2 Venous Thromboembolism
Summary
Venous thromboembolism has been increasingly recognized as a significant contributor to patient morbidity and mortality, and there has been rapid growth in the application of interventional techniques to the management of venous thrombotic disorders. For the physician managing a patient with a venous thrombosis, an excellent understanding of the mechanisms of disease, thrombosis physiology, pathophysiology of clotting disorders, data to support the application of different pharmacologic interventions, and risks and benefits of different therapies is critical to success. This chapter provides a thorough overview of the science and pharmacologic management of thrombosis, as well as details of patient evaluation, epidemiology, and expected outcomes of intervention.
2.1 Introduction
Venous thromboembolism (VTE) is a major public health burden globally and, after myocardial infarction and stroke, the third most common cardiovascular disease. The causes of VTE are complex and multifactorial and include both inherited and acquired factors. As our population ages and develops VTE risk factors, the incidence and prevalence of VTE will continue to increase—as likely will its attendant complications, including postthrombotic syndrome (PTS) and pulmonary hypertension. Thus, for providers in all settings, an understanding of VTE is warranted.
Starting with a clinical vignette, this chapter will review the pathophysiology and epidemiology of VTE, including common provoking risk factors and inherited thrombophilias. The appropriate use of algorithms to diagnosis deep vein thrombosis (DVT) and pulmonary embolism (PE) will be reviewed, including a discussion on potentially more complex and challenging diagnostic issues—such as recurrent ipsilateral DVT and when computed tomography pulmonary angiography (CTPA) is contraindicated in patients suspected of having acute PE. With the advent of the direct oral anticoagulants (DOACs), therapeutic options for the treatment of VTE have broadened. Accordingly, we will discuss central issues in the management of VTE, including acute antithrombotic therapy, thrombolytics for massive PE, and the duration of anticoagulation for provoked and unprovoked events.
2.2 Clinical Vignette
2.2.1 Patient Presentation
A 46-year-old white female presented to clinic with a 2-week history of progressive exertional dyspnea that had worsened over the past 2 days. She also had the onset of right pleuritic chest pain 1 day earlier and two episodes of presyncope with palpitations and chills. She denied fevers, cough, or wheezing. She had tried an albuterol inhaler prescribed by a friend without benefit. She was a lifetime nonsmoker. Her past medical history was notable for gastroesophageal reflux disease, irritable bowel syndrome, and menorrhagia. Her past surgical history included a remote left oophorectomy for an ovarian cyst. Her current medications included lansoprazole, oral contraceptive pills (taken for the past 2 years), and dicyclomine.
2.2.2 Physical Exam
She was a pleasant obese white female in no acute respiratory distress, although she appeared mildly anxious. Her body mass index was 42 kg/m2, blood pressure was 116/76 mm Hg, pulse was 91 beats per minute, respiratory rate was 16 breaths per minute, the temperature was 98.6°F, and her room air oxygen saturation was 96%. Her cardiovascular exam noted a jugular venous pulsation of 5 cm H2O, and there was a regular heart rate and rhythm without murmurs or gallops. Her lungs were clear to auscultation and her abdomen was soft, nontender, with normal bowel sounds and no masses or organomegaly. Her extremities were without edema, there was no calf tenderness to palpation, and distal pulses were intact.
2.2.3 Lab Evaluation
Complete blood count, prothrombin time and international normalized ratio (INR), partial thromboplastin time, and comprehensive metabolic panel were within normal range. The troponin-I was 0.03 ng/mL (normal: 0.0–0.03 ng/mL) and the D-dimer was elevated at 2.5 μg/mL (normal 0.0–0.04 μg/mL).
2.2.4 Noninvasive Testing
An electrocardiogram demonstrated normal sinus rhythm with a rate of 90 beats per minute and diffusely inverted T waves across precordial leads.
2.2.5 Imaging
A posteroanterior and lateral chest X-ray was normal. CTPA demonstrated bilateral filling defects involving both the right and left main pulmonary arteries and extending into the segmental and subsegmental arteries, with distention of the right ventricle suggestive of right heart strain. A transthoracic echocardiogram demonstrated normal left ventricular systolic function with an ejection fraction of 68%, normal right ventricular systolic function, and mildly elevated pulmonary artery systolic pressure.
2.2.6 Plan of Care
She was admitted to the hospital with a diagnosis of acute, submassive PE and was treated with antithrombotic therapy.
2.3 Pathophysiology and Epidemiology of Venous Thromboembolism
Venous thrombi are classically comprised largely of red blood cells, with some leukocytes and platelets, interwoven in a fibrin lattice (Fig. 2.1a). The formation of venous thrombosis occurs commonly in settings where there is vessel wall injury or stasis (e.g., surgery, trauma, malignancy), systemic inflammation (e.g., lupus, sepsis), and/or a procoagulant state (e.g., inherited thrombophilia, antiphospholipid antibodies). Vessel wall injury exposes tissue factor and collagen, leading to activation of the coagulation cascade. Historically, the coagulation cascade has been divided into the intrinsic and extrinsic pathways (Fig. 2.1b). More recently, a cell-based model of coagulation has been put forth, whereby activation of the coagulation cascade occurs on the cell surface in four overlapping steps: (1) initiation, (2) amplification, (3) propagation, and (4) termination. The primary event for initiation of this cascade is the interaction of activated factor VII (FVIIa) with exposed tissue factor, converting factor II (FII, also known as prothrombin) to thrombin (FIIa) and fibrinogen to fibrin.
