10 Intracranial Hemorrhage
10.1 Aneurysmal Subarachnoid Hemorrhage
10.1.1 Clinical Case
A 55-year-old male with sudden onset of worst headache of life.
10.1.2 Description of Imaging Findings and Diagnosis
Diffuse hyperdense blood in the subarachnoid space centered in the interhemispheric fissure. There is also a small amount of right-sided subdural hemorrhage. Severe hydrocephalus. Findings most consistent with a ruptured anterior communicating artery aneurysm (Fig. 10.1).
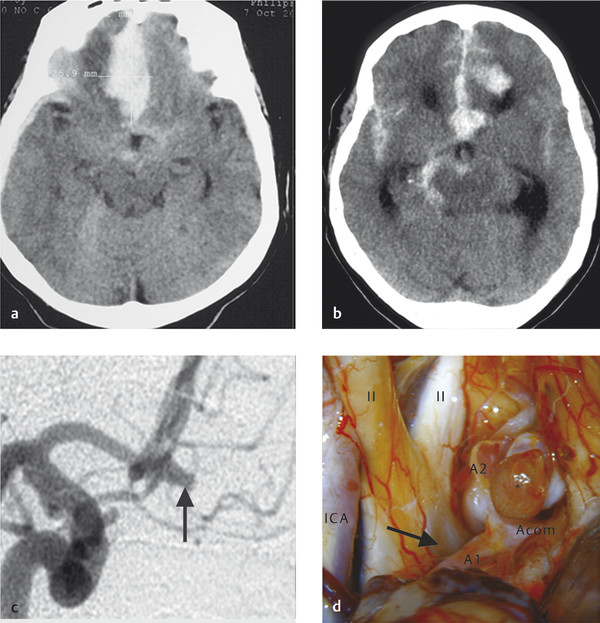
10.1.3 Background
Aneurysmal SAH is the most common form of nontraumatic SAH affecting up to 20 individuals per 100,000 persons/year. Rupture of a cerebral aneurysm results in the extravasation of blood into the subarachnoid space, which triggers a cascade of events that can result in severe disability or death. Failure to diagnose and treat an aneurysmal SAH can result in further impairment due to aneurysm rebleeding and delayed cerebral ischemia from cerebral vasospasm. While lumbar puncture is the gold standard for the diagnosis of SAH, CT has emerged as the diagnostic modality of choice with sensitivity and specificity of over 99% in the first days after the SAH.
It is important for neuroradiologists to understand some basic tenants of the pathophysiology of aneurysmal SAH. First, in general, once an aneurysm ruptures, the rupture point soon thromboses resulting in temporary cessation of bleeding. For this reason, active extravasation is rarely identified on cross-sectional imaging. However, rebleeding of an unsecured aneurysm is relatively common as the rate ranges from 1–2% per day for the first month following the initial insult. Rebleeding has a 60% mortality and severe disability rate; hence, the importance of identifying the aneurysm. Hydrocephalus is a relatively common complication of aneurysmal SAH and often presents with slowly progressive reduction in the level of consciousness, downward deviation of the eyes, and small pupils from pressure on the tectal area. Early identification of hydrocephalus is important so that EVD placement can be considered. Acute hydrocephalus typically occurs in the first 3 days following aneurysm rupture. Symptomatic and delayed cerebral infarction are also relatively common (10–20% of cases). These are important causes of SAH-associated morbidity and typically occur from 5–14 days following the initial insult. The neuroradiologist plays an essential role in the identification and prediction of these pathophysiologic processes.
10.1.4 Imaging Findings
The diagnosis of aneurysmal SAH on imaging is straight forward.
Non-contrast CT is the imaging modality of choice as it easily identifies hemorrhage and allows for quick identification of hydrocephalus. SAH is identified as hyperdense blood products, usually centered in the suprasellar cistern with extension into the interhemispheric and sylvian fissures. Sensitivity of CT for the identification of SAH decreases over time from about 99% in the first 24 hours to 30–50% after 1 week. In cases where CT is equivocal, MRI with FLAIR (fluid attenuation inversion recovery) or T2* sequences is helpful. Potential mimics of SAH include iodinated contrast, diffuse basal meningitis (i.e., tuberculosis), and pus.
