3 Intracranial Steno-Occlusive Disease
3.1 Moyamoya Disease
3.1.1 Clinical Case
A 23-year-old female with mild right arm weakness and headache (Fig. 3.1).
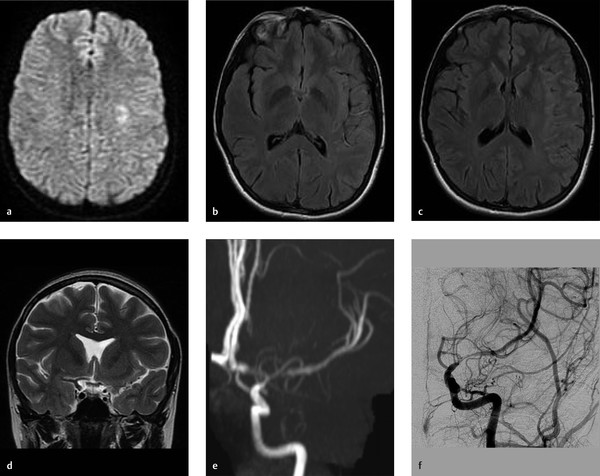
3.1.2 Description of Imaging Findings and Diagnosis
Diagnosis
Watershed infarct in the left cerebral hemisphere. High FLAIR signal in the pial arteries over the left hemisphere with sulcal hyperintensity. Absence of MCA flow void on the left. Angiogram confirms diagnosis of moyamoya.
3.1.3 Background
Moyamoya disease is an idiopathic steno-occlusive disease primarily affecting the terminus of the internal carotid artery and proximal anterior and middle cerebral arteries. The disease is histopathologically characterized by intimal hyperplasia and medial thinning. Intimal hyperplasia is caused by the proliferation of smooth muscle cells. The medial thinning is secondary to the degradation and apoptosis of smooth muscle cells and the degradation of connective tissue matrix from matrix metalloproteinase activity. Vessel occlusion occurs as a result of intimal hyperplasia and luminal thrombosis. Moyamoya collaterals are dilated perforating arteries that represent a combination of preexisting lenticulostriate perforators and newly developed vessels. Owing to increased flow, these arteries have fragmented elastic lamina, thinned media, and are prone to microaneurysm formation, which can result in hemorrhage.
Moyamoya has a bimodal age distribution with an early childhood peak and a middle age peak. Approximately, 70% of moyamoya patients present during childhood. The disease is more common in, but not exclusive to, the East Asian populations. In children, ischemic stroke is the primary presentation while in adults the primary presentation is that of intracranial hemorrhage. Moyamoya disease is idiopathic and sometimes familial. Moyamoya syndrome is secondary to conditions such as radiation induced vasculopathy, NF1, Down’s syndrome, tuberous sclerosis, and sickle-cell disease.
3.1.4 Imaging Findings
Moyamoya disease is often first identified using standard MRI protocols without the aid of vascular imaging (Fig. 3.2). On T2-weighted imaging there is often loss of the normal M1 segment flow voids, greater than the normal degree of flow voids in the basal ganglia and sulcal FLAIR hyperintensity consistent with the “ivy sign.” The areas of sulcal FLAIR hyperintensity are usually accompanied by enhancement on post-contrast imaging and are reflective of slow filling leptomeningeal collaterals that can also be seen in other condition of slow flow in the distal vasculature. Younger moyamoya patients often present with signs of cerebral ischemia including watershed infarcts and/or chronic cortical infarctions affecting the external watershed zones.
Both MRA and CTA are useful imaging modalities in evaluating the angioarchitecture of moyamoya disease. In 80% of cases, moyamoya disease is bilateral and affects the ICA termini. In 20% of cases, moyamoya disease is unilateral. In cases in which moyamoya disease is bilateral, it is possible that the disease state could be at different stages of progression on each side (Fig. 3.3). One of the key distinguishing features of moyamoya disease is the presence of robust lenticulostriate collaterals, which create a “puff of smoke” appearance. There are sometimes robust choroidal collaterals as well. It is important to point out that lenticulostriate collaterals are not always present in moyamoya, particularly in early and advanced cases. In advanced cases, there will be occlusion of the supraclinoid ICA with much of the intracranial blood supply provided by the vertebrobasilar system and external carotid arteries.
