1 Venous Anatomy and Physiology and Epidemiology of Venous Disorders
Summary
Venous anatomy and physiology, and the epidemiology of venous disorders are critical aspects of the knowledge base of any interventional radiologist caring for patients with venous disease. Venous disorders are a substantial component of interventional radiology clinical practices, with interventional radiologists performing a variety of procedures in multiple venous territories. This chapter provides a detailed overview of venous anatomy and embryology as a basis for understanding collateral pathways, which are described in detail. There is also a discussion of venous physiology, as well as an overview of the epidemiology of various venous disorders cared for by the interventional radiologist.
1.1 Introduction
Venous anatomy and physiology have been topics of great interest for centuries. As minimally invasive image-guided procedures have become increasingly common and complex, a thorough understanding of venous anatomy and physiology is essential for interventional radiologists to ensure effective treatment and management of venous disease.
The venous system is an extensive network of vessels with the primary function of returning blood to the heart for reoxygenation and recirculation. Veins are equally important and complex as their arterial counterparts, but are distinct from both anatomic and physiologic perspectives. The manifestations and treatment of venous pathology are also different from those relevant to arterial disorders. Veins are more than blood return conduits; they are dynamic structures responsive to many anatomic and physiologic changes. Veins play essential roles in hemodynamics and contain approximately 60 to 80% of the blood volume at any given time. 1 , 2 , 3
Histologically, vein walls are composed of three layers: the intima, media, and adventitia. The intima is uniformly comprised of a single layer of endothelial cells. Underlying the intima is the internal elastic lamina, a thin layer of connective tissue, present predominantly in larger veins. The internal elastic lamina may be incomplete or absent in small- to medium-sized veins. The media is a layer of smooth muscle cells that varies in thickness based on location and function. Interspersed within the media are connective tissue substances such as collagen and elastin. The adventitia is the outer layer comprised of loose connective tissue, the vasa vasorum, and nerve fibers. 2 , 4
While these layers are similar to the anatomic layers of arteries, the relative composition of each layer differs. Arteries are typically thick-walled vessels due to a more abundant media layer. The arterial media contains many more smooth muscle cells and elastic fibers, allowing the large elastic arteries and medium muscular arteries to accommodate higher blood pressures and pulsatile flow from the heart. Veins have a thinner media layer, decreasing wall thickness, and increasing vessel diameter. Therefore, veins at each anatomic location have a much larger capacitance than their companion arteries, and hold the majority of the blood volume in the vascular system. Regional composition of veins also varies based on vessel size and function. Valves are structures unique to veins and are essential in normal venous physiology. Valves are connective tissue infoldings of the intimal layer covered on both sides by endothelium. The primary functions of valves are the prevention of reflux and retrograde blood flow. Valves are predominantly found in larger veins and lower extremity veins. 2 , 4
1.2 Embryology
Embryologic development of the venous system occurs in multiple stages. By the third week of gestation, biochemical signals induce differentiation of progenitor cells such as hemangioblasts and angioblasts. During stage 1, the undifferentiated stage, primitive channels of endothelial cells form a capillary network. The retiform stage, stage 2, is marked by the formation of large plexiform structures. The maturation stage, stage 3, consists of larger primitive vascular channels that begin differentiating into arteries and veins. 3 , 4 Differentiation was historically thought to be driven by differences in blood flow. However, specific molecular markers also have important roles in vascular differentiation. 5 For example, the attachment of vascular endothelial growth factor (VEGF) to angioblasts determines whether the angioblast will differentiate into an artery or a vein. VEGF binds to the VEGFR2-NP-1 complex in arterial development, while VEGF binds only to VEGFR2 for venous development. 6
By the fourth week of gestation, venous networks begin to form. The cardinal system drains the body of the embryo. The vitelline (omphalomesenteric) system drains the yolk sac as well as the primitive gastrointestinal tract, and later becomes the hepatic portal venous system. The umbilical venous system carries oxygenated blood from the placenta to the embryo. 6
The venous system of the trunk begins as paired symmetric structures. As the embryo develops, the left-sided venous structures regress and the right-sided vessels persist to form the major systemic drainage pathways. In the systemic circulation, the bilateral anterior and posterior cardinal veins drain the cranial and caudal structures of the embryo, respectively. The primitive veins of the neck and thorax develop via the anterior cardinal network, which eventually mature and drain into the superior vena cava (SVC). Cranial and facial structures drain into the proximal anterior cardinal veins. Venous plexuses of the bilateral upper limbs fuse to form the subclavian veins and then drain into the anterior cardinal veins. By week 8, an anastomosis forms between the left and right anterior cardinal veins, channeling blood to the right. The conduit becomes the left brachiocephalic vein. Cranial to this anastomosis, the anterior cardinal veins become the internal jugular veins. The anterior and posterior cardinal veins join and become the right and left common cardinal veins. These drain into a common channel, the sinus venosus, which returns blood to the primitive heart. The distal portion of the left anterior cardinal vein regresses. The left common cardinal vein becomes the coronary sinus. As the system matures, the SVC forms at the confluence of the right and left brachiocephalic veins.
