11 Spinal Vascular Disease
11.1 Spinal Cord Infarction
11.1.1 Clinical Case
A 67-year-old male with sudden onset paraplegia following a motor vehicle accident (Fig. 11.1).
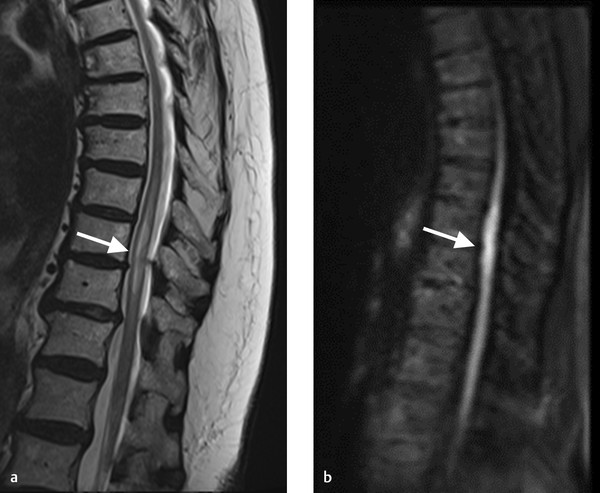
11.1.2 Description of Imaging Findings and Diagnosis
Diagnosis
T2 hyperintensity in the anterior spinal cord with associated restricted diffusion. There is a small disc protrusion at the level of the signal abnormality. Findings consistent with cord infarct, possibly related to fibrocartilaginous embolism.
11.1.3 Background
Given the dense anastomotic network, spinal cord infarcts are rare and account for 8% of myelopathies. Spinal cord infarction presents with abrupt onset of pain in 60–70% of cases. Pain is generally located at the dermatomal level of the cord along with weakness and/or sensory loss, with the possible loss of sphincter tone. In spinal cord arterial infarcts, the constellation of presenting symptoms is determined by the level and artery affected. Anterior spinal artery infarcts are the most common and can be seen in the setting of aortic or spinal procedures. Anterior spinal artery infarcts can cause respiratory compromise and tetraparesis if in the upper cervical segments, and paraplegia in the thoraco-lumbar segments due to corticospinal tract involvement and loss of pain and temperature due to spinothalamic involvement. Posterior spinal artery infarcts are rare and affect the dorsal column and therefore present with isolated loss of vibration and proprioception. Combined anterior and posterior spinal artery infarcts can also be seen from mechanical hyperflexion or hyperextension injuries.
Spinal sulcal artery infarcts presents with a sulcocommisural syndrome also known as Brown Sequard syndrome due to “hemi-cord” involvement. This includes ipsilateral weakness, loss of vibratory sense, and proprioception and contralateral loss of pain and temperature due to the spinothalamic tracts decussation. Central spinal artery infarcts are most likely due to prolonged hypoperfusion from hypotension or cardiac arrest and present with bilateral loss of pain and temperature without any weakness or proprioceptive loss. A complete transverse infarct would lead to bilateral loss of sensation and weakness and more likely due to embolic etiology. Examples of different territories of spinal cord infarcts is provided in Fig. 11.2.
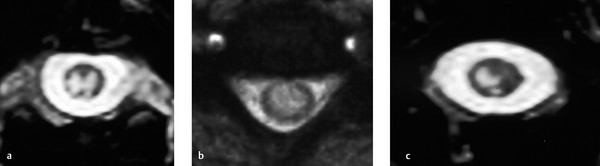
There are numerous etiologies implicated in spinal cord infarctions including aortic disease, iatrogenic causes, vertebral dissection, emboli (large artery or cardiac), hypercoagulable states (i.e., sickle cell disease, antiphospholipid syndrome, malignancy, etc.), decompression sickness, vasculitis, systemic hypotension or global hypoperfusion from cardiac arrest, radicular artery compression, discocartilagnious emboli, and trauma. However, approximately one-third of spinal cord infarcts do not have an identifiable etiology and therefor deemed to be idiopathic or cryptogenic. Treatments include blood pressure augmentation and sometimes, lumbar drain placement.