Collateral vessels should be closely inspected to evaluate the presence of microaneurysms, which can rupture and result in hemorrhage. Intraparenchymal hemorrhage in moyamoya is often the result of a ruptured lenticulostriate collateral aneurysm, whereas intraventricular hemorrhage is usually the result of a ruptured distal choroidal artery aneurysms. Hemorrhage is the most common presentation for elderly patients with moyamoya (Fig. 3.4).
There is overlap in the appearance of moyamoya with other steno-occlusive diseases such as atherosclerosis and vasculitis. High-resolution vessel wall imaging (VWI) with MRI has emerged as a useful tool to differentiate these entities. Several studies comparing the HR-VWI characteristics of moyamoya disease and intracranial atherosclerosis have demonstrated that these two diseases can in most cases be readily distinguished. Unlike atherosclerosis, moyamoya disease is often characterized by mild concentric vessel wall enhancement in the acute phase or no enhancement at all in the subacute phase. Moyamoya disease seems to lack the thicker enhancement patterns seen with vasculitis as well. Concentric wall enhancement in the distal ICA and proximal MCA is present in 90% of moyamoya disease patients, involving both symptomatic and asymptomatic segments with the degree of enhancement is generally lower than that seen with atherosclerosis. It has been suggested that the concentric enhancement may result from the intimal hyperplasia, although it is possibly also due to the associated inflammation. Moyamoya disease is typically characterized by negative remodeling (i.e., vessel wall thinning/shrinking), whereas atherosclerosis more commonly demonstrates positive remodeling (vessel wall thickening). Areas of negative remodeling and vessel obliteration demonstrate variable degrees of enhancement (Fig. 3.5).
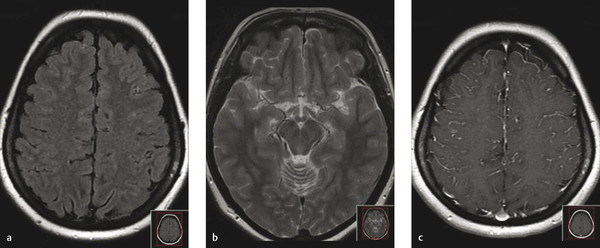
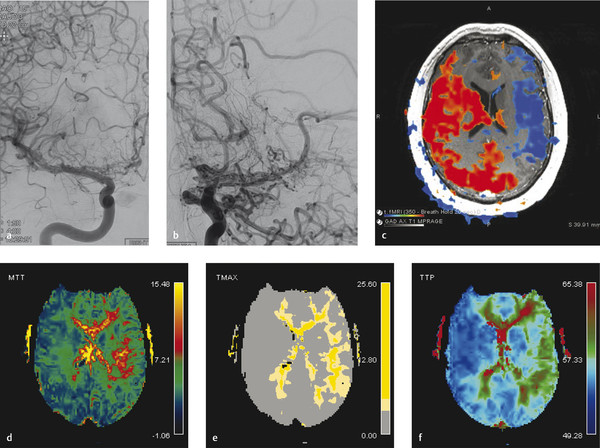
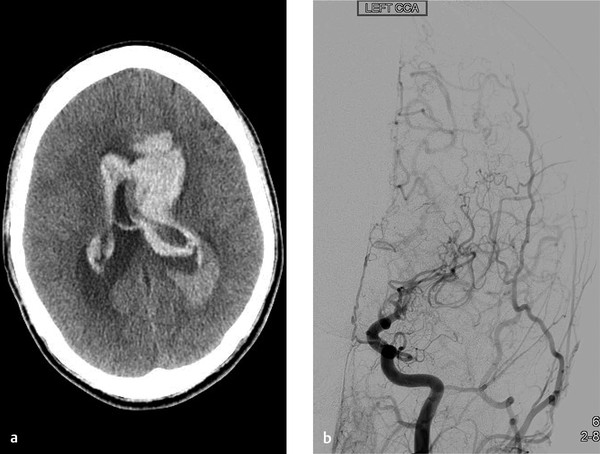
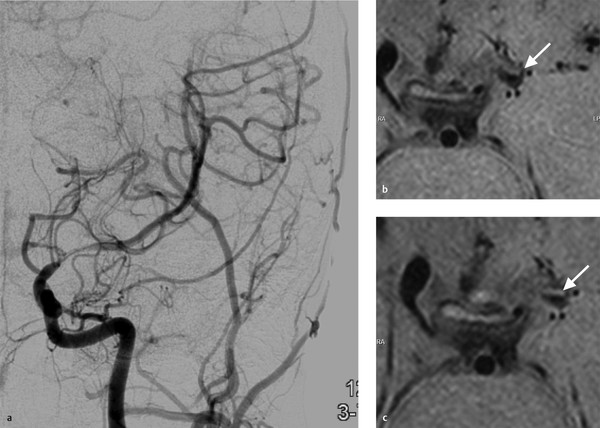
3.1.5 What the Clinician Needs to Know
General information regarding Suzuki stages of each affected vessel in moyamoya disease (Table 3.1)
Presence and location of aneurysms affecting collateral vessels
Evidence of prior ischemic injury including internal and external watershed territories
Impaired cerebrovascular reserve
Size of superficial temporal artery (STA) and occipital artery on affected sides for bypass planning
3.1.6 High-Yield Facts
Moyamoya disease in the young typically presents with ischemia, whereas moyamoya disease in the elderly presents with hemorrhage.