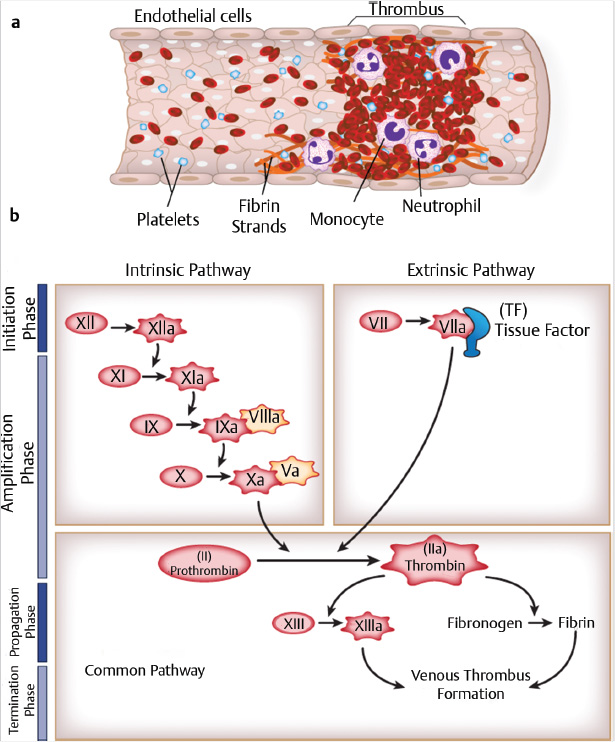
The epidemiology of VTE—including PE and DVT of the leg, pelvis, and arm—has recently been reviewed. 1 , 2 VTE carries a major global disease burden with respect to incidence, morbidity, mortality, and cost. 3 , 4 After myocardial infarction and stroke, VTE is the third most common cardiovascular disease, with a lifetime risk after age 45 years of 8.1%. 5 The age-adjusted annual incidence of VTE has increased by 80% from 1985 to 2009 to 1.7/1,000/year. While DVT incidence has remained stable, PE incidence has progressively increased, possibly due to a combination of an aging population, improved diagnostic techniques, and/or poorly defined increases in VTE risk factors. 6
VTE results in some 550,000 hospitalizations per year in the United States—350,000 for DVT and 200,000 for PE 7 —with at least 44,000 deaths per year. Moreover, this mortality rate is likely an underestimate as 10 to 25% of PE patients in the community may present with sudden death prior to diagnosis. 8 Other consequences of VTE include a VTE recurrence rate of 15% over 3 years and 30% over 5 to 10 years in the absence of anticoagulation 2 , 9 and a 6% rate of major bleeding over 3 years. 10 In addition, PTS (Fig. 2.2) occurs in 20 to 50% of patients within 2 years of a first episode of proximal DVT. 11 Severe PTS symptoms and/or venous ulceration develop in 5 to 10% of patients. 12 There is also a 2.8% incidence of chronic thromboembolic pulmonary hypertension within 1.5 years of an episode of PE. 13 The result is an estimated annual cost of VTE in the United States of $7 to 10 billion per year. 3
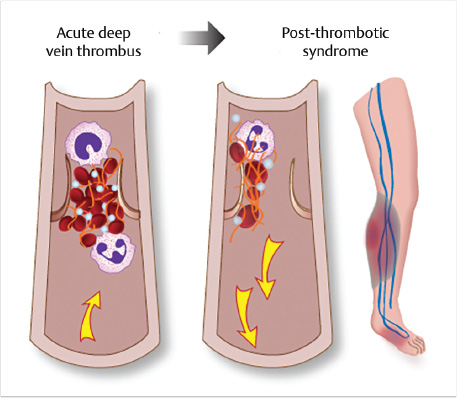
2.3.1 Risk Factors for Venous Thromboembolism
VTE results from the complex, multiplicative interaction of inherited and/or acquired predisposing risk factors, often superimposed by the appearance of temporary clinical risk factors (Table 2.1). 2 , 14 , 15 Recent cohort studies suggest that about 35% of first-episode VTE events are provoked by an identified temporary clinical risk factor, 20% are cancer-associated, and up to 45% are unprovoked (idiopathic). 10 , 16 The most common of the temporary clinical risk factors include surgical hospitalization within 3 months (24%), medical hospitalization within 3 months (22%), nursing home residence (14%), and central vein cannulation of the upper extremity (10%). 2 , 17 The incidence of VTE is strongly age-related, increasing three- to fivefold from patients aged 50 to 60 years to patients aged 70 to 80 years. 18 In adults aged 70 years and older, the annual VTE incidence is nearly 1%. 8 , 16 , 19 The incidence of VTE is also higher in blacks than whites, with Asian Americans and Native Americans at lowest risk, perhaps due in part to a variable prevalence of genetic thrombophilia. 20 Major (e.g., deficiencies of proteins C and S and antithrombin III) and minor (e.g., heterozygous factor V Leiden and prothrombin gene mutations) genetic thrombophilia increases the risk of a first VTE episode but minimally increase the risk of recurrent VTE. 21 For this reason, thrombophilia testing in VTE patients or their asymptomatic relatives rarely alters management decisions, may result in harm because of inaccurate interpretation and decision-making, and should be performed only in highly selected situations. 21 Testing for acquired antiphospholipid antibody syndrome may be useful in some patients. Independent risk factors for a first episode of VTE among cancer patients include: cancer site (especially brain, pancreas, other gastrointestinal organs, lymphoma, and leukemia), advanced cancer stage (especially with liver metastases), chemotherapy, central vein cannulation, and hospitalization or nursing home placement. 22
Independent risk factors for recurrent VTE include active cancer, unprovoked VTE, multiple VTE recurrences, male sex, increasing obesity, advancing age, antiphospholipid antibody syndrome, and persistently increased plasma D-dimer following cessation of anticoagulation. 2 PTS, even when severe, does not appear to be a risk factor for recurrent VTE. 23
2.4 The Diagnosis of Venous Thromboembolism