Successive venous networks form during embryonic development of the caudal circulation. The paired posterior cardinal veins form an iliac anastomosis in what will become the pelvis. The caudal portions of the veins form the common, external, and internal iliac veins, as well as the medial sacral vein. The cranial portion of the right posterior cardinal vein becomes the arch of the azygous. 4 The subcardinal veins form during the fourth week of gestation and coincide with the development of the mesonephros. The subcardinal venous network arises from the anastomosis of the internal veins of the Wolffian body and the posterior cardinal veins and replaces the posterior cardinal system. The subcardinal sinus becomes the left renal vein. The right subcardinal vein forms the right gonadal vein and portions of the pararenal vena cava. By the seventh week, the supracardinal system develops. The supracardinal system is located dorsally and runs parallel to the sympathetic chain. The bilateral supracardinal veins form anastomoses with one another and with the subcardinal veins on the right. The right supracardinal vein becomes the azygous vein and the left supracardinal becomes the hemiazygous vein. 6
The inferior vena cava (IVC) forms in segments arising from different embryologic networks. The hepatic segment of the IVC is formed by hepatic sinusoids. The right subcardinal vein forms the prerenal and pararenal IVC, accounting for the relatively anterior positioning of the IVC at this level. 4 The renal segment of the IVC forms from the subcardinal and supracardinal anastomosis. The caudal postrenal segment of the IVC originates from the right supracardinal vein. Due to the posterior development of supracardinal network, the distal IVC and common iliac veins are posterior to the distal aorta and common iliac arteries. 4 , 7
Vascular development of the limbs begins with a capillary network arising from arterial structures. As blood drains through the capillary network, blood collects in a marginal sinus within the limb bud. Early on, blood drains through a small superficial network back to the heart. As the limb develops, deep venous structures begin to form parallel to major arteries, and blood is shunted into the deeper veins. 6
Development of the portal venous system occurs separately through the vitelline or omphalomesenteric system. The vitelline vein transports blood from the yolk sac to the heart. During development, a vascular network from the vitelline vein forms around the primitive duodenum. As the liver primordium proliferates, the vitelline vein fragments and forms the hepatic sinusoids. Cranial to the liver, the vitelline vein drains into the sinus venosus. As the left side of the sinus regresses, the right vitelline vein forms an anastomosis and becomes the suprahepatic segment of the IVC. Caudal to the liver, the anastomotic network surrounding the duodenum becomes a single trunk and eventually develops into the main portal vein. Portions of the remaining right vitelline vein become the superior mesenteric vein, and the distal left vitelline vein becomes the splenic vein. The proximal right and left vitelline veins become the right and left hepatic veins. 8 , 9
The umbilical veins transport oxygenated blood from the placenta. The left umbilical vein persists during development and communicates with the IVC via the ductus venosus. By 12 weeks of gestation, the ductus venosus is developed and carries oxygenated placental blood directly to the heart and bypasses the hepatic circulation. Following birth, the ductus venosus atrophies and closes to form the ligamentum teres. 6 , 8
1.3 Anatomy and Variants
1.3.1 Upper Extremity and Thoracic Veins