Aneurysms affecting the lenticulostriate and choroidal collaterals are a well-known cause of hemorrhage in moyamoya patients.
Typical MRI findings include the loss of T2 flow voids in the MCAs, robust flow voids in the basal ganglia.
Further Reading
[1] Suzuki J, Takaku A. Cerebrovascular “moyamoya” disease. Disease showing abnormal net-like vessels in base of brain. Arch Neurol. 1969; 20(3): 288–299 [2] Horie N, Morikawa M, Nozaki A, Hayashi K, Suyama K, Nagata I. “Brush Sign” on susceptibility-weighted MR imaging indicates the severity of moyamoya disease. AJNR Am J Neuroradiol. 2011; 32(9):1697–1702 [3] Brinjikji W, Mossa-Basha M, Huston J, Rabinstein AA, Lanzino G, Lehman VT. Intracranial vessel wall imaging for evaluation of steno-occlusive diseases and intracranial aneurysms. J Neuroradiol. 2017; 44(2):123–1343.2 Intracranial Atherosclerosis
3.2.1 Clinical Case
A 73-year-old male with recurrent posterior circulation ischemic stroke (Fig. 3.6).
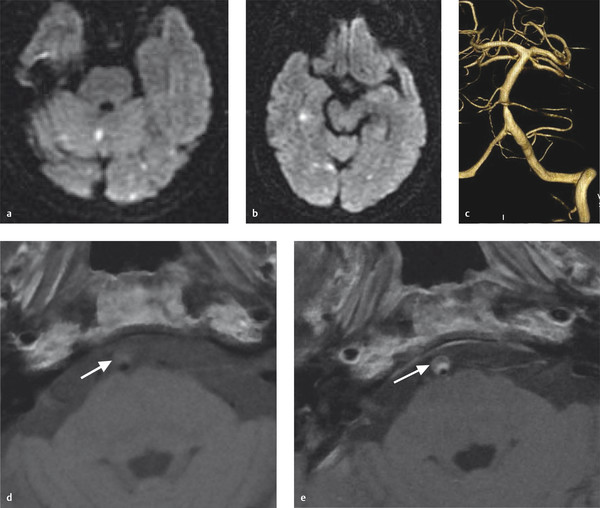
3.2.2 Description of Imaging Findings and Diagnosis
Diagnosis
Stenosis of the mid basilar artery with a vulnerable enhancing plaque. Strokes are likely secondary to embolic phenomenon related to the vulnerable plaque.
3.2.3 Background
Intracranial atherosclerosis is the most common cause of intracranial vessel narrowing in the adult population. Atherosclerosis is most commonly seen in adult patients with cardiovascular risk factors. It is more common in East Asian and African populations than in Caucasian populations and is thought to be one of the most common causes of stroke worldwide. Intracranial atherosclerosis can affect a single large vessel segment (supraclinoid ICA, M1 segment, basilar artery, etc.), be bilateral and appear similar to moyamoya or have multifocal involvement of large- and medium-sized vessels with an imaging appearance similar to CNS vasculitis.
