6 Pediatric Neurovascular Disease
6.1 Pediatric Aneurysms
6.1.1 Clinical Case
A 6-month-old boy, obtunded, with right-sided weakness (Fig. 6.1).
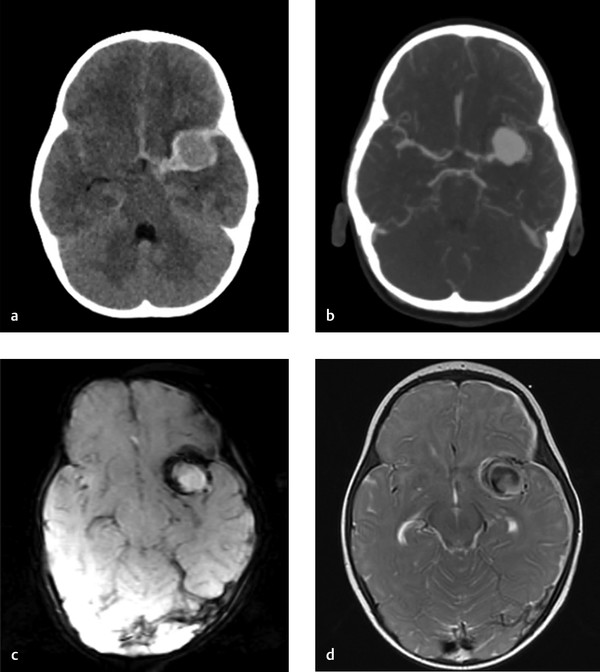
6.1.2 Description of Imaging Findings and Diagnosis
Diagnosis.
Giant “partially thrombosed” left MCA aneurysm.
6.1.3 Background
Pediatric intracranial aneurysms are exceedingly rare, comprising just 0.6% of all cerebral aneurysms. The pathogenesis and pathophysiology of pediatric cerebral aneurysms differ substantially from that of adult cerebral aneurysms. These lesions are typically the result of a dissecting disease that can be related to vasculopathy or connective tissue diseases. Other causes include infection, immunodeficiency, or trauma. In fact, previous (even minor) trauma has been identified as a major predisposing factor for pediatric cerebral aneurysms as up to 40% of all aneurysms are believed to be related to trauma. Unlike in adults, pediatric intracranial aneurysms are more commonly found in males than females. “Classical” saccular aneurysms are relatively less common in the pediatric age group as compared to adults.
Given their different etiology as compared to the adult population, the clinical presentation of these lesions is also different from that of adults. Pediatric cerebral aneurysms can present with compressive effects, focal ischemic deficits, intraparenchymal hemorrhage, seizures, or subarachnoid hemorrhage (SAH). Over 60% of pediatric patients with SAH have a cerebral aneurysm.
6.1.4 Imaging Findings
In a child with suspected SAH, the first diagnostic imaging modality of choice is non-contrast CT. If SAH is identified, MR angiography may be preferred over CT angiography due to the added radiation dose of CTA. However, at many institutions, the presence of SAH on CT prompts multimodality imaging including MR/MRA, CTA, and a diagnostic cerebral angiogram due to the relatively high rate of false-negatives on conventional cross-sectional imaging and the potentially life-threatening consequences of an undiagnosed ruptured aneurysm.
Important factors that must be distinguished on luminal imaging include aneurysm size, location, and morphology that may give clues on underlying etiology. Unlike in the adult population, giant aneurysms are relatively common in the pediatric population with up to 40% of aneurysms in some series. Regarding location, approximately 5–10% of aneurysms are located at the anterior cerebral artery (ACA)/Acom, 25% at the ICA terminus, and about 25% are located in the posterior circulation. This distribution of locations differs substantially from the adult population where 30% of aneurysms are located at the ACA/Acom, 5% are located at the ICA terminus, and 10% located in the posterior circulation. Similar to the adult population, about 20% of aneurysms are located along the MCA.