Veins of the upper extremities develop in both superficial and deep networks. Perforating veins connect the superficial and deep veins. 10 Cardiac function plays a major role in venous return from the upper extremities. Unlike in the lower extremities, the upper extremities do not rely heavily on valves for effective venous return. 4 The major deep veins include the radial, ulnar, brachial, and axillary veins. Deep veins course with the major arteries of the same name. The cephalic and basilic veins are the main superficial conduits. The median cubital vein connects the cephalic and basilic veins. The cephalic vein receives tributaries from the dorsal venous plexus on the radial side of the hand and continues on the ventral radial forearm. The cephalic vein courses over the lateral arm, traverses the clavipectoral fascia, and drains into the axillary vein. The basilic vein receives tributaries from the ulnar side of the dorsal venous plexus, courses up the ulnar forearm, and enters the deep fascia in the mid arm before continuing as the axillary vein. 10
The axillary vein begins anatomically at the inferior border of the teres major and drains centrally into the subclavian vein, which begins at the border of the first rib. 4 , 10 The subclavian vein and internal jugular vein join to form the brachiocephalic vein at the medial border of the anterior scalene muscle. The left brachiocephalic vein traverses the chest to join the right brachiocephalic vein at the SVC. The axillary vein receives many tributaries from the chest and neck, and these serve as the basis for collateral pathways in cases of obstruction. The pectoral branch of the thoracoacromial trunk, the lateral thoracic vein, and thoracodorsal vein all drain into the axillary vein. Posterior intercostal veins freely communicate with the anterior intercostal veins and the lateral and internal thoracic veins. The vertebral, internal thoracic, inferior thyroid, and left superior intercostal vein all drain into the brachiocephalic vein. 4 The internal jugular vein receives tributaries from the superior and middle thyroid veins. The external jugular vein communicates with the internal jugular and receives drainage from the transverse cervical, suprascapular, and anterior jugular veins. 11
A duplicated SVC is the most common SVC variant, occurring in approximately 0.3% of the general population. 12 Incidence is higher in individuals with congenital heart disease. 12 Duplication results from persistence of the caudal portion of the left anterior cardinal vein. An isolated left-sided SVC occurs when the caudal portion of the left anterior cardinal vein persists and the caudal portion of the right anterior cardinal vein regresses. The brachiocephalic vein still forms, connecting the right anterior cardinal vein to the left anterior cardinal vein. The left-sided SVC maintains drainage into the right atrium through the coronary sinus (Fig. 1.1). 4
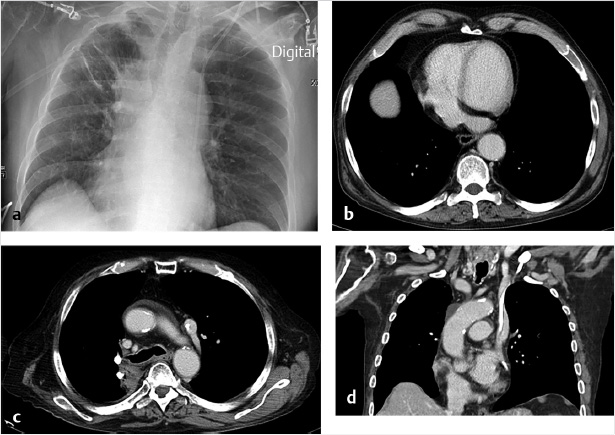
1.3.2 Abdominopelvic Veins
The IVC is the major venous conduit of the abdomen and courses along the right side of the vertebral column before terminating in the right atrium. Major abdominal tributaries to the IVC include the hepatic veins, bilateral renal veins, and the lumbar veins. The IVC also receives venous blood return from the pelvis. The inguinal ligament is the inferior anatomic landmark at which pelvic veins begin. The external iliac vein courses through the pelvis, receiving drainage from the inferior epigastric, deep circumflex iliac, and pubic veins. The internal iliac veins receive extrapelvic tributaries including the superior and inferior gluteal, internal pudendal, and obturator veins. 4 Intrapelvic drainage to the internal iliac vein comes from multiple venous plexuses within the pelvis. The presacral plexus, visceral plexus, and pudendal plexus provide free flow between both sides of the pelvis. Major tributaries include rectal, vesicular, uterine, prostatic, and vaginal veins. The external and internal iliac veins join in the region of the sacroiliac joint to form the bilateral common iliac veins, which then join to form the IVC. 4 , 13
