5 Cerebral Arteriovenous Malformations (AVMs) and Dural Arteriovenous Fistulas (dAVFs)
5.1 Evaluating AVM Angioarchitecture
5.1.1 Clinical Case
A 47-year-old female with new seizures (Fig. 5.1).
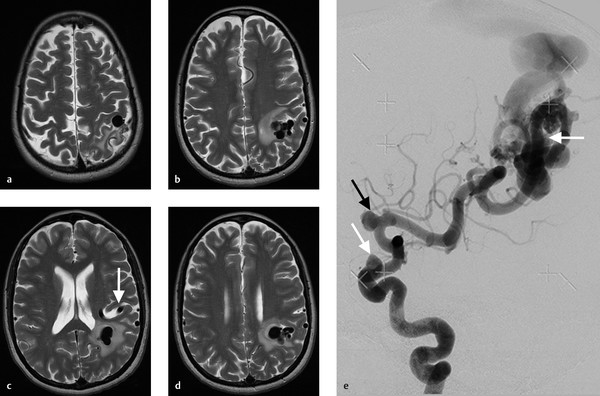
5.1.2 Description of Imaging Findings and Diagnosis
Diagnosis
Fistulous type arteriovenous malformation (AVM) in the left parietal lobe with multiple feeding arterial aneurysms, a markedly hypertrophied MCA branch suggesting a fistulous component, multiple intranidal aneurysms, large venous pouches, and a long draining vein.
5.1.3 Background
Brain AVMs are substantially rarer than intracranial aneurysms with an estimated prevalence of 20 per 100,000 people. These lesions are thought to form either in utero or early in life; however, there are a few reported cases of de novo AVMs developing in adult patients. AVMs can have a wide range of presentations including rupture, seizures, headache, cognitive decline, weakness, sensory changes, or bruit. The risk of rupture of a brain AVM is estimated to be 2–3% per year. A simple formula proposed for determining a patient’s lifetime risk of an AVM bleed is 105-(patient age in years).
There are a variety of characteristics that need to be considered when evaluating an AVM. Perhaps, the most well-known AVM grading scheme is the Spetzler-Martin Grade, which grades AVMs on a 1–5 scale based on size, eloquence, and venous drainage to determine the patient’s risk of neurological deficit after surgical resection (Table 5.1). This scale neither evaluates natural history nor does it determine risk factors or success rates of radiosurgical or embolization treatment and thus cannot be used to characterize the risk of hemorrhage or potential success and complication rates of treatments other than surgery. Through extensive study of the imaging characteristics of brain AVMs, we now have a better understanding of which angioarchitectural characteristics are important for understanding AVM natural history, pathophysiology, and treatment decisions. In this chapter, we focus on defining various angioarchitectural terms, which will be expounded on in later chapters. These terms are summarized in Table 5.1.
5.1.4 Imaging Findings
It is helpful to compartmentalize AVM angioarchitecture into three components: (1) arterial, (2) arteriovenous connection, and (3) draining veins. Each of these components have certain features which can either change natural history, affect clinical management, or influence the clinical presentation.
AVMs can have one or multiple feeding artery. Usually, the dominant feeding artery is large, whereas additional arterial feeders are smaller in caliber. Characterizing the feeding arterial branch locations, sizes, and tortuosity is important for surgical or endovascular planning purposes. Very high-flow AVMs can be associated with tortuosity of the cervical arteries as well. Occasionally, very superficial AVMs, which are large and have bled, can develop dural arterial feeders from the external carotid artery (ECA). These are difficult to identify without the help of conventional angiography. Arterial feeders should also be characterized as en-passage (i.e., multiple branch arteries supplying the nidus from a dominant arterial branch), or direct (i.e., single artery going directly into the nidus). Lastly, feeding arterial aneurysms should be identified and characterized as they are at risk of rupture due to the high-flow conditions associated with brain AVMs. Up to 20% of brain AVMs have a feeding arterial aneurysm either along the arterial pedicle just proximal to the nidus or flow-related aneurysms along branch vessels in the circle of Willis, even contralateral to the AVM. A substantial proportion of cases have multiple feeding arterial aneurysms (Fig. 5.1).