Larger case series classify aneurysm etiology into four categories: saccular, infectious, dissecting, and traumatic. Understanding aneurysm etiology is important because it dictates both treatment decisions and clinical presentation. The most common type of aneurysm is the “classical” saccular aneurysm, although its proportion (approximately 40–50% of all pediatric aneurysms) is lower as compared to the adult population. These aneurysms can be large and may have intraluminal thrombus within them. Saccular aneurysms are the most likely to rupture into the subarachnoid space. Dissecting or “fusiform” aneurysms comprise 30–40% of all pediatric aneurysms and are particularly common in patients with connective tissue diseases (Fig. 6.2). The number of layers involved in the dissection and perforator involvement by the intramural hematoma will determine their clinical presentation. Dissecting fusiform aneurysms can present with infarction via either reopening of the dissection into the parent artery, perforator occlusion through intramural hematoma progression, or complete vessel occlusion. In rare cases, repeated dissections can present with “growing” partially thrombosed aneurysms. Transmural dissecting aneurysms present with hemorrhage. Infectious aneurysms can be distal “mycotic” aneurysms, typical of those seen in patients with endocarditis, or proximal fusiform aneurysms related to viral infections or immunodeficiencies (HIV, familial candidiasis). These aneurysms can demonstrate mural enhancement secondary to the inflammatory process that is responsible for their pathogenesis and often present with infarction (Fig. 6.3). Traumatic aneurysms are generally located at the skull base or the periphery and associated with adjacent fractures.
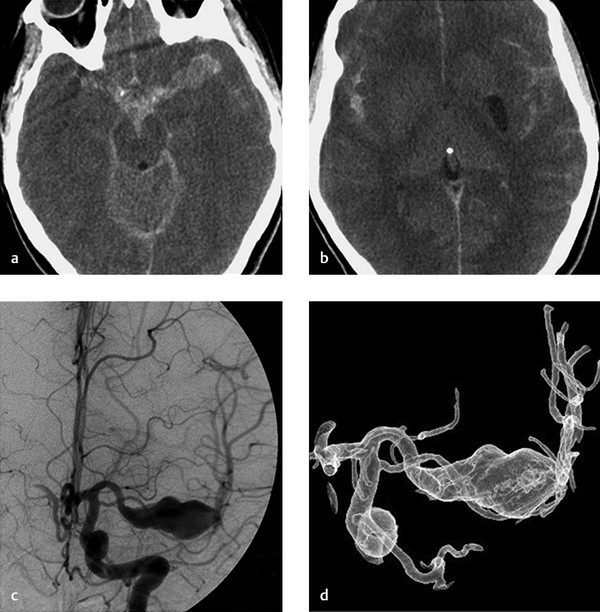
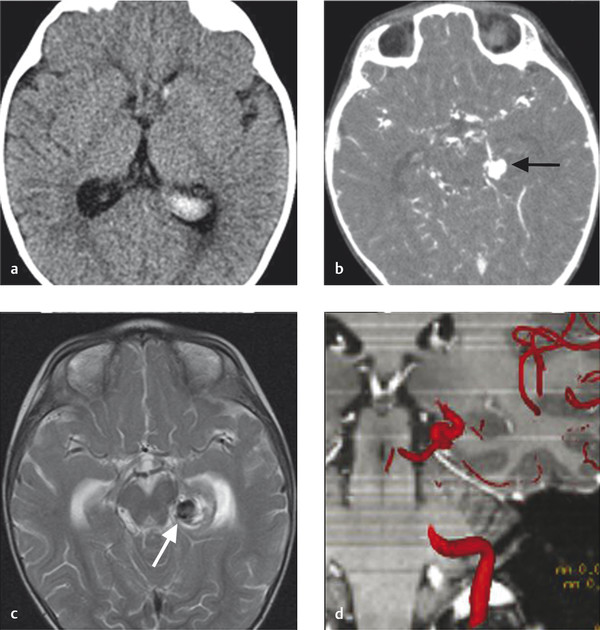
There are a number of important factors the radiologist must consider when interpreting a scan in a pediatric patient with a cerebral aneurysm. Close attention to the peri-aneurysmal environment focusing on perianeurysmal edema, wall enhancement/inflammation, mass effect, and infarction in the aneurysm vascular territory is essential. Attention to the remainder of the cerebral vasculature evaluating for the presence of additional aneurysms is important as about 15% of pediatric patients have multiple aneurysms. Intracranial stenosis, particularly of the ICA terminus, should also be ruled out as aneurysms can form secondary to occlusive arteriopathies such as moyamoya disease, sickle cell, or ACTA1 mutations. Cervical arterial tortuosity should be commented on as it could suggest an underlying connective tissue disease (Marfan’s syndrome, Loeys-Dietz syndrome) and has implications for endovascular management.