The diagnosis of lower extremity DVT and PE is currently based on validated, Bayesian algorithms derived from outcome-management studies. 24 , 25 , 26 These studies prospectively enrolled consecutive patients with suspected VTE. All patients progressed through a particular diagnostic algorithm to confirm or exclude VTE with at least a 3-month follow-up when VTE was excluded. The pretest probability (PTP) of VTE was assessed using validated clinical decision rules to stratify VTE risk as low, moderate (intermediate), or high, or alternatively as unlikely or likely. Patients with low, moderate, or unlikely PTP were then tested for high-sensitivity D-dimer (HS-DD). A normal HS-DD in these patients safely excluded VTE without the need for diagnostic imaging in 20 to 30% of patients, with the likelihood of a new VTE diagnosis over the next 3 months being less than 2% (upper 95% confidence boundary less than 3%). Nevertheless, as discussed below, this is not a safe approach in cancer patients with suspected DVT. 24 , 27 , 28 , 29 A normal HS-DD was also not sufficient to safely exclude VTE in patients with high or likely PTP and should not be routinely ordered. These patients and those with elevated HS-DD generally should proceed to diagnostic imaging with compression ultrasound (CUS) for suspected DVT or to CTPA, or alternative pulmonary imaging, for suspected PE. After a negative imaging study in these patients, the 3-month VTE rate is generally less than 2% for both suspected DVT and PE (Fig. 2.1). 24 , 27 , 28 , 29 Unfortunately, while these algorithms provide accurate and safe diagnosis of VTE, their actual utilization in clinical practice is suboptimal. 29 Omission or miscalculation of PTP assessment and/or omission of HS-DD testing has led to unnecessary imaging in many patients with consequent expense, radiation exposure, contrast nephropathy, contrast allergy, falsely positive or inconsequential incidental findings, and missed diagnoses with adverse patient outcomes. An algorithm for providers, based on these and other studies, is shown in Fig. 2.3.
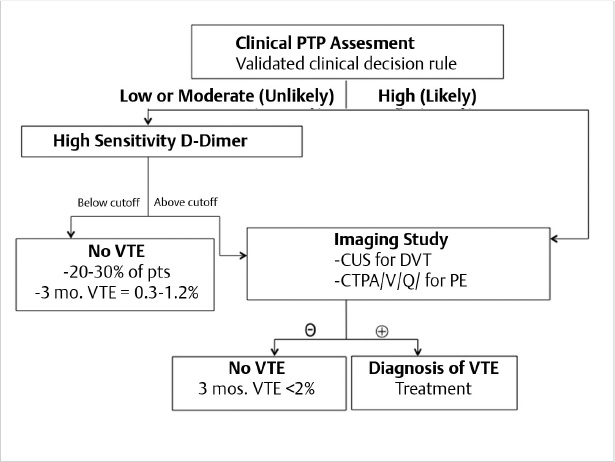
2.4.1 The Diagnosis of Deep Vein Thrombosis
A large, individual patient meta-analysis found that the combination of a low/moderate/unlikely 10-point Wells score (Table 2.2) and a normal HS-DD safely rules out DVT (3-month VTE recurrence rate < 2%) in about one-third of outpatients with suspected first-episode DVT. 24 However, the Wells score combined with HS-DD approach does not appear to be sufficiently safe or efficient to be used in cancer patients, 24 hospitalized patients, 30 or possibly in patients with suspected recurrent ipsilateral DVT. These patients should proceed directly to CUS evaluation. The use of an age-adjusted HS-DD cut-off (age × 10 for patients age > 50 years) rather than ≥500 μg/L has not yet been sufficiently validated for use in clinical practice for patients with suspected DVT. 24
CUS for DVT diagnosis may be applied only to proximal leg veins (termed limited CUS) or instead to both proximal and distal leg veins below the popliteal vein (termed whole-leg CUS). Direct comparisons of the two approaches demonstrate equivalent diagnostic accuracy. 31 Consistent with this, current guidelines 26 , 32 indicate that either approach may be used (Fig. 2.4). However, a normal limited CUS study requires a repeat CUS study in 1 week in patients with moderate PTP and an elevated HS-DD and in patients with high or likely PTP, in order to detect progression of isolated distal DVT (IDDVT) into the popliteal vein. This progression occurs in 8 to 15% of patients and primarily in the first week. 33 While a single, whole-leg CUS by a skilled operator safely rules out DVT, 34 , 35 40 to 50% of DVT events detected by whole-leg CUS are IDDVT. The clinical significance of IDDVT events and their need for anticoagulation remain controversial. 32 , 33 Recently, the prospective, multicenter PALLADIO study with 1,162 outpatients validated the safety and convenience of an algorithm combining limited CUS and whole-leg CUS to diagnose DVT. This approach eliminated the need for repeat CUS but also reduced the detection of clinically insignificant IDDVT. 36 With this diagnostic strategy (Fig. 2.4), the 3-month VTE incidence in untreated patients was 0.87% (95% confidence interval [CI]: 0.44–1.70).
