12 Vascular Abnormalities
12.1 Introduction
The intracranial vasculature can have congenital and acquired abnormalities that result in injury to the brain parenchyma. Understanding the normal anatomy of the intracranial vasculature (Fig. 12.1), developmental abnormalities that commonly affect it, and acquired conditions that affect it, can help identify possible causes of brain injury and may allow treatment before injury to the brain becomes irreversible.
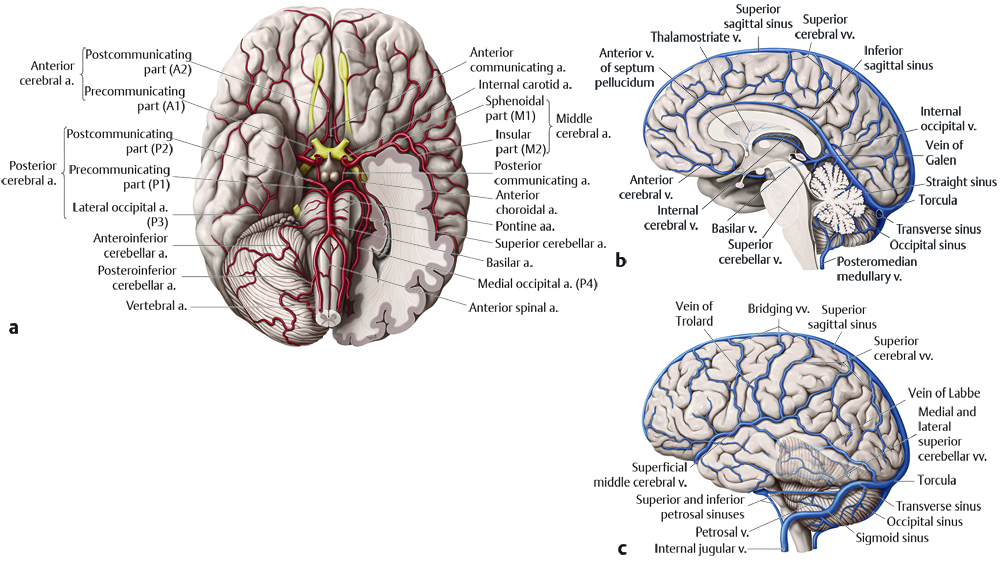
12.2 Normal Anatomy
12.2.1 Arterial
The arterial blood supply to the brain passes predominantly through four vessels: two internal carotid arteries comprising the anterior circulation of the brain and two vertebral arteries comprising the posterior circulation. The internal carotid arteries traverse the petrous temporal bone, pass through the cavernous sinus, give rise to the ophthalmic arteries, have communication with the posterior cerebral artery (PCA) through the posterior communicating arteries (PCOM), give rise to the anterior choroidal arteries, and then split into the anterior and middle cerebral arteries at the carotid terminus (Fig. 12.2). The segments of the anterior cerebral artery are referred are referred to sequentially as A1, A2, and A3. The A1 segment extends from the origin to the anterior communicating artery (ACOM). The A2 segment extends from the ACOM to the origin of the frontopolar artery, and the A3 segment extends from the frontopolar artery to the origin of the callosomarginal artery. At this last point, the anterior cerebral artery (ACA) gives off the callosomarginal and pericallosal arteries, which course respectively along the superior and inferior margins of the cingulate gyrus.
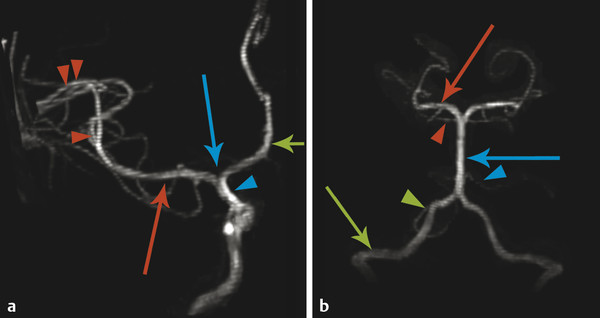
The branches of the middle cerebral artery (MCA) are likewise numbered sequentially, based largely upon the direction of each branch. The M1 segment is directed horizontally and begins at the terminus of the internal carotid artery. The M2 branches of the MCA extend superiorly along the insular surface within the sylvian fissure. The M3 branches travel horizontally to exit the sylvian fissure, and the M4 branches extend superiorly over the over the lateral surface of the parietal and posterior frontal lobes (Fig. 12.2). This naming convention originated largely through catheter angiography, and there is marked variability in the branching pattern of the MCA.