6.1.5 What the Clinician Needs to Know
Aneurysm size and location
Aneurysm etiology (i.e., saccular, dissecting, traumatic, or infectious)
Presence of intra-aneurysmal or intramural thrombus
Presence of aneurysm-associated cerebral edema, mass effect, or infarction
Tortuosity of cervical vasculature affecting endovascular access
Aneurysm multiplicity
Presence of vascular abnormalities (stenoses and tortuosity) elsewhere suggesting underlying moyamoya, sickle cell, or other occlusive arteriopathy
6.1.6 High-yield Facts
Etiology, as well as morphology, of pediatric aneurysms differs substantially from adult population; more likely to be giant, non-saccular, and located in posterior circulation.
Pediatric cerebral aneurysms often secondary to connective tissue diseases, infection, or trauma; important to evaluate for additional imaging findings suggestive of an underlying disease process.
Fusiform and infectious aneurysms are more commonly associated with infarction, whereas saccular are more commonly associated with SAH.
In pediatric SAH, it is reasonable to consider diagnostic cerebral angiography in lieu of CT angiography.
Further Reading
[1] Gemmete JJ, Toma AK, Davagnanam I, Robertson F, Brew S. Pediatric cerebral aneurysms. Neuroimaging Clin N Am. 2013; 23(4):771–779 [2] Jian BJ, Hetts SW, Lawton MT, Gupta N. Pediatric intracranial aneurysms. Neurosurg Clin N Am. 2010; 21(3):491–501 [3] Kanaan I, Lasjaunias P, Coates R. The spectrum of intracranial aneurysms in pediatrics. Minim Invasive Neurosurg. 1995; 38(1):1–9 [4] Krings T, Choi IS. The many faces of intracranial arterial dissections. Interv Neuroradiol. 2010; 16(2):151–160 [5] Chen L, Yau I, deVeber G, Dirks P, Armstrong D, Krings T. Evolution of a chronic dissecting aneurysm on magnetic resonance imaging in a pediatric patient. J Neurosurg Pediatr. 2015; 15(2):192–196 [6] Krings T, Geibprasert S, terBrugge KG. Pathomechanisms and treatment of pediatric aneurysms. Childs Nerv Syst. 2010; 26(10):1309–13186.2 Pediatric Dural Arteriovenous Fistulae
6.2.1 Clinical Case
A 6-year-old boy. Enlarging head circumference since infancy (Fig. 6.4).
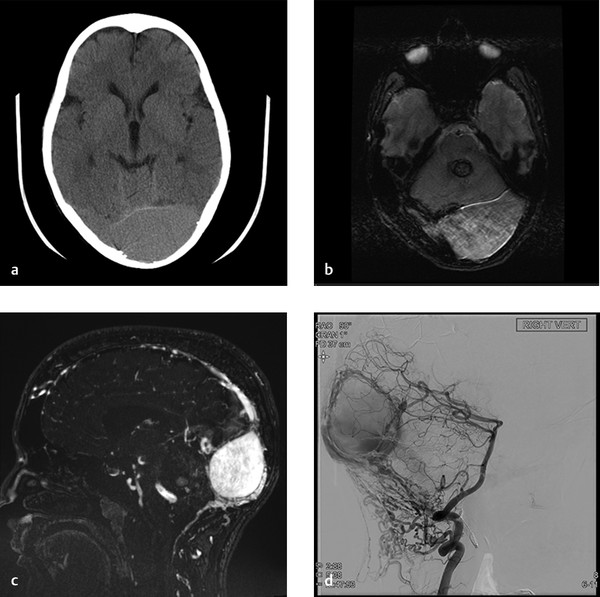
6.2.2 Description of Imaging Findings and Diagnosis
Diagnosis.