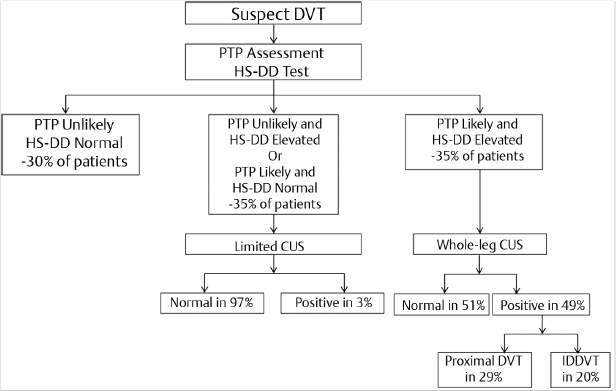
2.4.2 Diagnosis of Upper Extremity DVT
Upper extremity DVT (UEDVT) includes thrombosis in the brachial, axillary, subclavian, internal jugular, and brachiocephalic veins; the cephalic and basilic veins are considered distal, superficial veins. 37 UEDVT now constitutes about 10% of all DVT but up to 50% of DVT in hospitalized patients. 38 , 39 , 40 About 30% of cases of UEDVT may be classified as primary—that is, idiopathic or due to excessive upper extremity exercise or thoracic outlet vein compression. 41 Secondary UEDVT is associated with placement of central venous catheters or pacemakers and/or with cancer. 41 In some settings, central venous catheters may also increase the risk of lower extremity DVT, independent of causing UEDVT. 17 Complications from UEDVT are less frequent than with lower extremity DVT but PE (3–12%), PTS (7–46%), and recurrent VTE (2–9%) may still occur. 41 , 42 A high-quality, outcome-management study of 483 patients with suspected UEDVT has recently confirmed the safety of a normal single whole-arm CUS to exclude UEDVT. 43 In this study, the 3-month VTE rate was only 0.3% (95% CI: 0.05–1.68). 43 Another outcome-management study has found that an algorithm combining the Constans Clinical Decision Score, HS-DD, and whole-arm CUS safely ruled out UEDVT and did not require CUS imaging in 21% of patients, but further studies are necessary to confirm its safety. 44 , 45 , 46
2.4.3 Diagnosis of Recurrent Ipsilateral DVT
The accurate diagnosis of recurrent ipsilateral DVT may be challenging. 29 , 47 , 48 For over 10 years, up to 40% of patients with prior DVT present with signs and/or symptoms of possible recurrence. About one-half of these patients will have DVT recurrence confirmed, about one-third will have an inconclusive evaluation, and the rest will have an acute exacerbation of PTS or other diagnoses. 29 , 48 Missing the diagnosis of recurrent DVT exposes the patient to the complications of untreated VTE. Nevertheless, conversely, making a falsely positive diagnosis may commit the patient to indefinite or life-long anticoagulation with its attendant risks of bleeding and other adverse effects. Unfortunately, a validated, safe algorithm incorporating PTP assessment, HS-DD, and CUS is not yet available. While the 10-point Wells score includes one point for prior VTE, its reliability to safely exclude VTE when combined with a normal HS-DD has not been validated in a large outcome study. 24 The efficiency of this approach is also reduced as HS-DD may remain elevated in nearly 50% of VTE patients 3 months or longer after cessation of anticoagulation. Recommendations vary as to whether an unlikely PTP and normal HS-DD can 47 or cannot 29 , 48 be used to exclude recurrent ipsilateral DVT.
CUS results may be often inconclusive in these situations. A normal whole-leg CUS does rule out recurrent DVT. However, 1 year after a first DVT event, 40 to 50% of patients have persistent noncompressibility that cannot be definitively distinguished from acute thrombus. If a prior CUS study is available for comparison, new, noncompressible venous segments confirm the diagnosis of recurrent DVT. In settings where there may be residual vein thrombosis, whether a greater than 2- to 4-mm increase in the residual vein diameter of affected proximal leg veins confirms recurrent DVT, or its absence rules out recurrent DVT, is controversial. If the current CUS is nondiagnostic in comparison to a prior CUS, or if a prior CUS is unavailable, careful clinical judgment and consultation with a VTE specialist, where available, is recommended. Fig. 2.5 provides potential options for diagnosing recurrent ipsilateral DVT as suggested by several investigators. 29 , 47 , 48 , 49 A promising new diagnostic approach not yet validated by a large outcome-management study is magnetic resonance direct thrombus imaging. In a small study of 81 patients, this procedure differentiated acute recurrent from chronic residual thrombi with a sensitivity of 95% and specificity of 100% with good interobserver agreement. 50 A prospective outcome-management study is in progress. 18F-fluorodeoxyglucose positron emission tomography (18F-FDG-PET) is also being investigated as a potential imaging tool to distinguish the acuity of DVT. 51 , 52 , 53
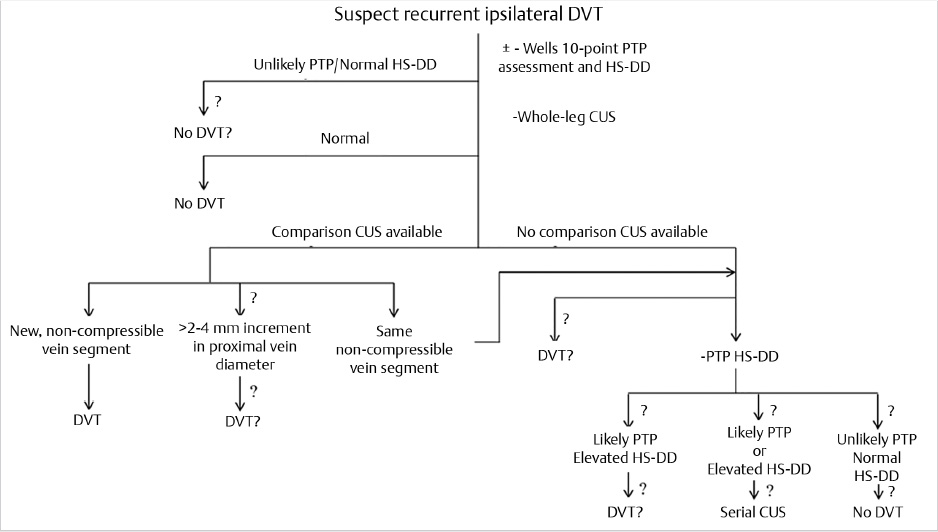
2.4.4 The Diagnosis of Pulmonary Embolism