The V4 segments (the intradural segments) of the vertebral arteries give rise to the posterior inferior cerebellar arteries (PICA), which supply the inferior aspect of the cerebellar hemispheres. The two vertebral arteries combine to form the basilar artery. The mid basilar artery may give rise to anterior inferior cerebellar arteries (AICA) supplying the mid portion of the cerebellum, but this is highly variable. The distal basilar artery gives rise to the superior cerebellar arteries, supplying the superior aspect of the cerebellum. The basilar artery terminates in the prepontine cistern, dividing into two posterior cerebral arteries (PCA) that extend posteriorly around the midbrain, along the crural and ambient cisterns (Fig. 12.2). The segment of the PCA that extends from the origin of the PCA to the insertion of the PCOM is known as the P1 segment, with the next segment known as P2 as the PCA travels in the ambient cistern. The P3 and P4 segments of the PCA are more distal branches that extend through the quadrigeminal cistern and occipital region.
12.2.2 Veins
The major venous sinus in the head is the superior sagittal sinus, which is located along the calvarial margin of the falx cerebri, with blood flow extending posteriorly and eventually inferiorly toward the torcula (Fig. 12.3). In this location, blood flow through the straight sinus joins the blood flow from the superior sagittal sinus, and two laterally directed, transverse sinuses carry the blood away. The transverse sinuses extend inferiorly behind the mastoid air cells as the sigmoid sinuses, and then extend into the jugular foramina and continue caudally within the neck as the internal jugular veins. The right transverse/sigmoid sinus system is typically dominant, responsible for a larger percentage of venous drainage than the left.
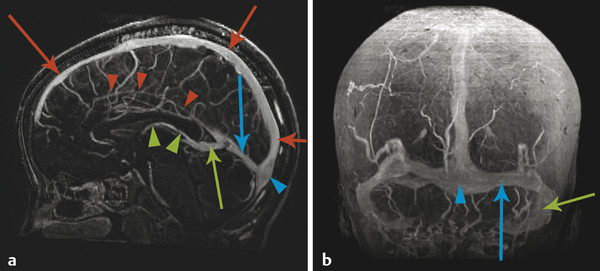
The deep gray nuclei drain into the thalamostriate veins. These join an anterior septal vein (which courses along the anterior septum pellucidum in each cerebrum) to form the internal cerebral vein. The internal cerebral veins extend posteriorly, and are joined by the basal veins of Rosenthal, which follow the course of the hippocampi. These four veins, along with a superiorly directed vermian vein, form the vein of Galen. The vein of Galen joins with the inferior sagittal sinus, and this becomes the straight sinus. As described above, the straight sinus joins with the superior sagittal sinus in the torcula, from which blood flow is directed laterally within the transverse sinuses.
The head contains two paired cavernous sinuses, which receive inflow of blood from both the superficial middle cerebral vein (sylvian vein) and the superior ophthalmic vein. The outflow of blood from the cavernous sinus on each side of the head is into the superior petrosal vein, which connects to the proximal sigmoid sinus, and the inferior petrosal vein, which joins the jugular bulb. The jugular bulb is the venous structure extending through the jugular foramen at the junction between the sigmoid sinus and the internal jugular vein.
Multiple superficial veins overlie the cerebral hemispheres, and are highly variable in their anatomy. There is typically one dominant, superiorly directed vein, known as the superior anastomotic vein (or vein of Trolard) overlying the lateral surface of both cerebral hemispheres and extending to the superior sagittal sinus, with multiple tributary veins. There is one posteriorly directed vein, known as the inferior anastomotic vein (or vein of Labbe), that connects with the distal transverse sinus bilaterally.