It is important to understand the histological characteristics of intracranial atherosclerosis in order to better understand imaging appearance. Traditionally, intracranial atherosclerosis was thought to occur as a result of endothelial dysfunction resulting in the infiltration of lipids into the intima, vascular wall inflammation, and remodeling. Recent evidence suggests that the vasa vasorum and adventitia are key players in the development of intracranial atherosclerosis as the vasa vasorum are responsible for transporting inflammatory cells to the adventitia, thus initiating and perpetuating inflammatory cascades. This assertion is supported by histologic and imaging studies, which demonstrate the proliferation of vasa vasorum in patients with intracranial atherosclerotic disease. Histologic studies demonstrate that atherosclerotic plaques with intraplaque hemorrhage, lipid-rich necrotic core, and other unstable features have increased the proliferation of fragile vasa vasorum, suggesting that vasa vasorum are involved in plaque progression. Vasa vasorum proliferation plays a key role in the positive remodeling (i.e., wall thickening) seen on HR-VWI of intracranial atherosclerotic diseases.
3.2.4 Imaging Findings
Conventional luminal imaging techniques (i.e., CTA, digital subtraction angiography [DSA], and MRA) are nonspecific in the evaluation of intracranial atherosclerotic disease. The typical imaging manifestation is a focal area of narrowing involving an intracranial vessel. The most common locations include the cavernous/supraclinoid ICA, vertebral artery, and basilar artery. However, atherosclerosis can affect any intracranial vessel. When evaluating any imaging modality for intracranial atherosclerosis the three most important factors the neuroradiologist should consider are (1) the mechanism of stroke, (2) the degree of stenosis, and (3) the vulnerability and location of the plaque. Intracranial atherosclerosis can result in stroke due to (1) occlusion of perforator vessels (i.e., brainstem perforator or lenticulostriates) (Fig. 3.7), (2) emboli from a vulnerable plaque (Fig. 3.6), or (3) hemodynamic “watershed-type” infarcts due to flow limitation from high degree of stenosis (Fig. 3.8).
CTA and MRA are the two most commonly used imaging techniques for evaluating intracranial atherosclerosis. These techniques allow for the assessment of degree of stenosis and location/length of the plaque. When reviewing CTA/MRA images, careful attention should be paid to determine the relationship of the plaque/narrowing to the perforating vessels. Stenosis degree should be measured. The technique for measuring the degree of stenosis in intracranial atherosclerosis is the WASID criteria.
CTA allows for the excellent delineation of intracranial artery anatomy and is more accurate than MRA in assessing luminal stenosis. However, CTA has limitations related to bony artifacts when evaluating the cavernous ICAs. CTA, particularly multiphase CTA, is a potentially useful tool in the assessment of collateral blood flow in patients with intracranial atherosclerosis. TOF-MRA and contrast-enhanced MRA are commonly used in the evaluation of intracranial atherosclerosis. TOF-MRA generally overestimates the degree of stenosis as low-flow distal to the lesion can result in signal loss. However, there is value in seeing the loss of flow signal distal to a lesion as it highly suggests that the lesion is flow limiting. Contrast MRA is less susceptible to flow-related artifacts.
Perfusion imaging is essential to imaging intracranial atherosclerosis as hypoperfusion is a common cause of stroke in these patients. CTP and MR perfusion studies can be used to identify hypoperfused areas that could be potentially salvageable with revascularization therapies (Fig. 3.9).
High-resolution VWI has been shown to be a valuable tool in the evaluation of intracranial atherosclerosis (Fig. 3.6 , Fig. 3.7 , Fig. 3.8 , Fig. 3.9). Typically, atherosclerotic plaque appears as eccentric wall thickening and enhancement. Intrinsic high T1 signal on fat saturated T1-sequences (50% higher than temporalis muscle) represents intraplaque hemorrhage. T2-weighted HR-VWI can also be used to characterize these lesions as most lesions have a thin juxtaluminal band of T2 hyperintensity with an underlying T2 hypointense component. This thin juxtaluminal band presumably represents a fibrous cap. T2 hypointense internal areas have been shown to correlate with areas of foamy macrophages on pathologic specimens imaged at 7 Tesla with a dedicated surface coil. Positive remodeling, which occurs in a majority of cases of atherosclerosis, is essentially wall thickening due to adventitial proliferation and intraplaque hemorrhage, whereas negative remodeling is reflective of shrinkage of the vessel wall. Positive remodeling has been associated with vulnerable plaques and can be present in the absence of stenotic disease. It is important to realize that non-stenotic intracranial atherosclerosis can result in symptoms from obstruction of lenticulostriate ostia along the superior aspect of the MCA and rupture of non-stenotic plaque resulting in distal emboli.