The underdiagnosis of PE has long been a concern, but recent studies suggest there may be increasing overdiagnosis of PE in community settings. CTPA utilization to diagnose PE has increased between 4- and 27-fold in recent years. 54 During this time, the age-adjusted incidence of PE increased by 80%, but PE mortality rates did not decrease. 55 These data may suggest that some detected PE either are clinically inconsequential because of small size and low recurrence risk, were asymptomatic incidental diagnosis, or were false-positive diagnoses. 55 Patients at very low risk for PE now undergo unnecessary diagnostic evaluation as indicated by the increasingly very low yield of PE diagnoses by CTPA, currently ranging from just 2.7 to 10% in U.S. emergency departments. 56 , 57 As many as 25% of currently detected PE may be false-positive diagnoses when the CTPA images undergo reinterpretation by a panel of subspecialty chest radiologists. 58 There may be multiple explanations for excessive use of CTPA and HS-DD to diagnose PE. These include clinician desire to rule out a potentially fatal diagnosis at any cost, clinician fear of litigation if a PE diagnosis is missed, patient expectations, the easy, immediate availability of CTPA, and clinician lack of knowledge about or simple failure to use evidence-based PE diagnostic algorithms. 25 A recent study found that only 45% of CTPA studies were ordered appropriately according to the results of PTP assessment and HS-DD measurement. 59 Over one-half of clinicians reported that they used formal PTP assessment tools in less than one-half of suspected PE cases. 60
Several new approaches have been proposed to reduce excessive diagnostic testing for PE and consequent overdiagnosis and overtreatment, unnecessary radiation exposure and expense, and other potential complications of CTPA. 25 , 26 First, it may be useful to increase the utilization of evidence-based PTP assessment and HS-DD by requiring these evaluations on CTPA order sheets and/or by incorporating the necessary clinical decision support into the electronic medical record. A systematic review of recent studies found that implementation of the Wells criteria for PE diagnosis increased the yield of CTPA by 33%. 56 A recent systematic review suggested that the modified and simplified Wells rules may be slightly more useful than the revised and simplified revised Geneva models in primary care settings. 61 Table 2.3 shows the simplified Wells rule and revised Geneva score. In contrast, a previous direct comparison of these rules in an outcome-management study found them equivalent, although the revised Geneva score has been validated only in outpatients. 62 Some investigators propose that clinician gestalt, especially by more experienced clinicians, is potentially equivalent to use of the formal PTP assessments. 63
A second approach reduces unnecessary testing with HS-DD in patients with very low probability of PE by combining the Pulmonary Embolism Rule-Out Criteria (PERC) with the Wells or revised Geneva models. The PERC criteria are listed in Table 2.4. 64 A meta-analysis of 12 studies found that when the PERC criteria were applied to patients with low PTP assessment assessed by a validated clinical decision rule, and all 8 PERC criteria were met, the proportion of missed PEs was only 0.3%, eliminating the need for HS-DD testing in 22% of patients with suspected PE. 25 , 64 However, the PERC criteria may only be applied to patients with low PTP. 25
Age < 50 y |
Initial heart rate < 100 beats/min |
Initial room air oxygen saturation > 94% |
No unilateral leg swelling |
No hemoptysis |
No surgery or trauma within 4 wk |
No history of venous thromboembolism |
No estrogen use |
A third approach to reduce unnecessary CTPA imaging is to use age-adjusted normal/abnormal cut-off levels for HS-DD in order to improve the specificity of the HS-DD test for PE. HS-DD levels increases progressively with age in the general population such that 90% of persons aged ≥ 80 years evaluated for suspected PE will have an HS-DD ≥ 500 ng/mL. 65 A meta-analysis 66 and a large prospective management trial 67 have evaluated the safety of using an age-adjusted, HS-DD cut-off level in patients aged > 50 years. In these studies, the HS-DD cut-off was the patient’s age multiplied by 10 (in ng/mL), rather than the standard cut-off of 500 ng/mL, to help rule out PE. In patients aged over 50 years with a low, moderate, or unlikely PTP and a normal, age-adjusted HS-DD, the 3-month incidence of VTE was just 0.3% (95% CI: 0.1–1.7). Thus, in these studies, use of an age-adjusted HS-DD allowed PE to be ruled out without diagnostic imaging in an additional 12% of patients aged over 50 years and in 30% of patients aged ≥ 75 years. 67 Based on these studies, in 2015 the American College of Physicians recommended the diagnostic approach in Fig. 2.6. 25