11.1.4 Imaging Findings
MRI is the preferred imaging modality for diagnosis of a spinal cord infarction; however, it is not very sensitive in detecting acute spinal cord infarction. Urgent MRI is vital in excluding other diagnosis on the differential with the most urgent being spinal cord compression. MRI can also be useful in differentiating spinal cord infarction from other vascular myelopathies (i.e., vascular malformations) or autoimmune myelopathies. Lumbar puncture is generally recommended to rule out an inflammatory etiology.
The ideal protocol for the assessment of spinal cord ischemia is sagittal spin-echo T2 or T2- STIR, sagittal spin echo T1, axial gradient echo T2 in the cervical spine and axial spin-echo T2 of the thoracic and lumbar spine. Diffusion-weighted imaging (DWI) should be performed in both the axial and sagittal planes in order to give the radiologist two shots at identifying the infarct. DWI is rife with technical challenges; however, due to motion artifact from physiological spinal cord movement, susceptibility artifacts and low signal-to-noise ratio due to the small pixel size required for appropriate imaging of the spinal cord. Contrast is not necessary but is helpful in excluding inflammatory, infectious, and neoplastic etiologies. The sensitivity of DWI in detecting acute spinal cord infarcts varies across studies, but more recent studies have suggested it is on the order of 90%. There is typically no role for spinal vascular imaging in the setting of spinal cord infarction. However, CTA of the neck, chest, abdomen, and pelvis can be helpful in identifying potential culprits such as atheroma, dissection or aneurysm (Fig. 11.3).
In the acute phase, spinal cord infarction presents with restricted diffusion and T2 hyperintensity (Fig. 11.1). Enhancement can occur in the subacute phase (Fig. 11.4). Infarction in the anterior spinal artery territory includes the anterior horns with or without adjacent white matter changes. Isolated gray matter changes are due to the fact that the gray matter is more vulnerable to ischemia. This gives a characteristic “owl eyes” appearance. Posterior spinal cord infarcts affect the posterior columns and/or surrounding white matter and can be unilateral or bilateral. Myelomalacia can be seen in the infarct territory in the chronic phase. Vertebral body infarct has been reported in the setting of spinal cord infarct as well and presents with high T2 signal and enhancement of the body and adjacent disc (Fig. 11.5). Hemorrhagic transformation is rare.
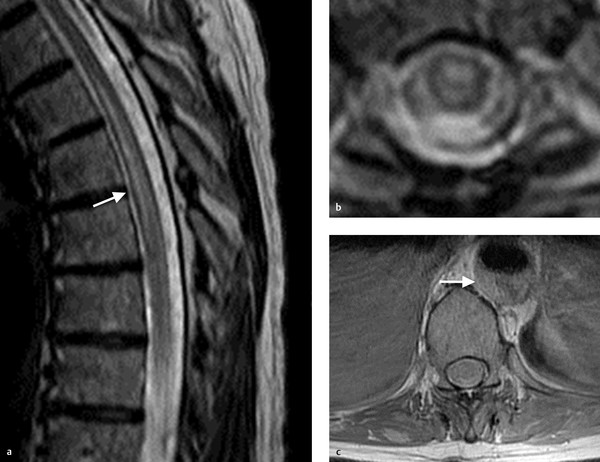
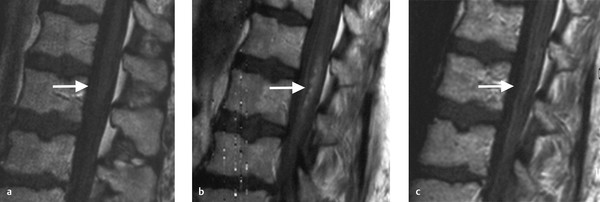
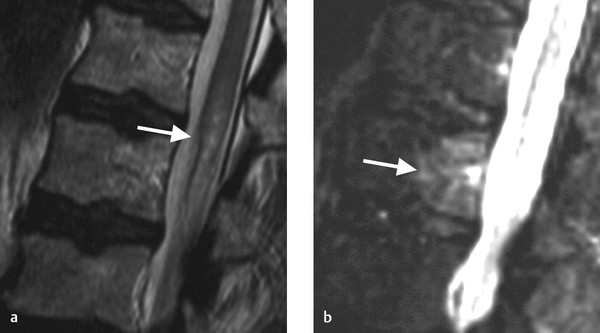
Distinguishing spinal cord infarction from other causes of myelopathy is essential. The most common mimicker is demyelinating disease. In demyelinating disease the lesions typically are smaller than cord infarct and involve the lateral and posterior aspects of the cord. Venous ischemia from congestive myelopathy (i.e., dural fistula) will result in cord expansion and diffuse T2 cord signal usually involving the conus and extending superiorly.