The modified Fisher scale is the most commonly used scale in grading SAH and is described in Table 10.1. It serves as a good template for describing imaging findings in aneurysmal SAH and is a useful tool in predicting future risk of vasospasm. Key points are the following: (1) thin SAH is <1-mm thick and thick SAH is >1-mm thick, (2) presence of IVH is key, and (3) the radiologist must seek out the presence of areas of focal SAH. Risk of vasospasm ranges from 24% for a mFisher of 1 to 40% for a mFisher of 4. Another key point is that the Fisher score does not account for degree of hydrocephalus. Thus, the neuroradiologist must be attuned to imaging findings of hydrocephalus including (1) temporal horn expansion, (2) convexity of the third ventricular walls, (3) rounding of the frontal horns, (4) sulcal effacement, and (5) ventricular enlargement out of proportion to sulcal dilatation.
Aneurysm detection is typically performed with CTA in most centers. However, given the fact that most ruptured aneurysms are treated endovascularly, some centers have advocated for skipping the initial CTA and going directly to diagnostic cerebral angiography. While it may be more cost effective to go straight to digital subtraction angiography (DSA), there is some benefit in doing an initial CTA as it can triage patients who are not typically endovascular candidates to surgery and allow them the possibility of skipping the DSA. DSA with 3DRA is the gold standard in aneurysm detection and characterization. CTA has a sensitivity of 100% for identifying ruptured aneurysms ≥4 mm in size but just 92% for aneurysms <4 mm. Thus, a negative CTA in the setting of diffuse SAH should prompt a diagnostic cerebral angiogram. 3D and MPR reconstructions are often essential in identifying smaller aneurysms on CTA. In cases of aneurysm multiplicity, one can refer to aneurysm size, morphology, and wall-imaging characteristics to identify the culprit lesion. In general, the ruptured aneurysm is the larger and more irregular lesion. MRI vessel wall imaging (VWI), described in a previous chapter may also be helpful as some studies have suggested that ruptured aneurysms are more likely to demonstrate circumferential wall enhancement than unruptured lesions.
It is important to point out that non-contrast CT can be very helpful in identifying the bleeding site, particularly in patients with multiple aneurysms. The location of the thickest/highest density blood is often the site of the aneurysm. If there is dense thick blood in the interhemispheric fissure, then an Acom aneurysm can be considered, sylvian fissure indicates MCA, interpeduncular cistern indicates basilar tip, and asymmetric blood in the suprasellar cistern suggests an ICA/Pcom etiology (Fig. 10.2).
Serial imaging is often performed in aSAH patients during their hospitalization. Over time, one should expect reduction in blood volume and density and improvement in hydrocephalus. Around days 5–14, screening for cerebral vasospasm is often performed. Vasospasm is identified by narrowing of intracranial arteries on CTA, MRA, or DSA. The narrowing is often most severe at the location of the densest blood. Perfusion imaging, particularly CT perfusion, is very helpful in determining how flow limiting the vasospasm is. Delayed cerebral ischemia from vasospasm is associated with high rates of infarction and poor neurological outcome. It is important to point out that about 20% of patients without cerebral vasospasm will develop DCI suggesting that factors other than vasospasm play a role. CTP perfusion studies of aSAH patients have found that patients with perfusion changes on CTP have a 23 times higher odds of DCI than patients with normal CTP. In addition, CTP performed within 4–14 days after aSAH predicts DCI. The best parameters to study in such cases are CBF and MTT.
One common imaging finding that is often not picked up on during the initial evaluation of SAH patients is the presence of blood in the globes (i.e., Terson’s syndrome). This finding is present in 20–50% of SAH patients and can be identified as layering blood in the posterior portion of the globe. Terson’s syndrome is a common cause of long-term vision loss in SAH and can be treated with vitrectomy (Fig. 10.3).