12.3 Imaging Techniques
12.3.1 Ultrasonography
Although ultrasonography has a limited role in evaluation of the intracranial vasculature, it does have some important applications. Doppler ultrasonography can have a role in imaging the neonatal central nervous system (CNS), such as suggesting the diagnosis of a vein of Galen aneurysmal malformation (VGAM) (Fig. 12.4). Vascular waveform analysis of the branches of the ACA in neonates may provide an indication of intracranial pressure (see Chapter 5). Transcranial Doppler ultrasonography plays an important role in the follow-up of patients with sickle-cell disease in helping to determine the timing of transfusions; increased flow velocities though the MCA have been shown to be associated with an increased risk of stroke, which decreases with transfusion.
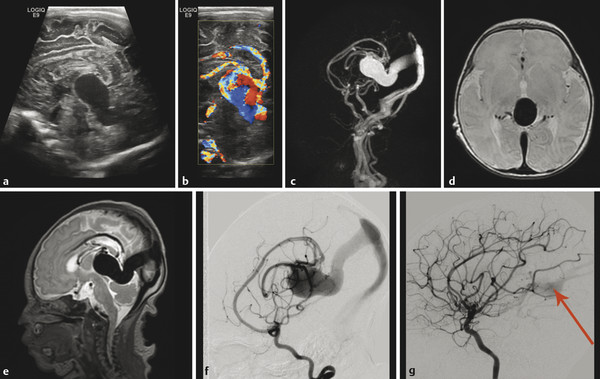
12.3.2 Computed Tomographic Angiography
Computed tomographic angiography requires an iodinated contrast material and ionizing radiation. Images made with this technique are obtained shortly after administration of the contrast material, which remains predominantly within the arterial system. This allows the evaluation of luminal abnormalities, stenosis, and occlusions. There is typically some degree of venous opacification, and allowing a slightly longer lapse after injection of the contrast material allows a more directed computed tomographic venogram. Multiplanar reformattings and three-dimensional (3D) reconstructions can be done, but 3D reconstructions in some projections may be limited by the osseous base of the skull.
12.3.3 Magnetic Resonance Angiography
Magnetic resonance angiography (MRA) is most commonly performed without intravenous contrast material, using a technique known as 3D time of flight. This provides information about flowing blood, but because the acquisition of images with the technique can take several minutes it is susceptible to artifacts caused by patient motion. Turbulent flow and high-grade stenosis can also create artifacts. Advantages of MRA include the lack of ionizing radiation and excellent depiction of vessels adjacent to, or surrounded by, bone. Contrast-enhanced MRA can be done with less artifact from turbulence or slow flow than imagbing without contrast enhancement, but it provides prominent visualization of veins, which can be both a liability and a benefit. Dynamic MRA can be performed during the administration of contrast medium, and constitutes a noninvasive, radiation-free technique that provides some of the dynamic information of conventional catheter angiography, albeit with lower spatial and temporal resolution and without the ability to use vessel-selective injections.
12.3.4 Magnetic Resonance Venography
Techniques exist for evaluating the intracranial venous structures (magnetic resonance venography). These include flow-sensitive non-contrast-enhanced techniques, such as two-dimensional (2D) time-of-flight as well as phase-contrast imaging. Both techniques are susceptible to turbulent flow and problems with stenosis, and given the slower flow in veins than in arteries, these techniques have more artifacts than MRA. When detailed venous characterization is required, postcontrast magnetic resonance venography provides additional information.
12.3.5 Digital Substraction Angiography
The traditional gold standard for evaluation of the vasculature is digital subtraction angiography (DSA), also known as catheter angiography or conventional angiography. This is an endovascular invasive technique, requires ionizing radiation, will almost always have to be done with sedation in children, and has risks of stroke and other vascular complications. However, the spatial and temporal resolution provided by DSA are unparalleled by any existing noninvasive technique, as is the ability to perform vessel-selective injections. The DSA technique is an excellent adjunct to endovascular therapy; it is typically used only for procedure planning or troubleshooting, and should not be a primary diagnostic tool.