Torcular dural sinus malformation.
6.2.3 Background
Pediatric dural arteriovenous shunts are a rare form of vascular disease in the pediatric population. With less than 200 cases reported in the literature, little is known regarding their natural history. The most widely accepted classification of pediatric dural arteriovenous shunts is that of Lasjuanias et al., which includes three types: (1) dural sinus malformation, (2) infantile dural arteriovenous shunt, and (3) adult type dural arteriovenous shunt. Common clinical manifestations of these lesions include seizure, macrocrania, hydrocephalus, and hemorrhage.
Regarding the classification of these lesions, dural sinus malformations often involve the torcula but can be found anywhere along the dural sinuses and are characterized by giant dural lakes and slow-flow mural arteriovenous shunting that derives its arterial supply from the meningeal branches. These lesions can involve other sinuses or the jugular bulb in which case they are associated with high-flow arteriovenous shunts. Infantile dural arteriovenous shunts are high-flow low-pressure lesions draining into a patent sinus and are not associated with a malformation of the sinus. Adult-type dural arteriovenous shunts develop following thrombosis in the sinus wall or trauma to the sinus.
Little is known regarding the pathogenesis of these lesions. Most of these lesions are considered to be congenital (Fig. 6.5). Dural sinus malformations, as in the case presented here, are thought to result from persistent ballooning of the torcula in utero. This results in sinus wall overgrowth and development of abnormal venous spaces/lakes, which may predispose to the development of arteriovenous shunts. Unlike in the adult population, cerebral sinus thrombosis is considered a rare cause of pediatric dural arteriovenous shunts.
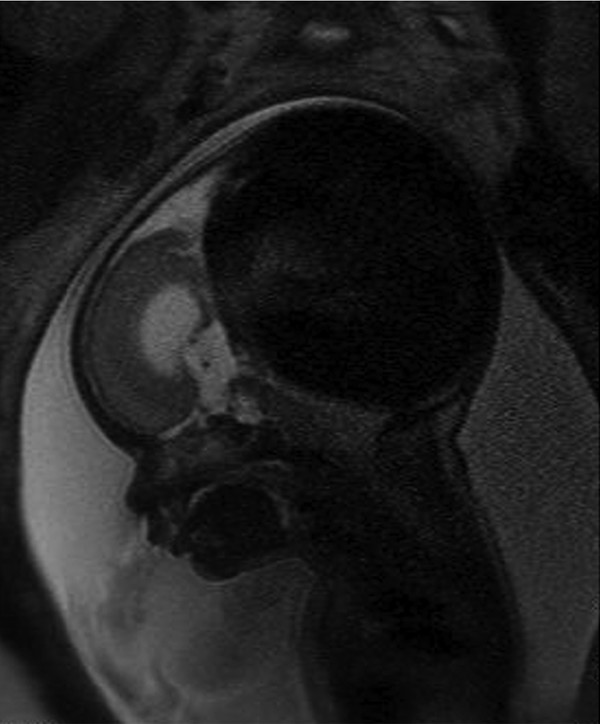
6.2.4 Imaging Findings
A number of imaging techniques can be used to evaluate pediatric dural arteriovenous fistulas (dAVFs) including CTA, MRA/MRI, ultrasound, and conventional angiography. On prenatal and neonatal ultrasounography, dural sinus malformations can be identified by the presence of an enlarged torcular with arterialized flow on Doppler (Fig. 6.5). Occasionally, intraluminal thrombus in the torcula can be seen on intrauterine ultrasound. This may be mistaken for an epidural hematoma. On non-contrast CT, dural sinus malformations classically present with a markedly enlarged torcular. Persistent venous hypertension can result in hydrocephalus as well. In terms of noninvasive neurovascular imaging CTA/V and MRA/V can both be used in evaluation of these lesions. However, given obvious concerns for radiation dose in a patient population that will require multiple sessions of repeat imaging, MRI with MRA/V is preferable.