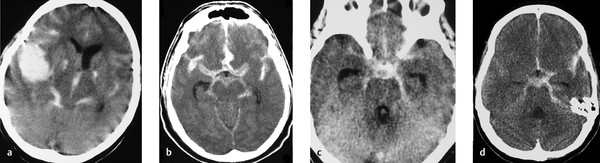
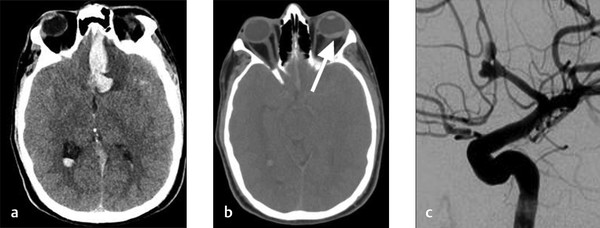
10.1.5 What the Clinician Needs to Know
Extent and distribution of subarachnoid blood with focus on parameters identified in Fisher scale.
The presence or absence of hydrocephalus and intraventricular blood.
Aneurysm location.
The presence or absence or risk factors for vasospasm and delayed cerebral ischemia. Discussion of role of perfusion imaging in prognosticating DCI can help guide critical care physicians in how aggressive they need to be in medical management. In setting of suspected vasospasm, CTP can help identify severity of perfusion deficit.
10.1.6 High-yield Facts
Sensitivity of CTA is 92% for aneurysms <4 mm in size. A negative CTA in the setting of a suspected aneurysmal SAH should prompt an immediate DSA.
Cerebral vasospasm is most common on days 5–14 post-SAH. CTA and CTP are useful screening tools in evaluating both anatomic and functional effects of vasospasm.
In setting of multiple aneurysms, factors that can help identify the culprit lesion include aneurysm wall enhancement on MRI, aneurysm morphology, aneurysm size, and location of the densest amount of blood products.
Further Reading
[1] de Oliveira Manoel AL, Mansur A, Murphy A, et al. Aneurysmal subarachnoid haemorrhage from a neuroimaging perspective. Crit Care. 2014; 18(6):557 [2] Dupont SA, Wijdicks EFM, Lanzino G, Rabinstein AA. Aneurysmal subarachnoid hemorrhage: an overview for the practicing neurologist. Semin Neurol. 2010; 30(5):545–55410.2 Non-Aneurysmal Perimesencephalic Hemorrhage
10.2.1 Clinical Case
A 43-year-old male with post-coital severe headache. No loss of consciousness (Fig. 10.4).
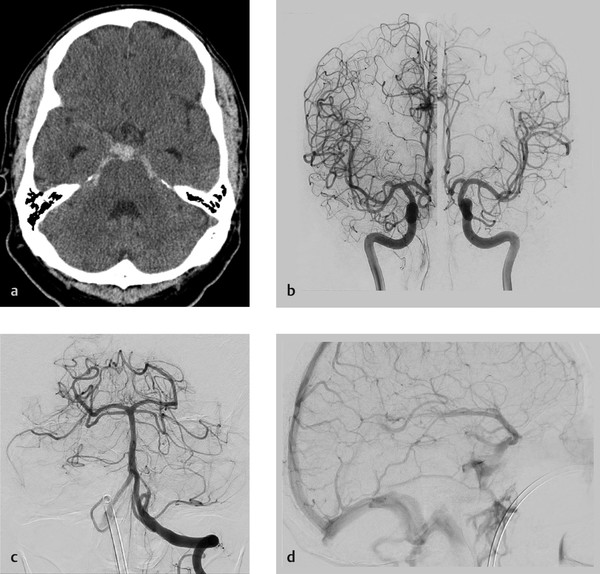
10.2.2 Description of Imaging Findings and Diagnosis
Diagnosis
Focal SAH isolated to the perimesencephalic cistern in the setting of a negative CTA and cerebral angiogram. Consistent with non-aneurysmal perimesencephalic hemorrhage.