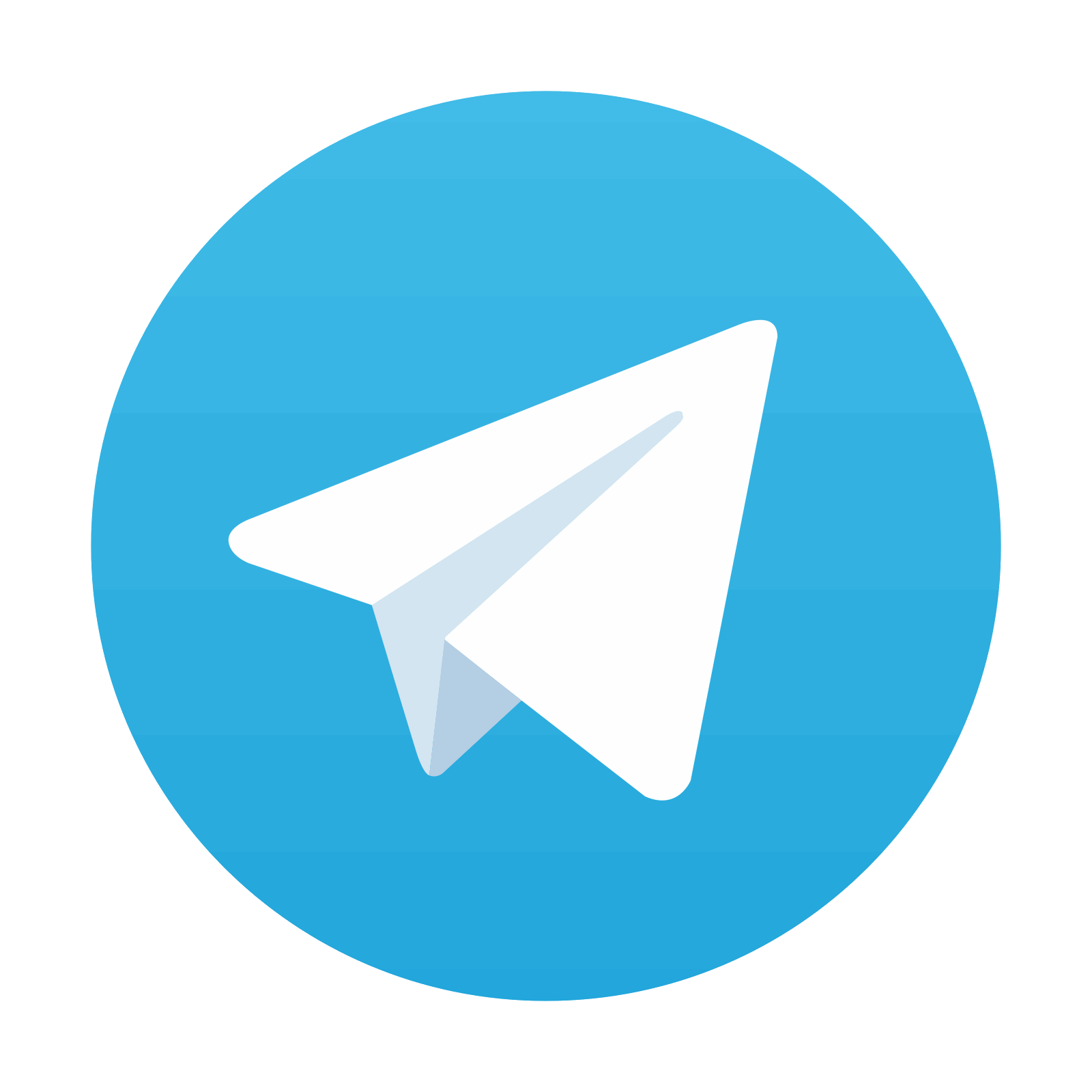
Stay updated, free articles. Join our Telegram channel

Full access? Get Clinical Tree
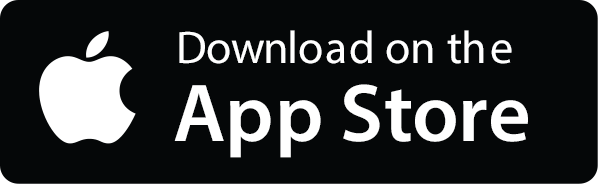
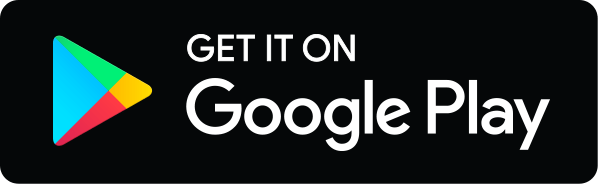