What the Clinician Needs to Know
Any imaging findings that can help point to an etiology for the cord infarct (i.e., dissection, aortic aneurysm, atheroma, etc.).
Distinguishing characteristics of spinal cord infarct from potentially reversible causes of myelopathy.
The presence of any mechanical injury which could have caused the infarct (i.e., cervical canal stenosis, compression of radicular artery by disc material, etc.).
11.1.6 High-yield Facts
DWI is essential to the diagnosis of spinal cord infarction.
The distribution of spinal cord infarct is highly dependent on the type of artery affected. Owl eye appearance is seen in anterior spinal artery infarct.
Spinal cord infarcts can enhance in subacute phase.
Further Reading
[1] Nogueira RG, Ferreira R, Grant PE, et al. Restricted diffusion in spinal cord infarction demonstrated by magnetic resonance line scan diffusion imaging. Stroke. 2012; 43(2):532–535 [2] Vargas MI, Gariani J, Sztajzel R, et al. Spinal cord ischemia: practical imaging tips, pearls, and pitfalls. AJNR Am J Neuroradiol. 2015; 36(5):825–83011.2 Spinal Dural Arteriovenous Fistula (MRI Findings)
11.2.1 Clinical Case
A 68-year-old right-handed white female who presents right lower extremity weakness with difficulty of getting off low chairs and difficulty going up stairs. Provided MRI interpreted as negative for cord or nerve root compression.
11.2.2 Description of Imaging Findings and Diagnosis
T2 hyperintensity of the conus with associated flow voids running up the dorsal aspect of the cord. Findings most consistent with spinal dural fistula or other spinal vascular malformation (Fig. 11.6).
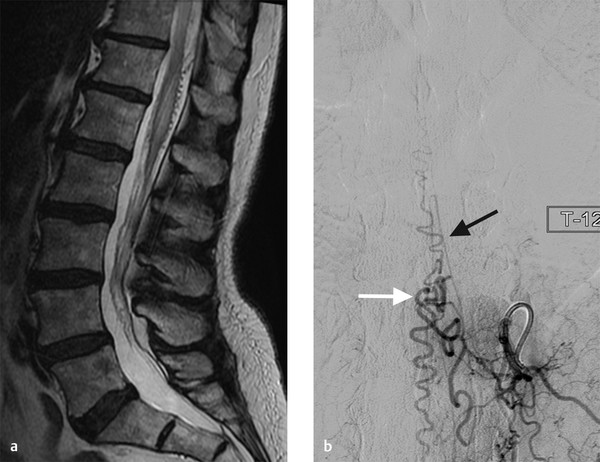
11.2.3 Background
Spinal dural arteriovenous fistulas (SDAVF) are spinal vascular lesions that classically present with vague symptoms such as leg dysesthesias and exertional leg weakness, but slowly progress to severe myelopathy with paraplegia and sphincter dysfunction. The slow and insidious onset of symptoms is generally considered to be the reason why so many of these lesions are diagnosed late or misdiagnosed. SDAVFs have been reported to be misdiagnosed and even treated as peripheral neuropathy, radiculopathies, multiple sclerosis, intramedullary tumors, neuromyelitis optica, and transverse myelitis. Furthermore, because of the demographic characteristics of patients affected by these lesions (typically, older men), they are often misdiagnosed as central spinal canal stenosis secondary to degenerative changes. The consequences of misdiagnosis are severe as each month in delay of diagnosis results in added morbidity which is often irreversible. With or without initial misdiagnosis, diagnostic delays are common and can be quite long. In fact, the estimated time from clinical symptom onset to diagnosis ranges from 11 to 27 months depending on the series.