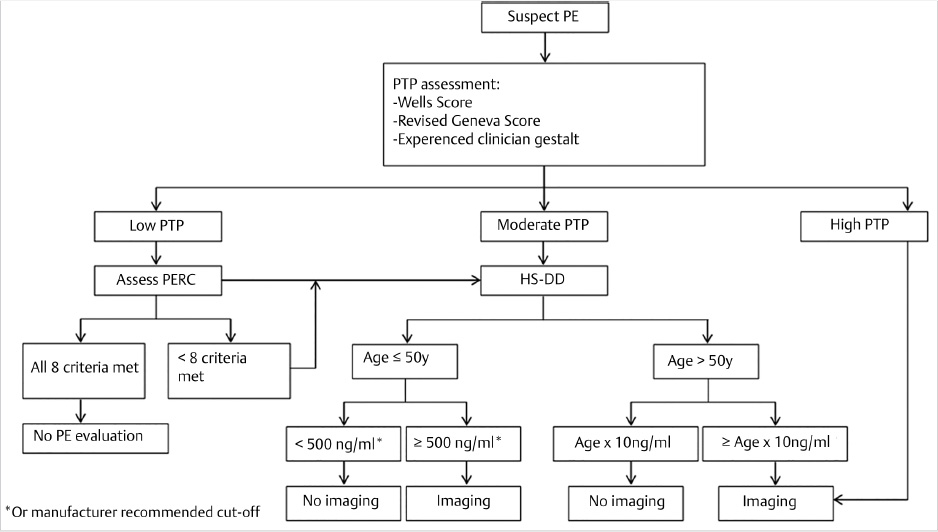
Most guidelines favor CTPA to confirm the diagnosis of PE in patients with an elevated HS-DD or high PTP in both outpatients and inpatients in the absence of contraindications. 25 , 26 An unresolved controversy is the clinical significance and management of isolated, small subsegmental pulmonary emboli (SSPE), which constitute up to 15% of all detected PE. 32 , 68 , 69 No prospective outcome-management studies are yet available to define optimal management of isolated SSPE, although such a trial is in progress in patients with SSPE who have normal bilateral CUS and no cancer (NCTO1455818). Some detected SSPE likely represent false-positive diagnoses, while others may carry no clinical significance. 32 , 55 Small retrospective studies have come to opposing conclusions as to whether treatment is indicated. 70 , 71 If bilateral CUS is normal, the 2016 Chest Expert Panel Report suggests that the decision to anticoagulate patients with SSPE (that has been confirmed by an experienced chest radiologist) depends on balancing the presence and strength of VTE recurrence risk factors, bleeding risk, baseline cardiopulmonary reserve, symptom severity, and patient preference (Table 2.6). 32
In the presence of relative contraindications to CTPA (e.g., renal insufficiency, severe contrast allergy, or a desire to limit radiation exposure, particularly in women under age 50 and in patients with prior CTPA or CT exposure), ventilation-perfusion lung scintigraphy combined with PTP assessment, HS-DD, and serial bilateral CUS can safely exclude and diagnose PE as well as CTPA, as demonstrated in outcome-management studies. 72 , 73 Fig. 2.7 shows the diagnostic algorithm from one of the studies. 72
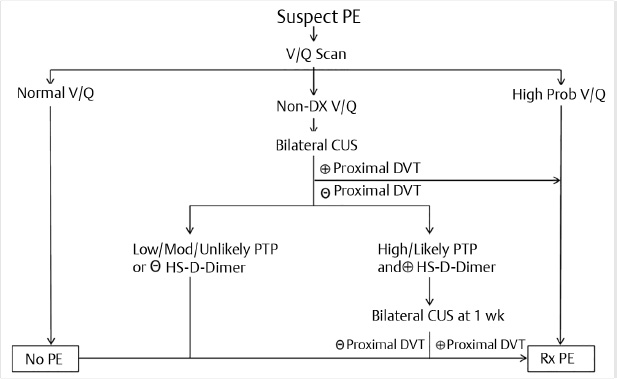
2.5 Acute Treatment of Venous Thromboembolism
The cornerstone of the acute treatment of VTE, once VTE has been diagnosed or is strongly clinically suspected, is the use of an antithrombotic agent with a rapid onset of action. 54 , 74 This helps prevent thrombus propagation or embolization. While traditionally accomplished through a parenteral antithrombotic agent, including intravenously or subcutaneously delivered unfractionated heparin (UFH), subcutaneous low-molecular-weight heparin (LMWH), or subcutaneous fondaparinux, in recent years the approval of the DOACs provides alternative therapeutic tools for providers. The DOACs, discussed in more detail below, include rivaroxaban, dabigatran, apixaban, and edoxaban (Table 2.5). As discussed below, in some instances the use of a DOAC for the acute treatment of VTE still requires lead-in therapy with a heparinoid, while other DOACs may be used as monotherapy without initial heparin therapy. While the use of UFH is declining for the acute treatment of VTE, particularly as more patients are treated in the outpatient setting, rather than being hospitalized, UFH remains a useful agent in patients with severe renal insufficiency or patients with a high bleeding risk where rapid anticoagulant reversal may be needed. Subcutaneous LMWH has more predictable pharmacology than UFH, allowing for outpatient VTE treatment with fixed, weight-based dosing. LMWH is also associated with fewer thrombotic complications, less major bleeding, and a mortality benefit when compared to UFH for VTE treatment. 75 In some patients, routine lab monitoring while LMWHs are being used may not be necessary.
The outpatient treatment of VTE is well established for appropriate patients with DVT without PE. Consistent with this, recent guidelines emphasize that patients with DVT alone may generally be treated as outpatients. Emerging data suggest that in carefully selected, low-risk patients with acute PE, outpatient therapy may also be safe and cost-effective, 32 , 76 , 77 , 78 , 79 although some uncertainties remain with regard to appropriate patient selection and treatment.