Another arterial phenomenon that provides some insight into AVM pathophysiology and symptomatic presentation is pernidal angiogenesis. In some cases, AVMs demand so much blood flow that they begin to recruit secondary feeding arteries, which go on to provide more blood flow for the dominant arterial feeder(s). This results in hypertrophy of leptomeningeal collaterals in the periphery of the AVM. On MRI, they are often dilated and tortuous vessels coursing along the sulci but lack the compact appearance of a nidus. Identifying these vessels is important because errant embolization, radiotherapy, or surgical resection of neoangiogenesis can result in infarction of normal brain (Fig. 5.2).
The arteriovenous connection is the weakest angioarchitectural point of the brain AVM. The location of the nidus in relationship to eloquent brain tissue is important. A list of eloquent regions in supplied in Table 5.2. Nidus size is important for planning surgical, endovascular, and radiosurgical interventions. It is best to measure the nidus in three dimensions to calculate a volume as certain volume thresholds indicate a poor response to radiosurgical treatment (i.e., more than 12cc). Niduses should be characterized as diffuse or compact. Most niduses are compact and do not have any intervening brain tissue. A diffuse nidus is defined as a nidus in which there is normal brain tissue interspersed between the vessels. AVMs should also be evaluated to determine if there are multiple intervening niduses (so-called multi-compartmental AVMs). Some brain AVMs can stimulate the formation of direct arteriovenous fistulas without an intervening nidus-identification of such lesions requires careful following of the course of all feeding arteries and draining veins. These fistulous components shunts can result in arterial steal phenomenon and nonhemorrhagic neurological deficits. Intranidal aneurysms should be identified and any change in size or shape of these lesions should be closely monitored. Intranidal aneurysms have been shown to portend a higher risk of rupture and are often the rupture point of hemorrhagic brain AVMs and are often located in the center of the hematoma.
The draining vein is often neglected when evaluating AVM angioarchitecture, but it often holds the key to understanding the AVM presentation and natural history. Radiologists are often inclined to describe the location and direction of the draining veins (i.e., deep and superficial), but this only helps in understanding operative risk and not clinical presentation or natural history. Venous ectasia, colloquially referred to as venous aneurysms, are associated with a higher rupture risk. These ectasias can become partially thrombosed as well resulting in mass effect. Stenosis of the draining vein is also associated with higher rupture risk as it leads to increased pressure in the nidus. Stenosis is most common at the junction between the draining vein and the dural venous sinus but can happen anywhere. Evidence of venous congestion surrounding the AVM should also be evaluated. This is best demonstrated by the presence of dilated transmedullary veins on CTA or SWI MRI. Venous congestion is often related to outflow obstruction secondary to venous stenosis or thrombosis and can result in hemorrhage or nonhemorrhagic neurological symptoms. Last, the course of the draining pial vein is important to comment on. A long pial course of the draining vein portends a higher rate of seizure and other nonhemorrhagic neurological presentations. The reason for this being the fact that a long draining vein under high pressure will not be able to drain normal parenchyma as well. In such cases, it is not uncommon to see venous congestion in the affected territory (Fig. 5.1 , Fig. 5.3).
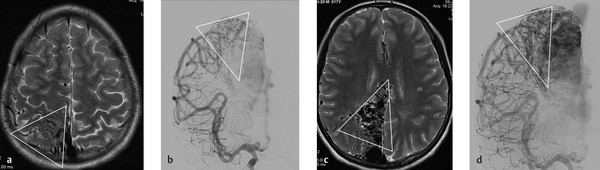
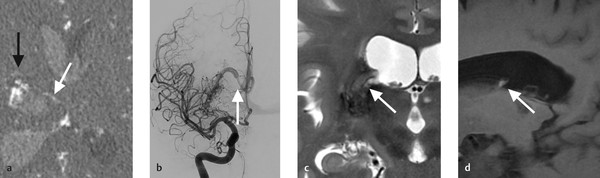
5.1.5 What the Clinician Needs to Know
Basic AVM characteristics including size, location, eloquence of surrounding brain, and venous drainage patterns
Angioarchitectural weak points of the brain AVM including intranidal aneurysms, flow-related aneurysms, venous ectasias, venous stenosis, deep venous drainage, single venous drainage and posterior fossa location
Presence of dilated cortical and transmedullary veins, which can influence the presentation of an unruptured AVM
5.1.6 High-yield Facts
AVM characteristics associated with the higher risk of future hemorrhage include prior hemorrhage, intranidal aneurysm, venous stenosis or ectasia, deep venous drainage, single venous drainage, and deep or posterior fossa location.