It has been proposed that SDAVFs result from loss of normal physiologic control of the glomeruli of Manelfe, a structure located between two layers of dura mater that is composed of two or more arterioles converging with a vascular ball (glomerulus) and being drained by a single intradural vein. However, the means by which the glomeruli of Manelfe lose their ability to be physiologically controlled is still unknown. Following formation of the fistula between the radiculomeningeal artery and radicular vein, venous congestion along the longitudinal venous network draining the spinal cord can occur. Congestion is generally most marked in the conus due to its dependent location resulting in the classic sensorimotor deficits and bowel and bladder symptoms. Progression of the lesion results in increased venous congestion and, in turn, chronic hypoxia and progressive myelopathy with the worsening of symptoms. The onset of symptoms is often insidious and can take place years after the fistula develops. In general, when left untreated, symptomatic SDAVFs progress to severe irreversible myelopathy with paraparesis and sphincter dysfunction. While the natural history of asymptomatic, incidentally discovered SDAVFs is unknown, it is believed that they will progress to become symptomatic with progressive loss of radicular venous outlets and thus formation of venous congestion.
11.2.4 Imaging Findings
MRI is essential to diagnosis of SDAVFs. These lesions have a characteristic appearance which includes T2 signal intensity of the conus extending superiorly across multiple segments (95% of cases), serpiginous enlarged intradural vessels seen as flow voids on T2-weighted imaging that are more pronounced along the dorsal compared to the ventral aspect of the cord (96% of cases) and sometimes, gadolinium enhancement of the cord itself (80% of cases). Of note, cord enhancement is often patchy (Fig. 11.7). High conus signal is unrelated to the level of the fistula due to the fact that the pathophysiology of the lesion is due to venous congestion, which affects the inferior aspect of the cord first.
Despite the characteristic imaging findings, up to 50% of these lesions are missed on initial imaging evaluation (Fig. 11.7). Because most of these patients will only receive imaging of the lumbar spine without contrast during the initial evaluation of their symptoms, oftentimes the only sign of a SDAVF will be a slightly increased signal in the conus with flow voids. These “edge of the film” findings are commonly missed, highlighting the importance for the radiologist to specifically examine the conus in every lumbar spine MRI. In cases of patients with unexplained myelopathy, a spinal MRA should be considered as a SDAVF is present in up to 30% of these patients. Spinal contrast-enhanced, time resolved MRA has a high sensitivity for the detection of SDAVFs. In cases where there is a high clinical suspicion, conventional spinal angiography should be performed as it is both safe, and the gold standard for detection of SDAVFs. In cases where an artery cannot be accessed or assessed on the first attempt, there should be a low threshold for repeat angiography at a later date in order to ensure that all vessels are fully evaluated.
Of note, there are some imaging findings which can help in the localization of the fistula. One finding which has been recently described is the presence of a curvilinear vein extending from the sacrum to the conus. This is considered to be an enlarged vein of the filum terminale and is an indicator of a low-lying fistula (i.e., L3 and lower). This can help in guiding where to center a spinal MRA as well as guide the angiographer in knowing where to start the spinal angiogram (Fig. 11.8).