Often initiated concurrent with antithrombotic therapy, warfarin was the cornerstone of oral VTE treatment for nearly 60 years until the introduction of DOACs. Warfarin’s narrow therapeutic window and numerous food and drug interactions necessitate routine INR monitoring for the duration of therapy. DOACs are a class of oral anticoagulants that more directly target specific coagulation factors. The DOACs currently FDA-approved for the treatment of VTE include dabigatran, rivaroxaban, apixaban, and edoxaban. Each of these agents has unique pharmacology and pharmacokinetics (Table 2.4). In addition, indications and dosing of the DOACs vary. A comprehensive review of each of these DOACs is beyond the scope of this chapter and the reader is referred to each product’s package insert and several recent reviews for more information. 54 , 74 , 80 , 81 , 82
Dabigatran is a direct thrombin inhibitor that competitively binds to soluble and fibrin-bound thrombin, inhibiting the conversion of fibrinogen to fibrin and subsequent thrombus formation. Rivaroxaban, apixaban, and edoxaban are factor Xa inhibitors that selectively block the active site of factor Xa, inhibiting the coagulation cascade without the use of a cofactor (antithrombin). Additional appealing attributes of the DOACs include fewer drug interactions and more predictable pharmacology than warfarin, minimizing the need for routine lab monitoring and frequent dose adjustments. 83 Randomized clinical VTE treatment trials have demonstrated the DOACs are noninferior to warfarin with respect to efficacy, and some agents may have superior safety profiles. 84 , 85 , 86 , 87 , 88 , 89 Some of these trials are discussed in more detail below.
The RE-COVER 88 , 89 and Hokusai VTE 85 studies compared warfarin to dabigatran and edoxaban, respectively. One design of these studies to highlight was the requirement of an initial course of heparin (UFH or LMWH) prior to DOAC initiation. In comparison, the EINSTEIN 86 , 87 and AMPLIFY 84 studies compared conventional therapy to rivaroxaban and apixaban as monotherapy without preceding heparin. These studies also established that the bleeding risk with apixaban, edoxaban, and rivaroxaban is lower compared to warfarin. Dabigatran has a similar bleeding risk profile to warfarin. Given the generally favorable bleeding profiles, the ability to forego parenteral anticoagulation with certain agents, and avoidance of routine lab monitoring, VTE treatment with DOACs provides some advantages for health care providers and carefully selected patients.
Unlike heparin and warfarin that can be reversed with protamine and vitamin K or plasma administration, respectively, DOAC reversal can be more challenging. Currently, the only FDA-approved reversal agent for any DOAC is idarucizumab: a monoclonal antibody fragment specific to dabigatran. 90 Specific reversal agents for factor Xa inhibitors (e.g., rivaroxaban, apixaban, and edoxaban) are in late-stage development. 91 , 92 , 93 Four-factor PCC (prothrombin complex concentrates), comprised of coagulation factors II, VII, IX, and X, has been evaluated in small studies and may be effective in correcting coagulation assay derangements following rivaroxaban administration. 94 Activated forms of PCC (aPCC) or recombinant FVIIa may be effective in reversing effects of dabigatran. 95 Unfortunately, observational data on novel oral anticoagulant–associated intracranial hemorrhage failed to demonstrate significant improvements in clinical outcomes among patients receiving PCC. 96 Until specific reversal agents for factor Xa inhibitors are approved, management of major or life-threatening bleeding remains supportive care with blood-product transfusion and mechanical intervention whenever possible (e.g., endoscopy, manual compression of compressible sites, intravascular embolization). With major or life-threatening bleeding associated with dabigatran use, idarucizumab administration should be considered.