AVM characteristics associated with a higher risk of nonhemorrhagic neurologic deficits include a high-flow shunt, venous congestion or outflow obstruction, long pial course of the draining vein, and arterial steal.
Spetzler-Martin score is associated with surgical risk and not natural history.
Further Reading
[1] Geibprasert S, Pongpech S, Jiarakongmun P, Shroff MM, Armstrong DC, Krings T. Radiologic assessment of brain arteriovenous malformations: what clinicians need to know. Radiographics. 2010; 30(2):483–501 [2] Mokin M, Dumont TM, Levy EI. Novel multimodality imaging techniques for diagnosis and evaluation of arteriovenous malformations. Neurol Clin. 2014; 32(1):225–236 [3] Leclerc X, Gauvrit JY, Trystram D, Reyns N, Pruvo JP, Meder JF. [Cerebral arteriovenous malformations: value of the non invasive vascular imaging techniques]. J Neuroradiol. 2004; 31(5):349–3585.2 Evaluating the Ruptured Brain AVM
5.2.1 Clinical Case
A 47-year-old female with sudden onset severe headache and the loss of consciousness.
5.2.2 Description of Imaging Findings and Diagnosis
Diagnosis
Ruptured vermian AVM with intraparenchymal and intraventricular hemorrhage with an intranidal aneurysm, which is projecting directly into the large hematoma (Fig. 5.4).
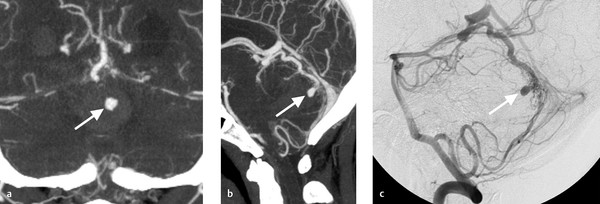
5.2.3 Background
Ruptured brain AVMs present a major clinical challenge for surgical and endovascular neurovascular specialists. One of the key challenges in managing these lesions is deciding when and how to treat the lesion. Unlike ruptured intracranial aneurysms, current recommendations state that it is preferable to wait at least a month before attempting to cure a patient of a ruptured AVM. The reasons for this are two-fold. First, many studies suggest that patients who are rehabilitated and then undergo AVM resection may experience better outcomes than those who are treated in the acute phase. Second, in some cases, the hematoma from the AVM rupture can compress part of the nidus or one of the draining veins making part of the AVM invisible on imaging. Tackling the lesion while there is a hematoma could lead to incomplete treatment. There is no ambiguity when it comes to emergency decompressive surgery or hematoma evacuation for patients who have large hematomas causing mass effect. However, even in these cases, resection of the AVM is not recommended.
There is a growing body of literature suggesting that targeted treatment of intranidal and feeding arterial aneurysms in ruptured brain AVMs in the acute phase may be of benefit. In patients with an AVM-associated aneurysm and a ruptured brain AVM, the rate of rehemorrhage is 11% per patient-month, whereas it is just 1% per patient-month without an aneurysm. Because of this, it is imperative for neuroradiologists to identify any and all angiographic weak points within a ruptured AVM. The neuroradiologists should ask herself “what part of the AVM ruptured?” whenever encountered with a ruptured AVM. Among ruptured AVMs, associated aneurysms are the cause of hemorrhage in roughly 50% of cases.
5.2.4 Imaging Findings
Again, when assessing angiographic weak points in an already ruptured brain AVM, it helps to compartmentalize AVM angioarchitecture into three components: (1) feeding arteries, (2) arteriovenous connection/nidus, and (3) draining veins. Each of these components have certain features, which can affect the clinical management of patients with ruptured AVMs.