There are a number of important imaging findings that one must hone in on when evaluating a pediatric patient with a dural arteriovenous shunt. The location of the shunt is important in that it can determine natural history. In general, shunts involving the torcula have a poorer prognosis than those involving either the superior sagittal sinus or transverse sinus (Fig. 6.6). MR venography can be very helpful in evaluating for venous sinus thromboses and stenoses in the draining veins and sinuses. The presence of jugular bulb stenosis is particularly important as it portends a poorer prognosis due to increased rates of venous hypertension and future hemorrhage. MR venograms should also be inspected for the presence of dilated corkscrew pial and medullary veins suggestive of chronic venous hypertension in the setting of cortical venous reflux. On MR angiography, one should evaluate the types of arterial feeders to the fistula with particular attention to the middle meningeal and occipital arteries. MRI may show vasogenic edema in the vascular territory draining the arteriovenous fistula (AVF), if there is chronic venous hypertension. In neonates and infants, this can result in delayed myelination, focal or diffuse venous ischemia, venous infarcts or hemorrhage, severe hydrocephalus, and, in terminal cases, brain calcifications from focal or diffuse melting-brain syndrome (Fig. 6.7).
Dural sinus malformations are unique in that they are typically slower flowing than other dural arteriovenous shunts. Because of this, they are more prone to undergo spontaneous thrombosis (Fig. 6.6). This can result in devastating consequences including venous infarction and intraparenchymal hemorrhage. In cases of spontaneous thrombosis of a dural sinus malformation, the radiologist must try to figure out how the brain is draining. If this occurs late in infancy, following cavernous sinus capture of the basal veins and sylvian veins, the cavernous sinus will be the primary venous drainage outlet. If this occurs prior to cavernous sinus capture, cortical and medullary veins will preferentially be used to drain the brain, resulting in venous hypertension and hemorrhage.
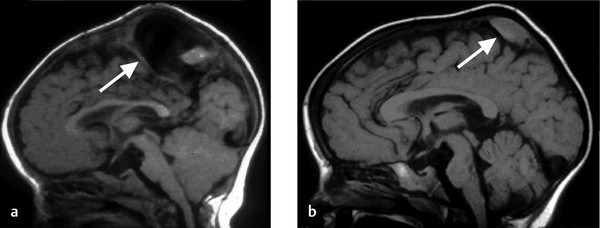
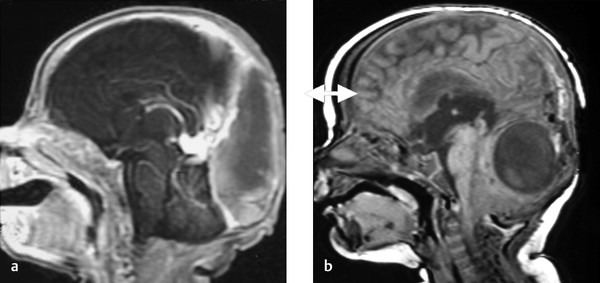
Treatment of these challenging lesions therefore is thus comprised of a staged embolization approach that allows the dural sinuses to remodel. A complete obliteration of all shunts in the neonatal period is contraindicated.
6.2.5 What the Clinician Needs to Know
Classification of the shunt (i.e., dural sinus malformation, infantile dAVF, and adult type dAVF)
The presence of venous thrombosis or venous stenosis
The patency of the jugular bulb
The presence of sylvian vein or basal vein drainage into the cavernous sinus
The location of arterial feeders
Imaging evidence of chronic venous hypertension (i.e., dilated corkscrew medullary veins, vasogenic edema)
6.2.6 High-yield Facts
Dural sinus malformations are characterized by a markedly dilated, “malformed” dural sinus and slow-flow arteriovenous shunts.
Involvement of the torcular is associated with poorer prognosis as compared to involvement of the transverse sinuses alone.
Infantile dural arteriovenous shunts are considered congenital and not the result of venous sinus thrombosis.
Jugular venous stenosis and thrombosis of the draining venous sinus or any other venous outlet obstruction portend a poorer prognosis with higher risk of hemorrhage and venous hypertension.
Thrombosis of a draining vein prior to cavernous sinus capture of the basal and sylvian veins portends a poorer prognosis.
Venous hypertension signs include dilated corkscrew medullary veins and vasogenic edema.