10.2.3 Background
Non-aneurysmal perimesencephalic subarachnoid hemorrhage (pSAH) was first described by van Gijn in 1985 as an SAH in which blood was limited primarily to the perimesencephalic cisterns with no evidence of aneurysm on angiography. Definition of pSAH is somewhat variable across studies; however, in general, most authors seem to agree that for an SAH to be considered a pSAH it has to meet the following imaging criteria: (1) center of hemorrhage located in front of the brainstem, (2) no blood in the interhemispheric or lateral Sylvian fissures (except for minute amounts), (3) minimal intraventricular hemorrhage, and (4) no intraparenchymal hemorrhage. In addition to imaging findings, patients need to have minimal symptoms beyond a severe headache. Non-aneurysmal pSAH patients are typically alert, orientated, and have a Glasgow Coma Scale of 15. These relatively minor symptoms are the reason why many authors believe that non-aneurysmal pSAH is the result of a venous hemorrhage rather than an arterial bleed. The differential diagnosis of a pSAH is fairly broad. The prevalence of a saccular aneurysm in the setting of a pSAH ranges from 1–9% depending on the study, with most aneurysms being located in the posterior fossa. Other potential etiologies include arterial dissection/dissecting aneurysm, posterior fossa or spinal vascular malformation, and hypervascular tumors like hemangioblastomas. In most cases, the hemorrhage is idiopathic with no structural lesion to account for the hemorrhage.