Feeding arterial aneurysms are present in roughly 20% of ruptured brain AVMs. The pathophysiological basis for these lesions is thought to be an underlying vascular defect or a result of the dynamic interaction between hemodynamic stress, vasoactive substances, and functional alterations from vascular remodeling. There are three types of flow-related aneurysms, each with different levels of severity. First, unrelated aneurysms are aneurysms which form in the intracranial circulation but are remote from the AVM itself. These lesions can rupture just like any other intracranial aneurysm but the site of the rupture is remote from the AVM itself. An example of an unrelated aneurysm would be a left MCA aneurysms in a patient with an AVM in the right frontal lobe. Regarding flow-related aneurysms, we have proximal and distal flow-related aneurysms. Proximal flow-related aneurysms are located on a vessel that is proximal to the artery, which is directly feeding the AVM. These aneurysms are located on the ICA, circle of Willis, MCA (M1 segment or bifurcation) or vertebrobasilar trunk. An example of a proximal flow-related aneurysm would be a left M1 segment aneurysm in a patient with a left sylvian fissure AVM supplied by M2/M3 branches. Distal flow-related aneurysms are located along the artery, which is directly supplying the AVM (Fig. 5.5). There is a direct relationship between the proximity of the aneurysm to the AVM and the risk of rupture.
Intranidal aneurysms are present in up to 50% of ruptured AVMs. These lesions form as weak points within an already weak nidus and, when present, are often the culprit for the hemorrhage. Oftentimes, the intranidal aneurysm will project directly into the hematoma cavity, and when that happens, one can be certain that the aneurysm itself was the culprit for the hemorrhage. Conveying this information is important because targeted endovascular or surgical treatment can be performed to exclude the intranidal aneurysm while leaving the rest of the AVM intact.
Bleeding can result from draining vein pathology as well. Venous ectasias and varices, colloquially referred to as venous aneurysms can rupture. These hemorrhages can vary from large intraparenchymal hematomas or local hemorrhage solely surrounding the venous varix (Fig. 5.6). Ruptured venous varices are often associated with local thrombosis as well. Targetting treatment to these lesions is difficult without targeting the AVM in its entirety, but the presence of a ruptured venous varix spells a poorer natural history for the already ruptured AVM and may prompt earlier treatment. AVM rupture can also result from draining vein stenosis or occlusion/thrombosis. Draining vein stenosis or thrombosis can cause rupture due to the associated increased in intranidal blood pressure. Stenosis of the draining vein is also associated with higher rates of re-rupture as well and may prompt earlier treatment (Fig. 5.7). Occasionally, draining vein thrombosis/stenosis can result in rupture and subsequent obliteration of the AVM. Stenosis is most common at the junction between the draining vein and the dural venous sinus but can happen anywhere.
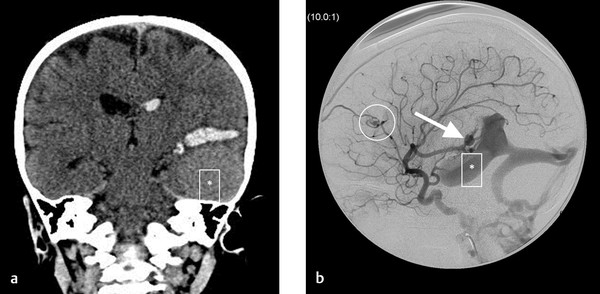
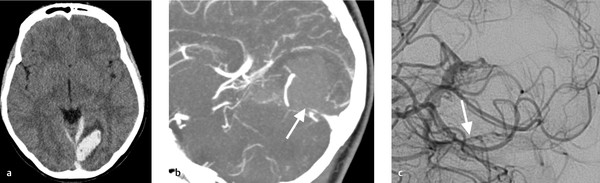
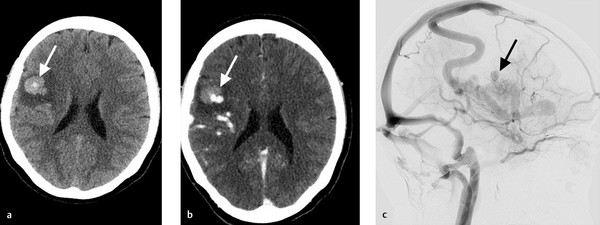
A point should be made about negative CTA/MRA/DSA in a patient with a suspected AVM. A small proportion of brain AVMs are micro-AVMs, defined as lesions which measure 1 cm or less in size. When these lesions rupture they are often completely compressed by the surrounding hematoma and may be occult on conventional angiography and cross-sectional imaging. In cases where there is no clear etiology for the hemorrhage or when an AVM is suspected (i.e., younger patient with occipital hematoma), repeat imaging should be performed around 3 months post-hemorrhage. Such imaging should include DSA and CTA/MRA. If these imaging tests are negative then a repeat CTA/MRA at around 6–12 months is reasonable.