Further Reading
[1] Barbosa M, Mahadevan J, Weon YC, et al. Dural Sinus Malformations (DSM) with Giant Lakes, in Neonates and Infants. Review of 30 Consecutive Cases. Interv Neuroradiol. 2003; 9(4):407–424 [2] Yang E, Storey A, Olson HE, et al. Imaging features and prognostic factors in fetal and postnatal torcular dural sinus malformations, part I: review of experience at Boston Children’s Hospital. J Neurointerv Surg. 2017 [3] Yu J, Lv X, Li Y, Wu Z. Therapeutic progress in pediatric intracranial dural arteriovenous shunts: A review. Interv Neuroradiol. 2016; 22(5):548–556 [4] Krings T, Geibprasert S, Terbrugge K. Classification and endovascular management of pediatric cerebral vascular malformations. Neurosurg Clin N Am. 2010; 21(3):463–4826.3 Pediatric Pial Arteriovenous Fistula
6.3.1 Clinical Case
A 2-day old boy with multiorgan failure.
6.3.2 Description of Imaging Findings and Diagnosis
Diagnosis.
Pial arteriovenous fistula with cerebral atrophy (Fig. 6.8).
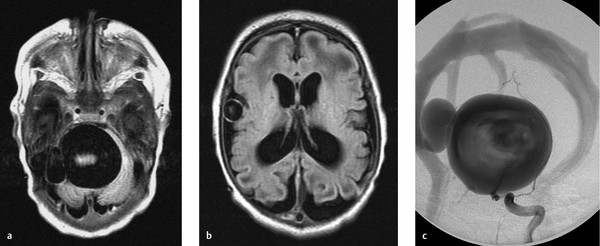
6.3.3 Background
Pediatric pial arteriovenous fistulas are extremely rare intracranial vascular malformations comprising less than 2% of all arteriovenous malformations. To date, less than 200 cases of these lesions have been reported in the literature and their exact incidence is unknown. These lesions usually become symptomatic in childhood, although some of them may be diagnosed prenatally. Neonates with large shunts may present with congestive heart failure, whereas infants present with increased head circumference and focal neurological deficits. Beyond childhood, these lesions can present with headaches, seizures, and focal neurological deficits. Because these lesions consist of high-flow direct shunts between a pial artery and a cortical vein, prognosis is often poor with conservative management due to problems of venous congestion, mass effect of the pouches (Fig. 6.9), or arterial steal from normal brain parenchyma. Natural history data are missing and these shunts cannot be sub grouped into criteria set out in the ARUBA trial or other natural history studies of brain vascular malformations.
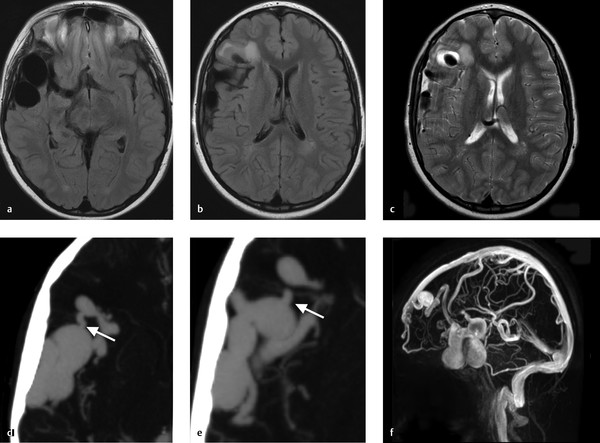
Greater than 90% of pial AVFs are diagnosis before the age of 5. There is a male predominance (M:F = 2:1), similar to that seen in vein of Galen malformations.
Multiplicity of these pial arteriovenous fistulas is a strong predictor for a congenital origin (hereditary hemorrhagic telangiectasia [HHT]).
6.3.4 Imaging Findings
Pial AVFs may be identified on prenatal ultrasound as a dilated high flow shunt with pial dilated arteries and veins without an intraparenchymal nidus. Prenatal diagnosis has not been found to be associated with patient prognosis in most large series of pial AVFs. The key to diagnosis of these lesions is the identification of a dilated pial artery connecting directly into an ectatic and dilated draining vein. At the site of the arteriovenous connection, the vein is usually markedly dilated (Fig. 6.10). Most lesions are located supratentorially with the vast majority of lesions located in the frontal or temporal lobes.