Duplication of the IVC occurs in about 2 to 3% of the population and results when the left supracardinal vein fails to regress (Fig. 1.2). An isolated left-sided IVC occurs in less than 0.5% of the population and results from persistence of the left supracardinal vein and regression of the right supracardinal vein. 3 , 7 The IVC ascends to the left of the aorta and crosses over to the right at the level of the renal arteries. The right adrenal and gonadal veins empty into the right renal vein, while the left adrenal and gonadal veins drain directly into the IVC. Renal vein anomalies also occur and can be clinically significant. A posterior or retroaortic left renal vein occurs in approximately 1 to 2% of the population (Fig. 1.3). A circumaortic renal vein is more common, occurring in 1 to 9% of the population. 3 , 4 , 14
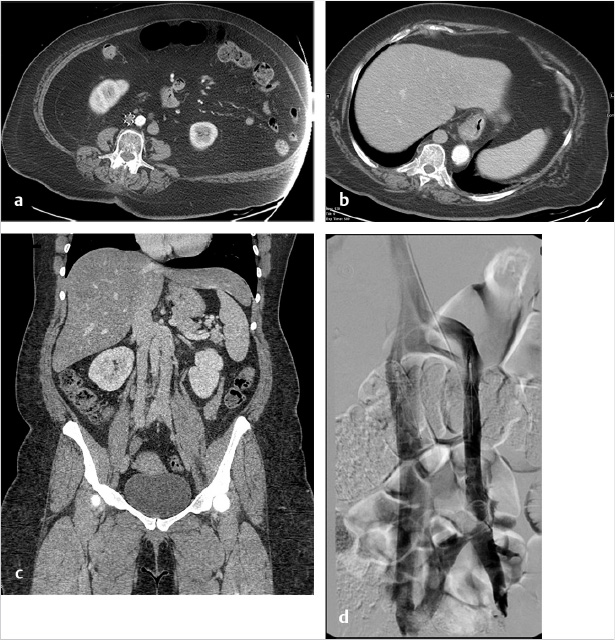
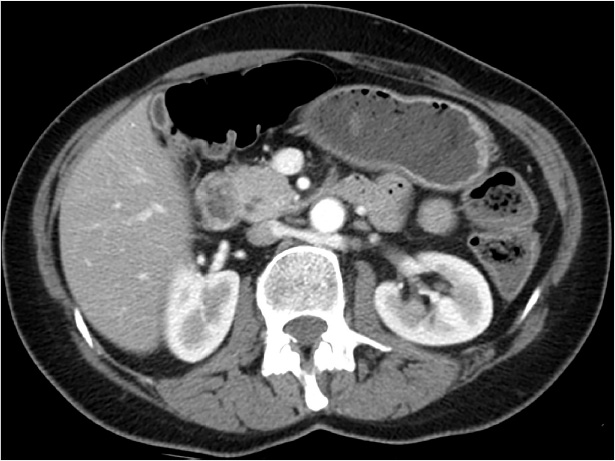
1.3.3 Azygous System
The azygous, hemiazygous, and accessory hemiazygous veins form a paired paravertebral venous pathway. The azygous system is an important collateral pathway between the SVC and IVC, and can serve as a shunt in cases of obstruction. The azygous vein is variable in origin, but usually begins at the junction of the right ascending lumbar and subcostal veins. The azygous vein ascends posteriorly on the right side to the level of T4–T6, where it passes anterior to the right mainstem bronchus and joins the SVC. The hemiazygous vein originates at the L1–L2 level and ascends on the left side of the vertebral column. The hemiazygous vein crosses midline at approximately T8 and passes posterior to the aorta and esophagus, draining into the azygous vein. The accessory hemiazygous vein originates from the left brachiocephalic vein and descends on the left side of the vertebral column, draining into the azygous or hemiazygous at approximately T7. The superior intercostal vein, bilateral posterior intercostal veins, bronchial veins, and the vertebral venous plexus also drain directly into the azygous system. 12 , 15
The azygous system can be variable and congenital variants are rarely symptomatic. Azygous continuation of the IVC (Fig. 1.4) occurs in roughly 0.6% of the population. 14 , 15 The hepatic segment of the IVC is absent and the hepatic veins drain directly into the right atrium. 15 Azygous continuation is commonly associated with duplication of the IVC. Typically, the left IVC will terminate at the left renal vein and the right IVC will remain as the azygous vein, bypass the liver, and join the SVC. 15 Separate right and left azygous veins occur in 1 to 2% of individuals. A single midline azygous vein also occurs in approximately 1 to 2%. 12 Incomplete medial migration of the right posterior cardinal vein results in an azygous lobe of the right lung in 0.5 to 1% of the population (Fig. 1.5). 15
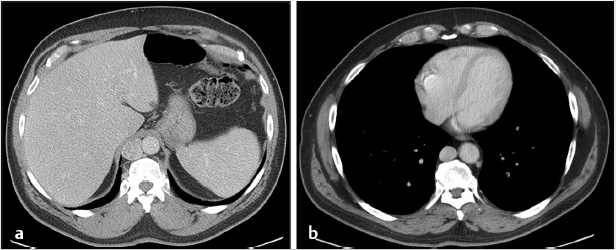
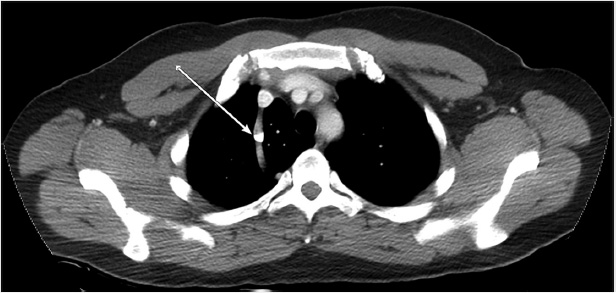
1.3.4 Lower Extremity Veins
Lower extremity veins, like upper extremity veins, are divided into superficial and deep networks. Perforating veins connect the superficial and deep systems. Intraluminal valves play an essential role in lower extremity venous return, helping to maintain unidirectional flow and regulate venous pressure as blood is returned to the heart. 13