Regardless of the antithrombotic agent and/or oral anticoagulant chosen, baseline assessment of bleeding risk, measurement of renal and hepatic function, platelet count, baseline coagulation measurements, and a thorough medication review are essential. 97 Patient and caregiver education is also paramount and may help inform decisions regarding the anticoagulant, intensity, and duration of therapy chosen. Patient preferences are especially important to ascertain in settings where there may be equipoise on anticoagulation initiation and/or duration (e.g., for a single, unprovoked distal DVT). Once anticoagulation is initiated, regular monitoring of renal function and other indicated laboratory tests, and medication compliance is important in older adults, given their increased bleeding risk. 97 In some settings, anticoagulation clinics, which historically managed patients on a vitamin K antagonist (VKA), now also follow patients on DOACs. Anticoagulation clinics may serve as key resources for patients and providers, helping ensure compliance, providing patient education, and assisting with periprocedural anticoagulation management. Patient-focused educational materials for anticoagulation are also recommended. 98 , 99
2.5.1 Thrombolysis for Acute, Massive Pulmonary Embolism
Adverse outcomes in patients with PE vary, based in part on initial presentation and the presence or absence of sustained hypotension (e.g., systolic blood pressure < 90 mm Hg or requiring inotropic support; commonly termed “massive PE”). 32 , 100 While published mortality rates vary, hypotension and/or cardiopulmonary arrest in patients with massive PE is associated with a significantly higher risk of short-term death than patients with submassive PE (e.g., systolic blood pressure > 90 mm Hg but with evidence of right heart strain or dysfunction) or patients with nonmassive PE (systolic blood pressure > 90 mm Hg and no evidence of right heart strain). For example, in-hospital mortality in patients enrolled in the Management Strategy and Prognosis of Pulmonary Embolism Registry (MAPPET) ranged from 8.1% (hemodynamically stable) to 25% (cardiogenic shock) to 65% (cardiopulmonary resuscitation). 100 , 101 Similarly, 90-day mortality rates in patients studied in the International Cooperative Pulmonary Embolism Registry (ICOPER) were 52.4% in PE patients with hypotension versus 14.7% in PE patients without hypotension upon initial presentation. 60 , 102 Given this high mortality, patients with confirmed massive PE and no contraindications (Table 2.5) are often considered for fibrinolysis. While the fibrinolytic agent chosen, as well as dosing and route of administration, may vary, the use of fibrinolytics improves lung perfusion and reduces mortality in patients with massive PE. 100 , 103 , 104 , 105 These clinical benefits with fibrinolytics are offset by an increased risk of major bleeding (10% or greater in unselected patients) and intracranial hemorrhage (2 to 3%) compared to anticoagulation alone. 101 , 106 , 107 , 108 Recent meta-analyses suggest that in massive PE, the number needed to treat to prevent recurrent PE or death was 10, while the number needed to harm was 8. 109 As such, current guidelines recommend the administration of fibrinolytics in patients with massive PE who do not have a high bleeding risk. 32 , 100
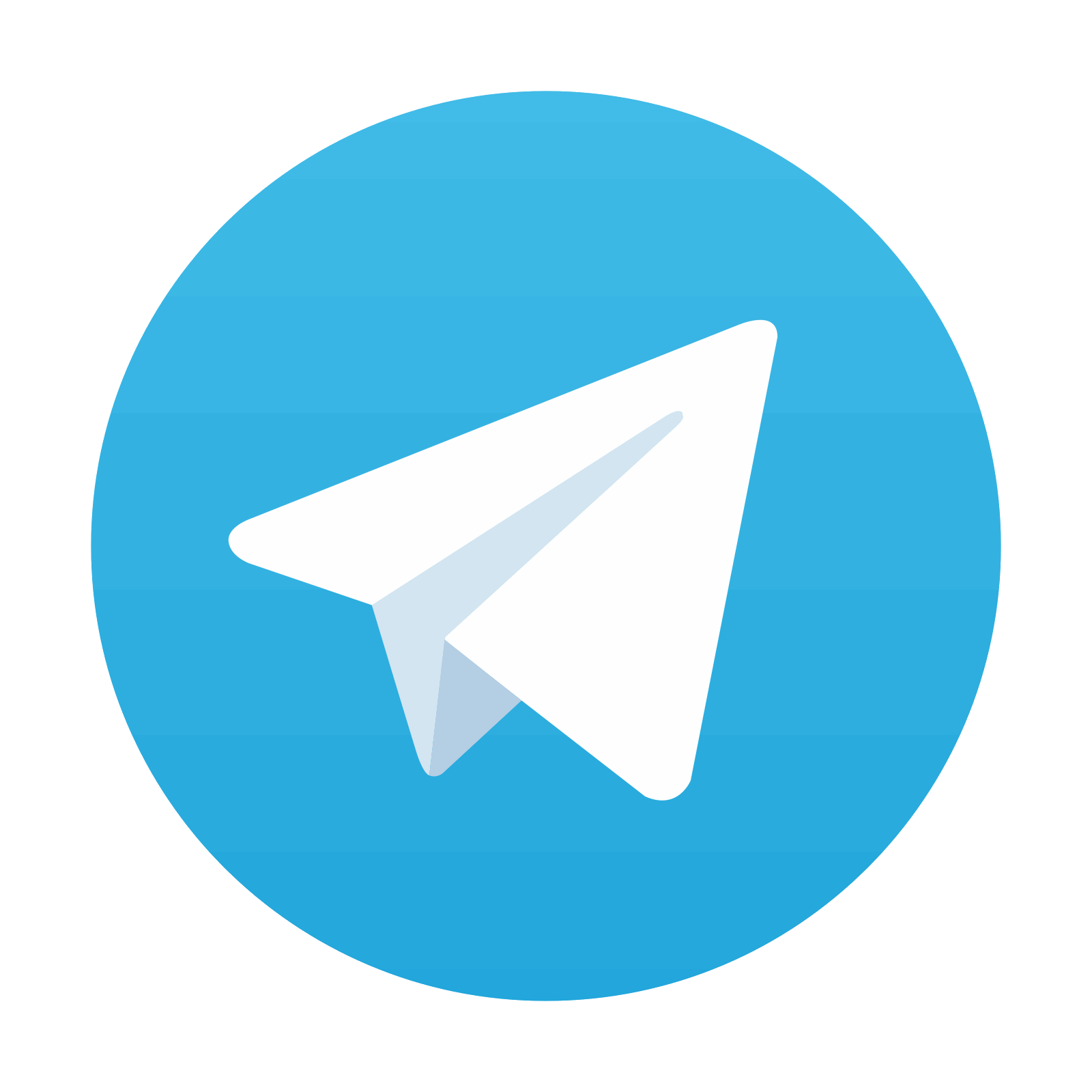
Stay updated, free articles. Join our Telegram channel

Full access? Get Clinical Tree
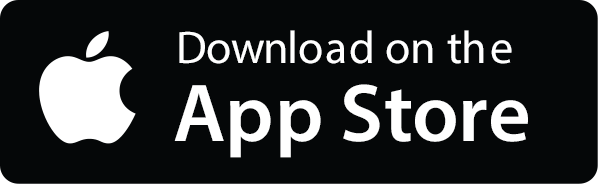
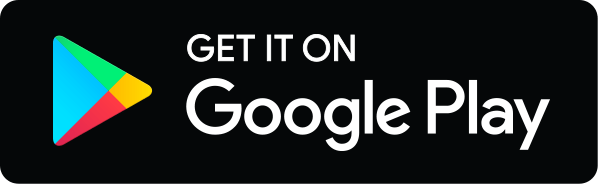