There are a number of important angioarchitectural characteristics that should be relayed to the treating physician in interpreting scans of pial AVF patients. On the arterial side, identification of the arterial feeder, its maximum diameter and the presence of feeding arterial aneurysms or stenoses is important. It is interesting to point out that arterial pathology in these lesions is rare with less than 5% of patients having arterial stenosis or aneurysms. Many lesions will have multiple arterial feeders converging on a single venous pouch.
The neuroradiologist must pay special attention to the venous side, however, as this is what will likely influence the presentation and natural history of the lesion. Venous ectasia is present in up to 90% of patients and should be commented on (Fig. 6.8 , Fig. 6.9 , Fig. 6.10). The presence of pial venous stenosis, seen in 40% of patients, is also important as anything that limits venous outflow is a risk factor for hemorrhage. Patency of the dural venous sinuses is important as the presence of dural venous sinus thrombosis can substantially increase the rupture rate of the fistula. Dural venous sinus thrombosis is present in up to 20% of patients. False venous aneurysms are a potential bleeding source and should be identified (Fig. 6.9).
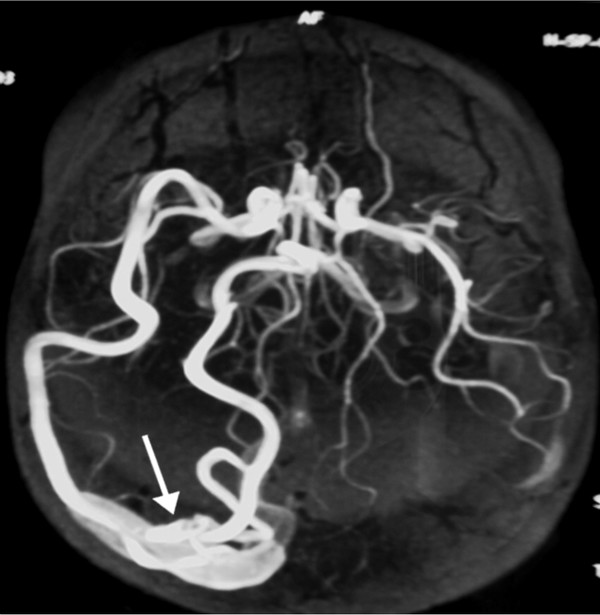
Approximately 40% of patients will have multiple AVFs. By convention, multiple arterial feeders converging on to a single draining vein would comprise a single AVF. AVF multiplicity is defined as multiple distinct arteries feeding different draining veins with distinct fistulous points. AVF multiplicity, or, the presence of an AVF with a concomitant AVM should prompt an investigation for HHT or a RASA1 mutation. Nearly 30% of pial AVF patients have such a mutation.
Hemorrhage from pial AVFs is not rare. There are three distinct patterns of hemorrhage: (1) at the site of the fistula – usually associated with a venous pseudoaneurysm, (2) regional venous hemorrhage due to local venous hypertension from pial venous reflux, and (3) remote venous hemorrhage secondary to global venous hypertension with sinus congestion and pial reflux.
“Melting brain syndrome” is a rare manifestation of these lesions. It is related to a progressive brain apoptosis related presumably to arterial steal from normal brain parenchyma and subsequent brain death. When present, it is usually located in the territory of the fistula (i.e., where the steal occurs). Because there is typically no subpial drainage associated with these AVFs, medullary venous congestion is rare.