Superficial veins are located outside of the deep muscular fascia. The superficial veins of the lower extremity drain blood from the skin and subcutaneous tissues of the lower extremity. Superficial venous anatomy is highly variable. The dorsal and plantar venous networks comprise the superficial veins of the foot. The dorsal venous arch overlies the proximal metatarsal heads. The medial end of the arch becomes the medial marginal vein, which drains into the great saphenous vein. The lateral aspect becomes the lateral marginal vein, which drains into the small saphenous vein (SSV). 4 , 13
The main conduit in the superficial system is the great saphenous vein (GSV), which is contained within the saphenous subcompartment and covered by a fascial layer. The saphenous fascia separates the GSV from the remainder of the superficial compartment. 4 The GSV ascends the medial aspect of the lower extremity, beginning anterior to the ankle and terminating at the fossa ovalis. Duplication of the GSV is a relatively common anatomic. Duplication below the knee is seen in approximately 25% of the population and duplication above the knee occurs in approximately 8%. 4 The SSV begins in the distal calf and ascends the calf laterally in the subcutaneous tissue. The SSV enters the deep fascia in the upper calf between the medial and lateral heads of the gastrocnemius muscle. The SSV enters the popliteal fossa and drains into the popliteal vein. Often, a small cranial extension of the SSV ascends into the thigh. 3 , 4
Deep veins are located within the fascial compartments and run parallel to the major arteries. The deep plantar venous arch receives drainage from the foot, becoming the plantar veins and eventually forming the paired posterior tibial veins. 4 , 13 The posterior tibial veins drain the deep and superficial posterior compartments in the calf and are located between the flexor digitorum longus and the tibialis posterior. Dorsally, the anterior tibial veins receive drainage from the dorsalis pedis veins. The anterior tibial veins drain the anterior compartment. The peroneal vein courses deep to the flexor hallucis longus muscle and communicates with both the anterior tibial and posterior tibial veins. The posterior tibial and peroneal veins form the tibioperoneal trunk. The anterior tibial vein and tibioperoneal trunk join to form the popliteal vein. The popliteal vein becomes the femoral vein in the adductor canal. The femoral vein and the profunda femoral veins join to become the common femoral vein. The common femoral vein courses medial to the artery and at the level of the inguinal ligament and becomes the external iliac vein. 4
Perforating veins are the connection between the superficial and deep veins of the lower extremity. Like other lower extremity veins, perforating veins also contain valves, which ensure one-way flow from the superficial to the deep venous system. 13 While highly variable in location, there are several regions where perforating veins are predictably found. In the foot, a perforating vein is usually located between the first and second metatarsals connecting the dorsal venous arch to the pedal vein. Multiple perforating veins are present in the ankle, both medially and laterally. The medial calf perforating veins are the most widely studied, and can be divided into the posterior tibial and paratibial perforating veins. 4 The posterior tibial perforators are divided into lower, middle, and upper sections. The posterior tibial perforators connect the posterior accessory GSV to the posterior tibial veins, while the paratibial perforators drain the GSV. 4 Numerous other perforating veins occur superior to the knee, draining the superficial veins into the femoral vein.
1.3.5 Portal and Hepatic Venous System