Many authors have claimed that the etiology of idiopathic pSAHs is venous in nature. Many patients with pSAH report performing some variant of a Valsalva maneuver at the time of the initial ictus. It is thought that the Valsalva maneuver can result in intracranial venous engorgement and rupture due to the fact that Valsalva increases intrathoracic pressure, which blocks internal jugular venous return thus resulting in increased intracranial venous pressure. A number of studies have found that patients with non-aneurysmal pSAH have variant venous drainage patterns as well, further lending support to the venous hypothesis. Patients with pSAH have been found to have more primitive drainage patterns of the basal vein of Rosenthal including drainage directly into the dural sinuses (cavernous sinus via the uncal vein, the superior petrosal sinus via the tentorial sinus or the pontomesencephalic vein) rather than into the Galenic system as classically described. The direct connection of the perimesencephalic and basal veins with the dural sinuses may predispose to sudden increases in venous pressure and venous rupture during Valsalva. Other reported venous culprits for pSAH include the vein of Galen stenosis and straight sinus stenosis (Fig. 10.5).
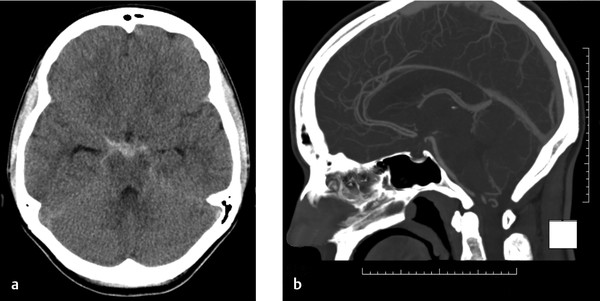
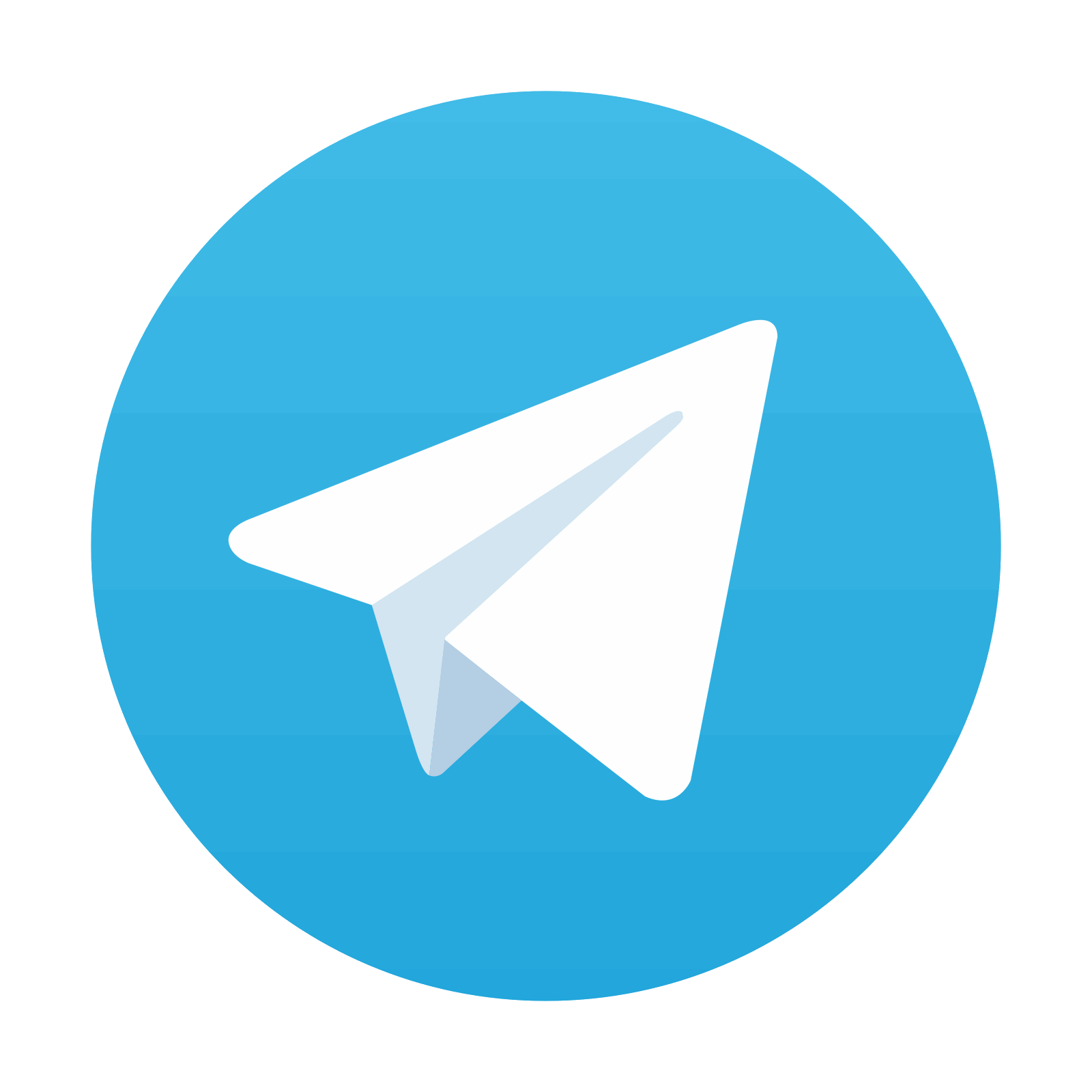
Stay updated, free articles. Join our Telegram channel

Full access? Get Clinical Tree
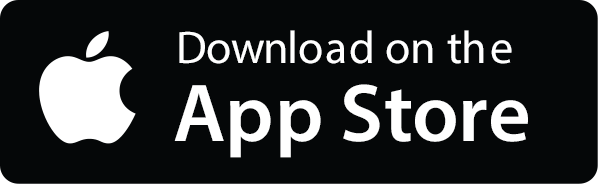
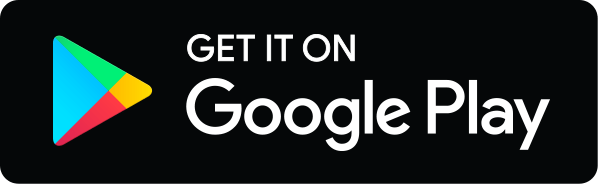