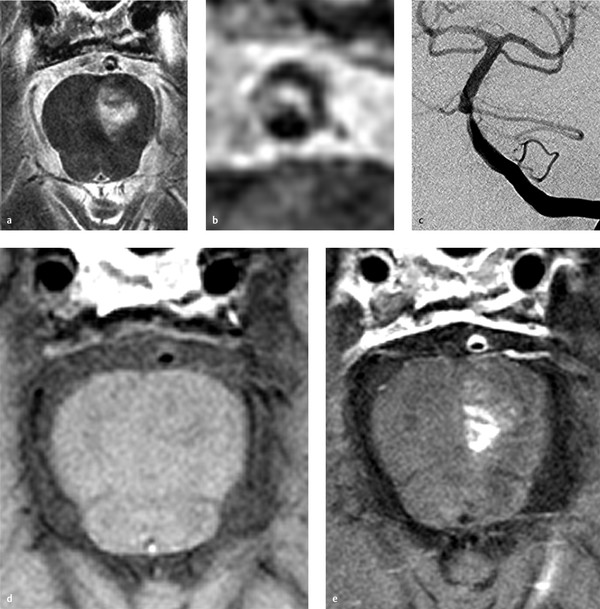
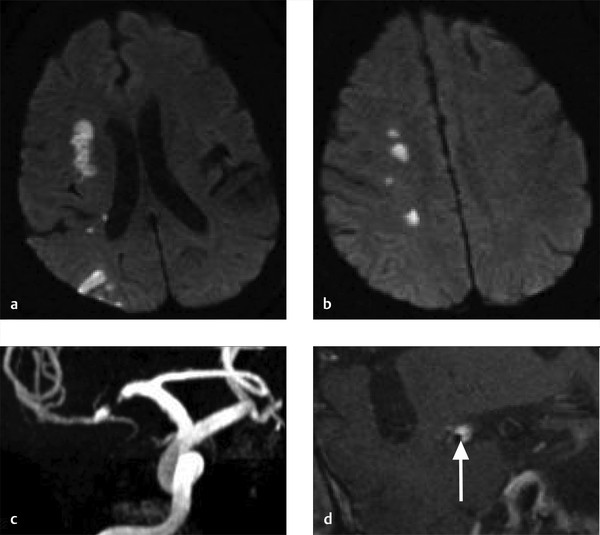
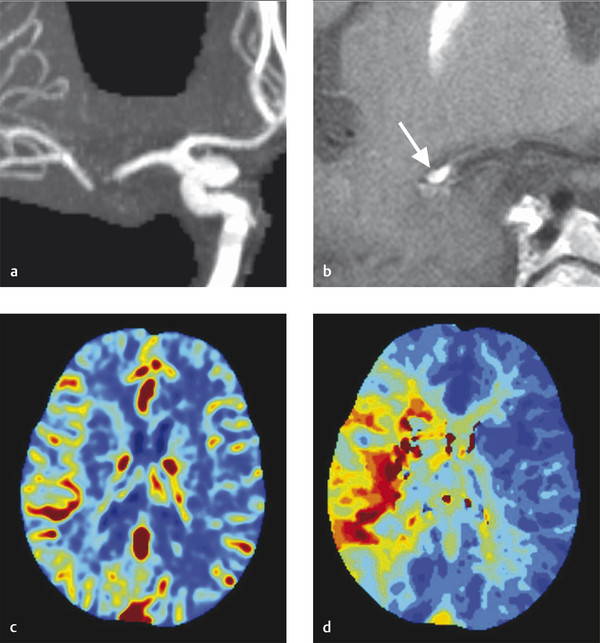
In addition to its value in the diagnosis of intracranial atherosclerosis, HR-VWI is also a valuable tool in the identification of vulnerable and culprit plaques. Studies strongly suggest that enhancement characteristics and extent of remodeling of intracranial atheromatous plaques can serve as biomarkers of plaque activity. Current evidence indicates that all symptomatic atherosclerotic plaques enhance, whereas asymptomatic plaques variably enhance. In fact, plaque enhancement is associated with a 35-times higher odds of symptomatic status. Degree of enhancement of symptomatic plaque seems to decrease over a period of weeks following an acute ischemic stroke. Enhancement characteristics can help identify culprit plaques in patients with acute ischemic stroke.