5.2.5 What the Clinician Needs to Know
Presence of lesions which can be targeted for partial embolization or surgical treatment such as feeding arterial or intranidal aneurysms.
Venous pathologies which may have resulted in the AVM hemorrhage including venous aneurysms, venous stenosis, or venous occlusion.
CTA and MRA are not sensitive for detection of smaller AVMs in the setting of a rupture and repeat imaging around three months post-hemorrhage should be considered.
5.2.6 High-yield Facts
Aneurysms associated with AVMs can be (1) unrelated to the AVM itself (i.e., contralateral), (2) proximal feeding arterial (i.e., ICA, basilar artery, vertebral artery, M1, CoW), (3) distal (i.e., on the direct arterial feeder), or (4) intranidal.
Venous aneurysms can rupture and often produce a small hematoma surrounding the venous aneurysm itself.
Draining vein stenosis/occlusion can result in higher rates of re-rupture of the AVM.
Further Reading
[1] Geibprasert S, Pongpech S, Jiarakongmun P, Shroff MM, Armstrong DC, Krings T. Radiologic assessment of brain arteriovenous malformations: what clinicians need to know. Radiographics. 2010; 30(2):483–501 [2] Mokin M, Dumont TM, Levy EI. Novel multimodality imaging techniques for diagnosis and evaluation of arteriovenous malformations. Neurol Clin. 2014; 32(1):225–236 [3] Leclerc X, Gauvrit JY, Trystram D, Reyns N, Pruvo JP, Meder JF. [Cerebral arteriovenous malformations: value of the non invasive vascular imaging techniques]. J Neuroradiol. 2004; 31(5):349–3585.3 Evaluating Unruptured AVMs and the Peri-AVM Environment
5.3.1 Clinical Case
A 47-year-old female with sudden onset severe headache and visual aura (Fig. 5.8).
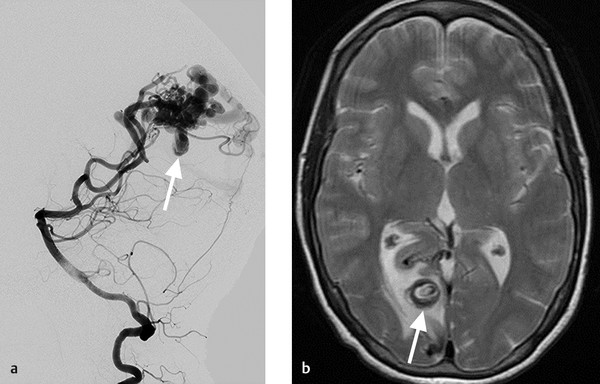
5.3.2 Description of Imaging Findings and Diagnosis
Diagnosis
Unruptured brain AVM in the right occipital lobe. The AVM has a large inferiorly projecting venous pouch. On T2 MRI, the pouch is partially thrombosed and there is severe associated surrounding vasogenic edema.
5.3.3 Background
Unruptured brain AVMs currently present a major management conundrum for neurovascular specialists. Following publication of the ARUBA trial that showed superior outcomes for conservative management over curative therapy for unruptured brain AVMs, there has been some pushback against treatment of these lesions. However, unruptured brain AVMs can result in substantial morbidity and mortality due to their risk of rupture as well as their proclivity to cause neurological symptoms in the absence of rupture.