The portal vein forms at the confluence of the splenic and superior mesenteric veins, posterior to the head of the pancreas and anterior to the IVC. The portal system receives blood from the mesenteric, splenic, gastroduodenal, pancreatic, and cystic veins. About 75 to 80% of the hepatic blood supply comes from the inflow from the portal system. 8 The main portal vein runs in the hepatoduodenal ligament with the common bile duct on the right and the hepatic artery on the left. The right portal vein divides into anterior and posterior segments in the right hepatic lobe. The left portal vein has a transverse segment supplying the caudate lobe and an umbilical segment that divides into superior and inferior branches. The hepatic veins and portal veins are major landmarks in the Couinaud functional segmental anatomy of the liver. The portal vein creates a horizontal plane, which divides the liver into superior and inferior portions. The major hepatic veins create vertical planes separating segments. The right hepatic vein divides the right lobe into anterior and posterior sections. The middle hepatic vein lies in the main lobar fissure and divides the liver into the right and left hepatic lobes. The left hepatic vein divides the left lobe into medial and lateral sections. The three vertical planes and one horizontal plane divide the liver into eight segments. The hepatic veins function to provide outflow from the liver into the systemic circulation. Intralobular veins coalesce from the hepatic sinusoids, eventually draining into the hepatic vein branches. The right hepatic vein drains the posterior and superior liver segments and commonly joins the IVC separately. The middle hepatic vein drains portions of the right hepatic lobe and medial portions of the left hepatic lobe. The left hepatic vein drains both lateral left hepatic lobe segments and the superior medial segment. The middle and left hepatic veins often form a common trunk before entering the IVC. 8
1.4 Physiology
The venous system functions to return blood to the heart as well as provide cardiovascular homeostasis through changes in venous tone. 1 Venous return, the rate of blood flow toward the heart, is a complex process requiring a central pump, pressure gradients, peripheral muscle pumps, and one-way valves. 1 , 3 Veins, unlike arteries, are high-capacitance vessels able to accommodate highly variable blood volumes without significant changes in venous pressure. 3 The heart serves as the central pump, moving blood through both arteries and veins and creating dynamic pressure. Normal right atrial pressure ranges from 4 to 7 mm Hg and capillary bed pressures are approximately 12 to 18 mm Hg, and blood is returned to the heart along this gradient. 1 , 2
Venous flow is highly dependent on hydrostatic pressure in the upright position. Hydrostatic pressure is the weight of the blood column below the right atrium, as determined by blood density and the acceleration of gravity. The effect of gravity increases by 0.77 mm Hg per cm of height below the right atrium and the effects of hydrostatic pressure must be overcome in order for blood to return from the dependent portions of the body. 1 , 2 , 3 The skeletal muscle pump and venous valves function to counteract hydrostatic pressure in the lower extremities. The calf muscle pump is the most widely studied and produces the most physiologic impact. Contraction of the gastrocnemius and soleus muscles forcefully advances blood back toward the heart, generating pressures of over 200 mm Hg with each contraction. This pressure is enough to eject 60% of the usual 100 to 150 mL blood contained within the veins of the calf. 1 , 3 Similar physiology is thought to occur in the plantar venous plexus, as well as the thigh; however, the effects on venous return are thought to be minimal in comparison to the calf.