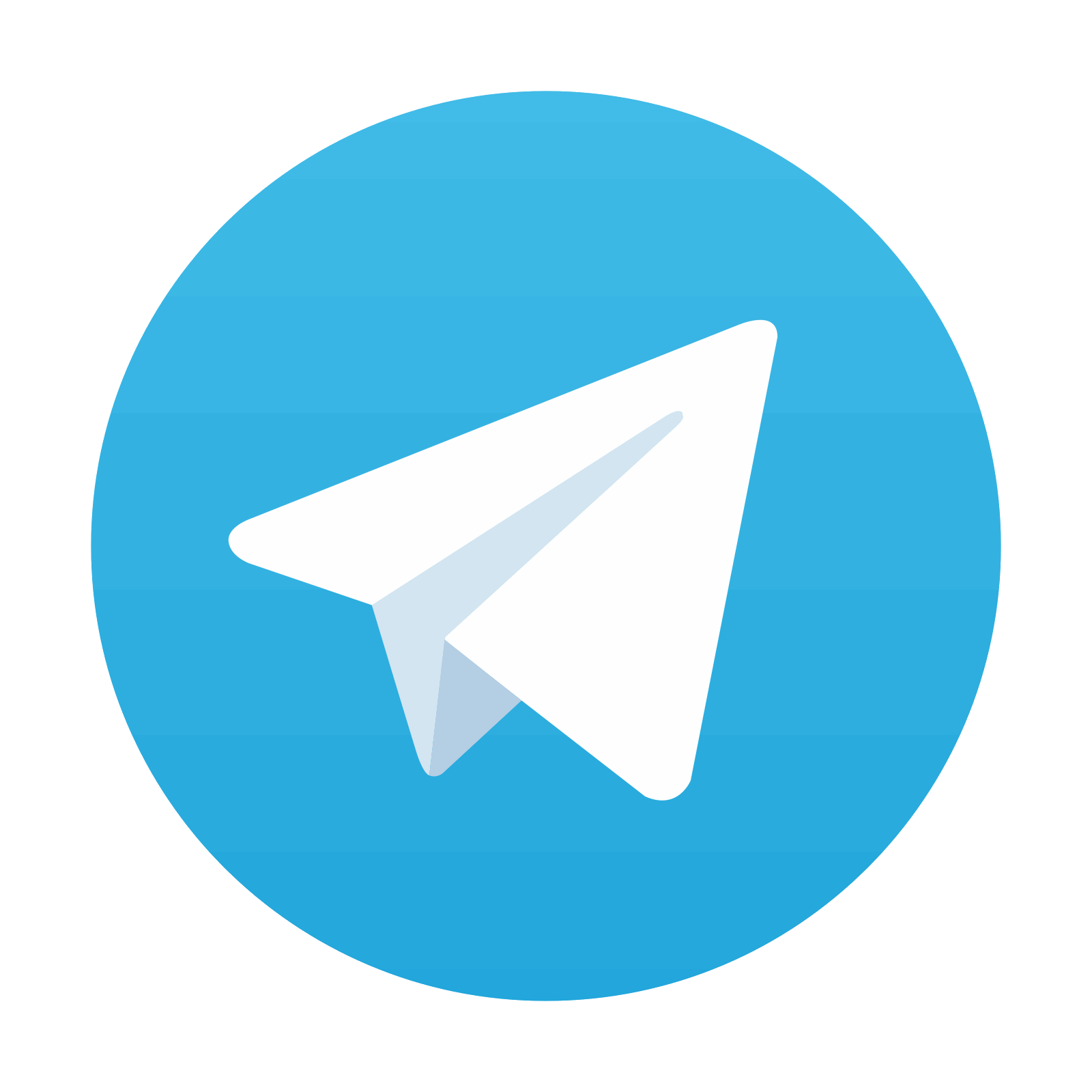
Stay updated, free articles. Join our Telegram channel

Full access? Get Clinical Tree
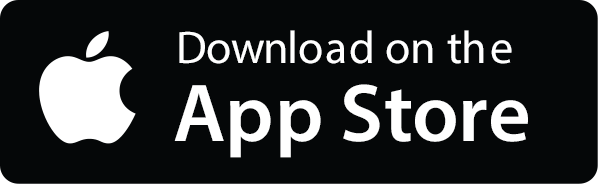
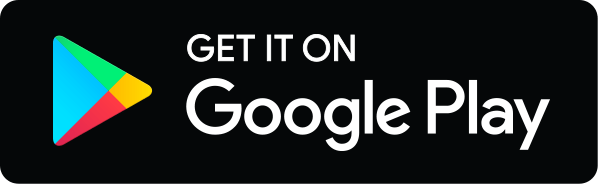