When evaluating an unruptured AVM, it is important to characterize the lesion using the angioarchitectural features described in the prior sections 5.1 and 5.2. This is especially important for evaluating risk of rupture. However, it is also important to consider how the lesion is interacting and affecting the adjacent brain parenchyma. Aside from hemorrhage, unruptured high-flow shunting AVMs can impact the adjacent brain parenchyma in a variety of ways including hypoxemia, mass effect, venous congestion, and vasogenic edema. These mechanisms can result in focal neurological deficits, seizures, developmental delay, cognitive decline, headaches, and hydrocephalus. Here, we will describe how one should characterize both the unruptured AVM and peri-AVM environment to better understand the clinical presentation and natural history of an unruptured brain AVM.
5.3.4 Imaging Findings
When determining the rupture risk of a brain AVM, angioarchitecture is key. There are a few salient angioarchitectural findings, which are associated with increased risk of rupture of a previously unruptured brain AVM and they include feeding arterial aneurysms, intranidal aneurysms, venous ectasias, and venous outflow stenoses. The prior two chapters provide representative images for these features.
MRI is the preferred modality for evaluation of the peri-AVM environment. Salient findings in the peri-AVM environment that should be evaluated on MRI include medullary venous congestion, prior AVM bleeding, signs of perilesional arterial steal, presence of a long draining vein (i.e., 3 cm or longer), and AVM-related edema (Table 5.3).
As described in the sections 5.1 and 5.2, venous drainage patterns have a substantial effect on the natural history and clinical presentation of brain AVMs. When evaluating the draining vein of a brain AVM, it is important to try to think about how arterialization of the draining vein will affect the normal brain, which was originally draining through this vein (Fig. 5.9). This is especially true in deep brain AVMs that drain into internal cerebral veins or the galenic system as the venous drainage of the deep structures is also dependent on these veins to drain. Venous arterialization can impair the normal physiological gradients of medullary venous drainage and result in medullary venous congestion. On imaging, this will manifest as deep white matter edema with or without dilated transmedullary veins. In extreme cases, medullary venous congestion can result in hydrocephalus from impaired CSF clearance and cognitive dysfunction (Fig. 5.10). Perfusion imaging in these patients will show increased CBV (from venous congestion) and MTT (from delayed transit through the medullary venous system). High flow in the draining vein can also result in a venopathy and eventual stenosis or thrombosis of the draining vein. If this involves a vein which is also draining normal vein, the patient can suffer a venous infarct in the venous drainage territory (Fig. 5.11).
The presence of a large venous pouch does not only portend a higher risk of rupture, but also can result in mass effect and associated vasogenic edema (Fig. 5.8). Mass effect and edema from a venous pouch can be exacerbated in the setting of acute thrombosis of the pouch itself. Attention should be paid on conventional T1 and T2-weighted imaging for the presence of thrombus or enhancing thrombus in a venous pouch and its associated mass effect. Mass effect can result in focal neurological deficit, seizure activity, or hydrocephalus, if it is in the region of the cerebral aqueduct or other CSF drainage pathways.
Perfusion imaging can provide valuable insights into how an AVM is affecting blood flow in the extranidal brain parenchyma (Table 5.4). One phenomenon that is commonly discussed in the context of brain AVMs is that of “arterial steal.” Arterial steal is a process in which increased blood flow through a low-resistance system (i.e., brain AVM) diverts blood flow from a region of normal brain. This results in relative hypotension in these regions and hypoxemia. Studies of CT perfusion in brain AVMs have shown three types of extranidal brain parenchymal perfusion patterns: (1) functional arterial steal, (2) ischemic arterial steal, and (3) venous congestion. In the functional arterial steal pattern, there is decreased CBF, CBV, and MTT (Fig. 5.12). The decreased MTT in these patients reflects a sumping effect from the AVM. In an ischemic arterial steal setting, there is increased MTT and decreased CBF and CBV, which reflects arterial steal from an indirect collateral connection or in an area remote from the nidus in which blood flow is rerouting from normal brain toward the AVM. These perfusion patterns are important as they are associated with AVM-related symptoms. Patients with functional arterial steal most commonly present with seizure while those with ischemic arterial steal most commonly present with focal neurological deficit. Chronic arterial steal of any type can result in local encephalomalacia and gliosis on MRI as well as perinidal angiogenesis and an increased prominence of arterial flow voids surrounding the nidus.