Along with the muscle pump, valves play a critical role in lower extremity venous return. Deep veins in the lower extremity contain numerous valves, at increasingly frequent intervals moving proximal to distal. Deep veins of the calf have valves occurring in approximately 2-cm intervals. 4 A valve is almost always found at the junction of the femoral vein and profunda femoral vein. The proximal common femoral vein usually contains only one valve, and the common iliac vein and IVC contain no valves. Likewise, upper extremity veins contain fewer valves because the effects of gravity are significantly less. 4 The calf muscles also contain venous sinuses, thin-walled chambers able to hold a significant blood volume. Venous sinuses are frequently found within the soleus muscle, and contract with ambulation. Sinuses fill from superficial veins and subsequently drain into the deep posterior tibial veins. The sinuses are valveless, but the draining veins connecting the deep veins contain numerous valves, preventing backflow. 1 , 4
Respiration also affects venous return by movement of the diaphragm and changes in intra-abdominal pressure. For blood to drain from the peripheral veins through the abdominal cavity, pressure in the IVC must exceed that of the abdominal cavity. Increase in intra-abdominal pressure, caused by descent of the diaphragm during inspiration, will cause a decrease in the pressure gradient and therefore slow or stop venous return. For blood return from the upper extremities, low intrathoracic pressure creates a pressure gradient favorable for venous return. Therefore, respiration has less effect on upper extremity venous return. In cases of congestive heart failure or pulmonary hypertension, central pressure is elevated and flow pattern depends on right heart function. 2