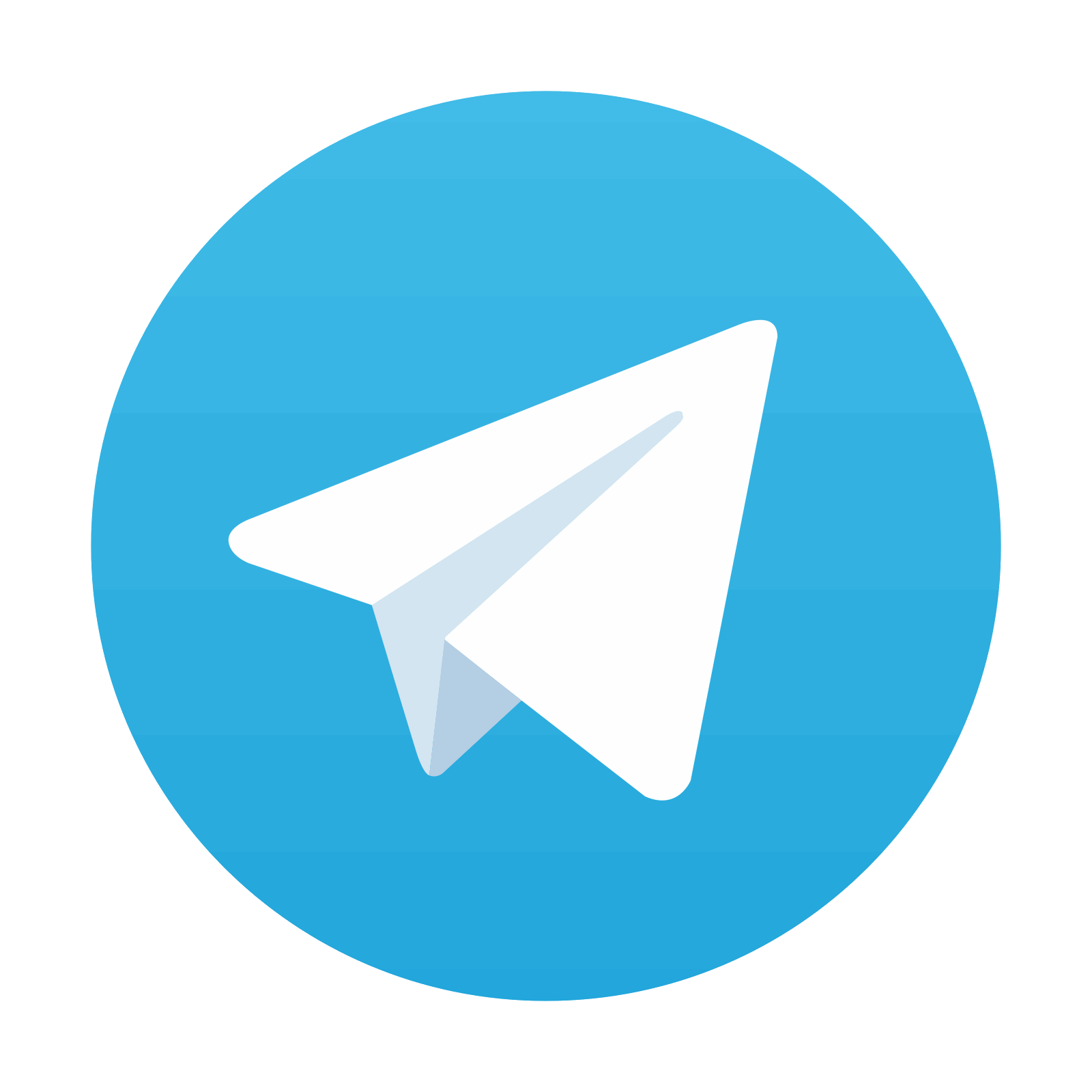
Stay updated, free articles. Join our Telegram channel

Full access? Get Clinical Tree
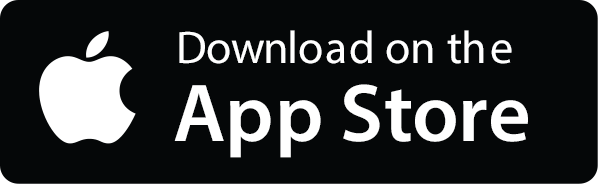
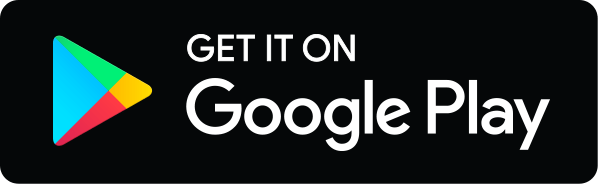