T2* imaging is an essential component to brain AVM imaging. Many patients with “unruptured” brain AVMs have had silent bleeding from a brain AVM, which is best demonstrated on GRE or SWI imaging and hemosiderin staining surrounding the brain AVM itself (Fig. 5.13). This is thought to be associated with a higher rate of future rupture.
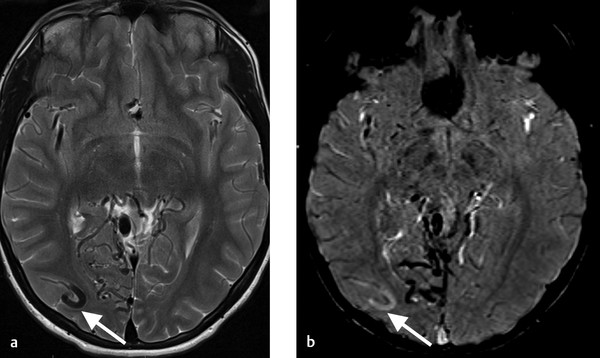
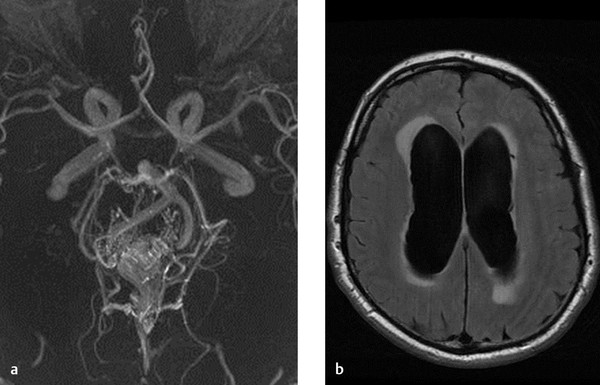
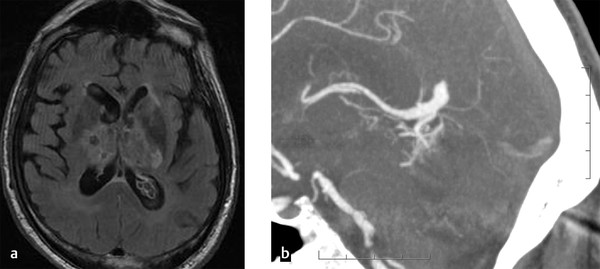
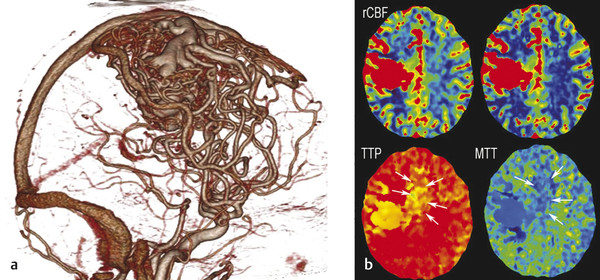
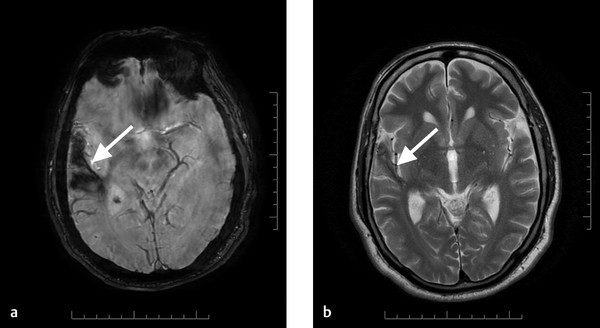
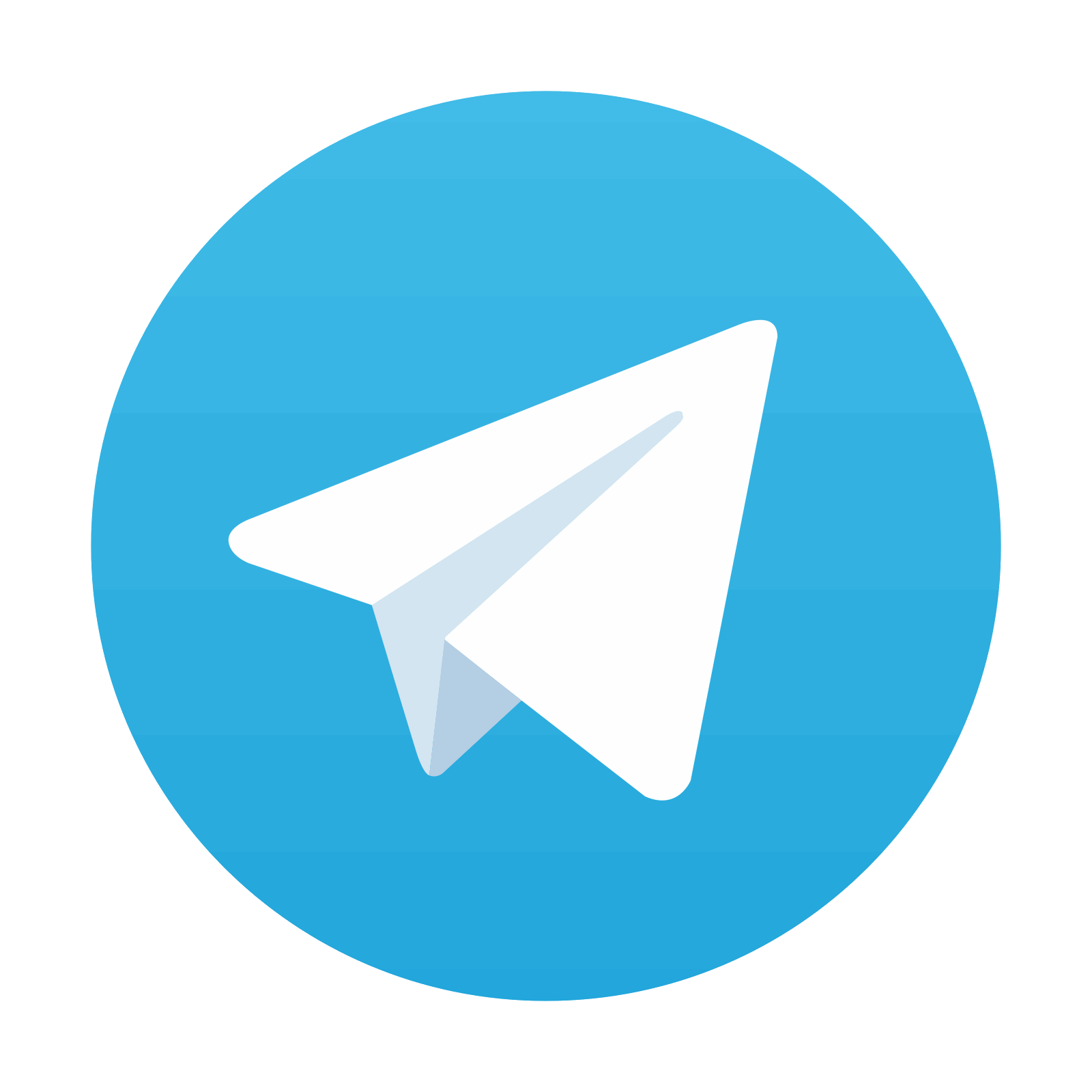
Stay updated, free articles. Join our Telegram channel

Full access? Get Clinical Tree
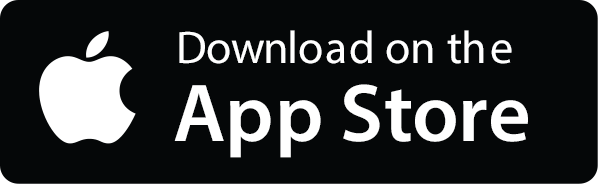
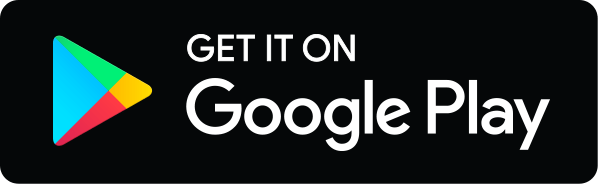
