporo-occipital artery in a terminal fashion. A flow-related aneurysm was present at the bifurcation of the angular artery proximal to the nidus proper. A single massively enlarged posterior parietal vein drained the AVM without signs of distal venous stenosis. A dramatic reduction in flow in the remainder of the middle cerebral artery (MCA) branches on angiography suggested significant arterial steal into the AVM. The AVM nidus measured 19.4 mm3 (Fig. 17.2). Large pial AVM with prenidal flow-related aneurysms and arterial steal Fig. 17.2 DSA. Right ICA injection in (A) AP and (B) lateral views, arterial phase. The arrows point toward the flow-related prenidal aneurysm. Following diagnostic angiography, a 5F multipurpose catheter was placed in the distal infrapetrous internal carotid artery (ICA) and continuously flushed. A flow-directed microcatheter with a steam-shaped 45-degree curve was introduced into the MCA and advanced into the proximal angular artery. With the aid of a micro-guidewire that had a short, sharp curve, we passed the arterial segment harboring the prenidal aneurysm. Three pedicles were treated with a mixture of 1 mL of glue and 1.2 mL of Lipiodol, which achieved a significant reduction in size and flow. Immediately after the procedure, 4 mg of dexamethasone was administered. Follow-up angiography after 3 months, before radiotherapy, demonstrated a significant reduction in the size of the AVM and complete regression of the flow-related prenidal aneurysm (Fig. 17.3). The patient underwent radiosurgery, resulting in obliteration of the AVM after 2 years. Following embolizaton, the patient no longer experienced headaches and TIA-like symptoms. The purpose of embolization before radiosurgery is either size reduction or targeted embolization of symptomatic or angioarchitectonic weak points. In the present case, the purpose of embolization was (1) size reduction and (2) reduction of the arterial steal; the prenidal flow-related aneurysm was not considered a target. Intranidal aneurysms and pseudoaneurysms in the setting of an acutely ruptured AVM are discussed in Case 18. Proximal flow-related aneurysms in unruptured AVMs by themselves do not seem to alter the natural history of the AVM, and although they may be a marker of generalized increased vessel fragility, they are not a priority target of treatment. Rupture of remote flow-related aneurysms following embolization has not been observed in our experience, and flow-related aneurysms typically regress, especially if the aneurysm is close to the nidus and more than 50% of the AVM is obliterated. For embolization before radiosurgery, the following points have to be kept in mind: The size of the AVM determines the dose given and therefore the risk for adverse effects of radiosurgery (i.e., the smaller the AVM, the higher the rate of complete obliteration without side effects). On the other hand, large amounts of radiopaque liquid embolic agent make definition of the residual target difficult and may even reduce the radiation dose delivered because of absorption within the embolic agent. Therefore, a multidisciplinary approach before embolization is of great importance. Irrespective of these considerations, an intranidal deposit of the liquid embolic agent is mandatory to reduce the potential for delayed reopening, which has occurred in embolized and irradiated AVMs. Fig. 17.3 DSA. Right ICA injection in (A) AP and (B) lateral views, arterial phase, at follow-up after 3 months. The nidus size is markedly reduced, and there is complete regression of the flow-related aneurysm. MRI AND CT
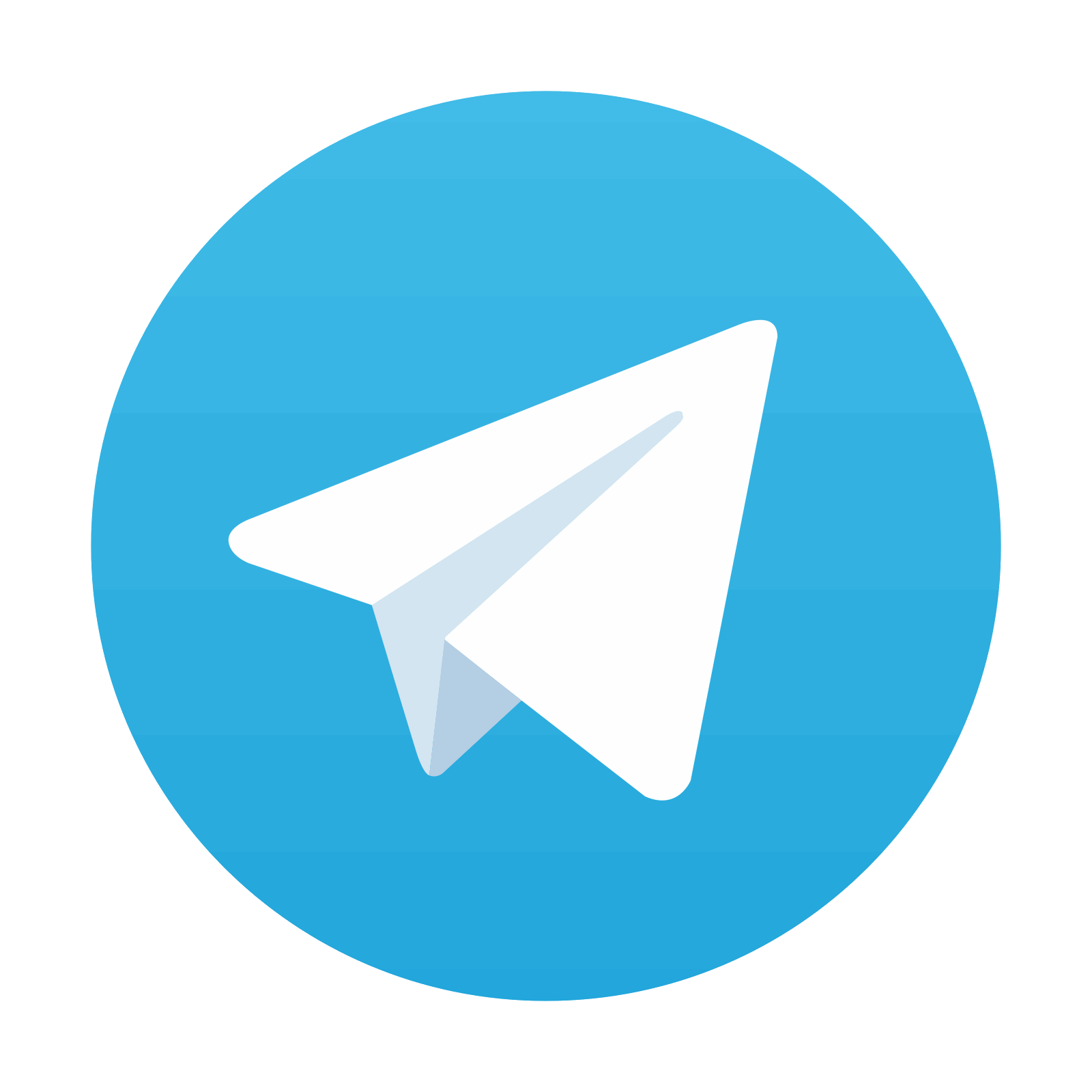
Stay updated, free articles. Join our Telegram channel

Full access? Get Clinical Tree
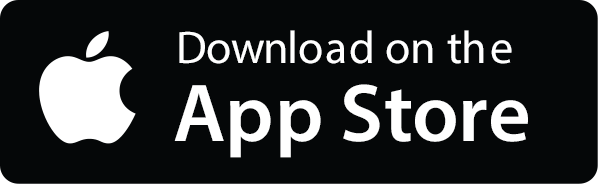
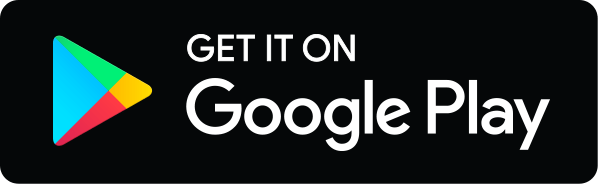