In addition to blood return, veins play an important role in hemodynamic stability. Veins have both high capacitance and compliance, allowing the venous system to accommodate as much as a 20 to 30% increase in volume if needed. 1 Changes in vasomotor tone, distention, and recoil of the vein wall allow for these changes in volume. The venous wall is collapsible and varies in shape depending on volume and pressure. When volume is low, the vein walls are coapted and have an ellipsoid shape. Relatively large volume shifts can occur with little pressure change. As volume increases, veins distend to a circular shape, at which point pressure begins to increase more significantly per unit volume. The transmural pressure is the difference between intraluminal pressure distending the vein and the external pressures collapsing the vein. An increase in transmural pressure from 0 to 15 mm Hg corresponds to a 250% increase in volume. 2
Smooth muscle activity in the wall of both arteries and veins is mediated by the sympathetic nervous system. Venous innervation is greatest in the splanchnic and cutaneous venous system, where smooth muscle is most prevalent. The splanchnic circulation usually accounts for approximately 25% of the total blood volume. 1 Stimulation of the sympathetic nervous system results in acute changes in splanchnic blood volume. Arterial receptors such as the carotid sinus can also cause changes in venous capacitance. For example, when blood pressure is low, catecholamine release causes increased venous smooth muscle tone and increase in venous return to the heart. 1 , 2
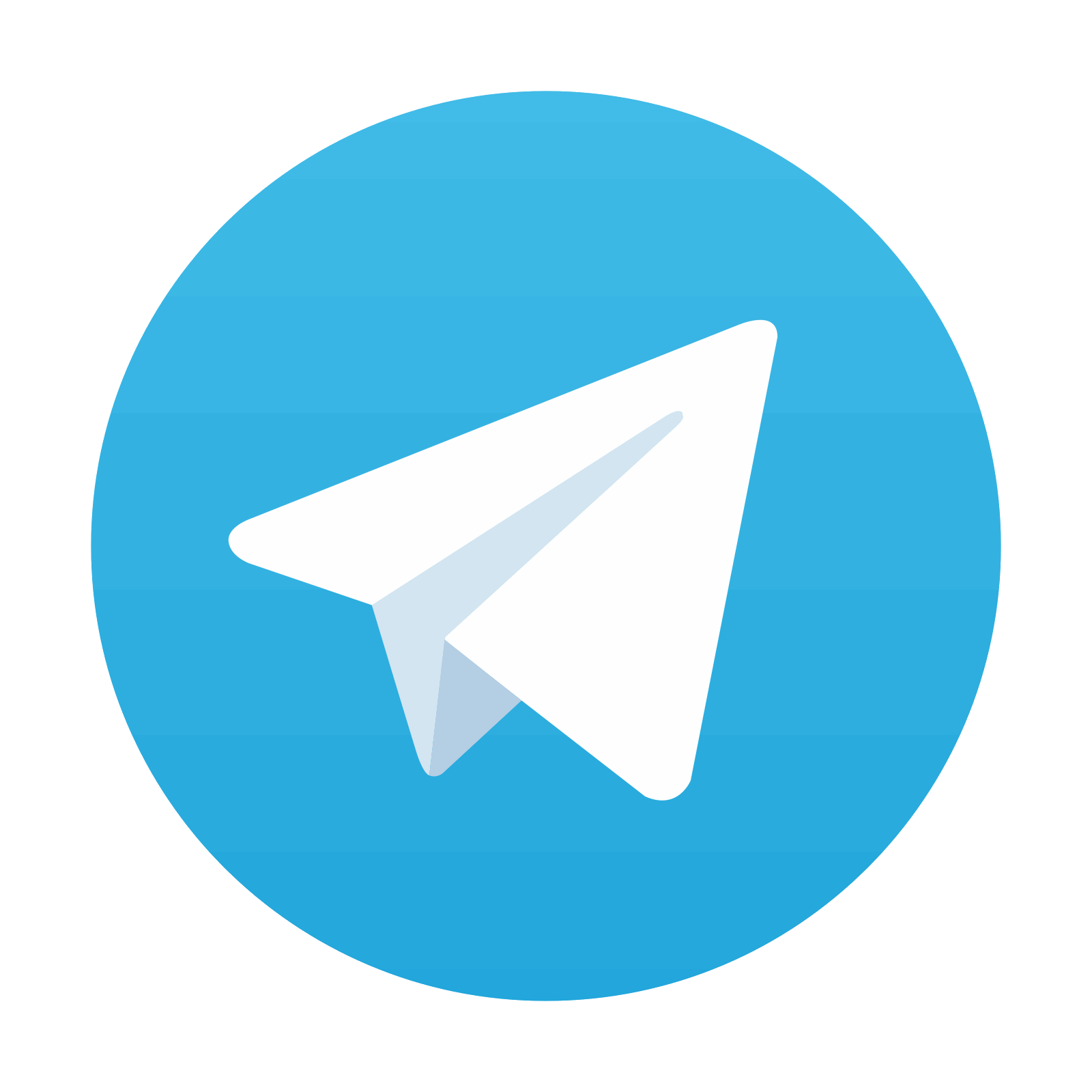
Stay updated, free articles. Join our Telegram channel

Full access? Get Clinical Tree
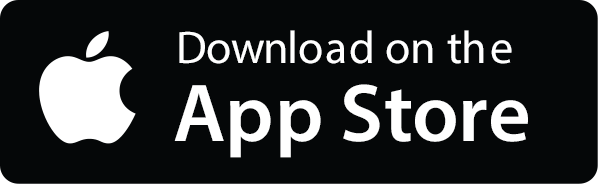
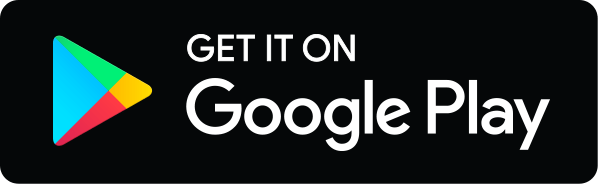