There are many tips and tricks to interpretation of spinal MRAs in localizing a fistula. One key thing to keep in mind is that this needs practice. One piece of advice is as follows: familiarize yourself with dural fistulas and how they look on conventional angiography. Trace out the feeding artery as it arises from the aorta and segmental artery and identify the draining radicular vein and dilated coronal venous plexus. When interpreting spinal MRAs, it helps to look at the images in axial, sagittal, and coronal planes. The coronal plane will be the most likely to demonstrate a similar finding to what is seen on angiography (Fig. 11.9). In general, what one is looking for is a prominent artery/vein coursing under the pedicle and along the expected course of the nerve root. It is important to distinguish between feeding arteries/arteriovenous fistula sites and prominent veins which may be draining the fistula. In order to do this, it helps to follow the course of the prominent foraminal vessel and determine if it connects with the aorta (in which case, this is likely the site of the fistula) or the vena cava (in which case it is a prominent draining vein).
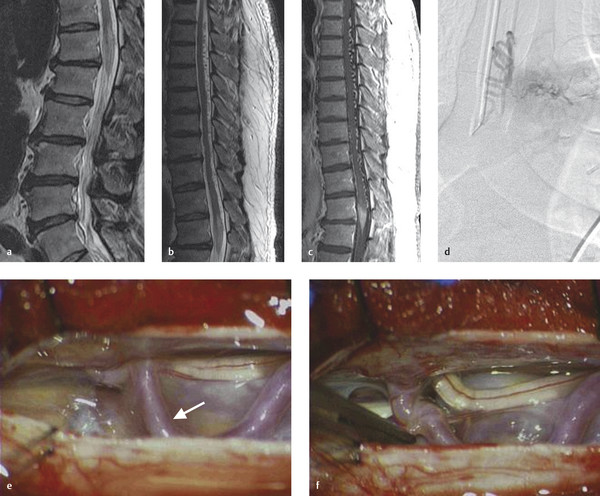
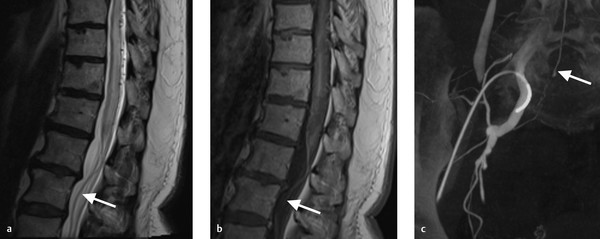
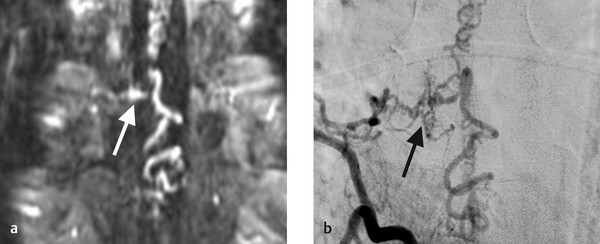
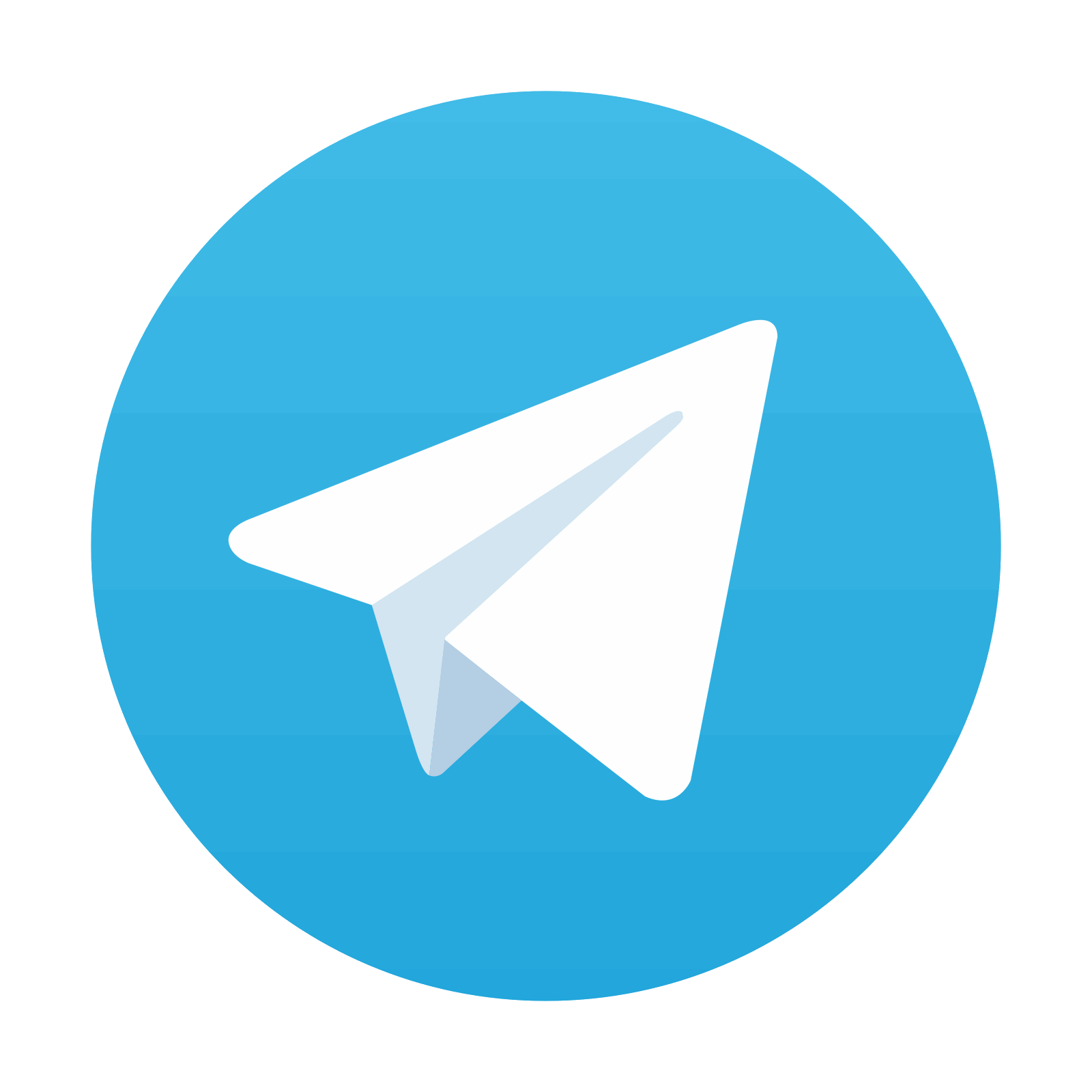
Stay updated, free articles. Join our Telegram channel

Full access? Get Clinical Tree
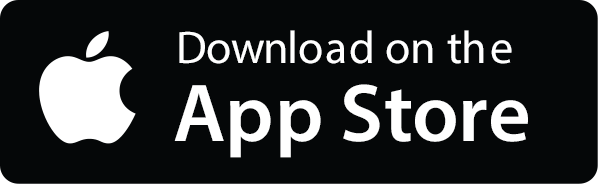
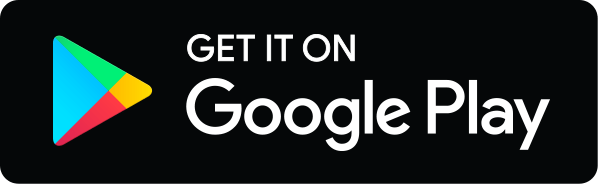
