215CHAPTER 14
Adrenal SBRT
The role of radiation therapy (RT) in the treatment of adrenal tumors has been limited until recent years and, historically, RT for adrenal tumors has been limited to palliation for pain (1–3). However, with the advent of stereotactic body radiation therapy (SBRT; also known as stereotactic ablative radiation therapy [SABR]), which allows for highly conformal, high-dose radiation treatment delivered in a limited number of fractions, the role of radiation in the treatment of adrenal tumors is expanding (4, 5). This chapter reviews the emerging literature on SBRT for primary adrenocortical carcinomas (ACCs) and metastatic lesions in the adrenal glands.
INDICATIONS FOR ADRENAL STEREOTACTIC BODY RADIATION THERAPY
Primary Adrenal Tumors—Adrenocortical Carcinoma
ACC is a rare malignancy with only 9 to 46 cases reported per year between 1973 and 2000, according to the National Cancer Institute’s Surveillance, Epidemiology, and End Results (SEER) database (6). The mainstay of curative-intent therapy for ACC is complete tumor resection and the goal of adjuvant therapy has been to decrease disease recurrence through suppressing adrenal cortical hormone production with the drug, mitotane (7). RT, whether adjuvant or definitive, is rarely used, with large database studies (National Cancer Database [NCDB], and SEER databases) citing only approximately 10% of ACC cases in the United States having received RT as part of their management (6, 8, 9). Unfortunately, up to 85% of patients develop disease recurrence even after complete (R0) surgical resection (10). When used, RT is typically delivered in conventional fractionation at 1.8 to 2 Gy per fraction to the postoperative adrenal bed or an unresectable adrenal tumor (11). One study by Desai et al. described a single case of SBRT with CyberKnife® to a primary ACC tumor delivering 20 Gy over five fractions (12). This patient failed locally 4 months after SBRT and died 11 months after SBRT.
Secondary Adrenal Tumors—Adrenal Metastases
More commonly, malignant tumors of the adrenal gland represent metastases from another primary site. One autopsy study of 1,000 patients with metastatic carcinoma of multiple primary sites revealed that the adrenal gland had metastatic involvement by the primary cancer in 27% of patients (13). This study found that 54%, 36%, 24%, and 21% of breast, lung, renal, and gastric cancer, respectively, had adrenal metastases. Surgery with an adrenalectomy is usually the first-line approach for patients with adrenal metastases, particularly in the setting of oligometastatic disease (defined as having one to five metastases). A pooled analysis by Tanvetyanon et al. evaluated the role of adrenalectomy in patients with non–small-cell lung cancer (NSCLC) with adrenal 216metastases (14). The analysis included 10 publications with 114 patients, 42% of whom had synchronous (disease-free interval [DFI] ≤6 months) metastases and 58% had metachronous (DFI >6 months) disease. Patients with metachronous disease as compared with those with synchronous disease had a greater median overall survival (OS; 31 months vs. 12 months, respectively; p = .02) after adrenalectomy. The 1- and 2-year survival rates were 80% and 52% for metachronous disease and 45% and 30% for synchronous disease, respectively. Approximately one-quarter of all resected patients with both metachronous and synchronous lesions remained alive at 5 years.
Although surgical resection remains the accepted gold standard for patients with adrenal metastases, a select number of patients are not good surgical candidates on the basis of medical comorbidities, difficult anatomy, local tumor invasion, and personal preference. Although there are no randomized controlled trials comparing the efficacy of adrenalectomy with SBRT for adrenal metastases, recent findings that evaluate the use of ablative local RT have been promising. Typically the use of SBRT has been confined to patients with oligometastatic disease who are not amenable to surgical resection in an effort to improve progression-free survival (PFS) (5).
SELECTION CRITERIA FOR ADRENAL STEREOTACTIC BODY RADIATION THERAPY
The published studies evaluating adrenal SBRT are summarized in Table 14.1 (4, 15–24). Patient selection for SBRT was based on a variety of factors. Patients were required to have biopsy-proven diagnosis of malignancy with or without specific biopsy-proven adrenal metastasis (15, 23). If the adrenal metastasis was unable to be biopsied for confirmation, patients could be included if PET scan revealed evidence of metastases (15, 23). Many of the studies based eligibility on limited number of metastases, often requiring an oligometastatic state, and control of systemic disease by either local or systemic therapies (4, 15, 16, 21, 22). However, if adrenal metastases were symptomatic, these patients were also eligible (4, 15, 19, 21). Most studies emphasized inclusion of patients discussed in a multidisciplinary setting who were thought to be poor surgical candidates or who may have refused surgery (16, 18, 20, 21). Patients had to have a good performance status, Eastern Cooperative Oncology Group (ECOG) score of 0 to 2 (16, 23) or 0 to 1 (21), or a Karnofsky Performance Status (KPS) of 70% or greater (20). In these published experiences, patients were excluded if they had uncontrolled, unaddressed systemic disease (15) and if life expectancy was less than 3 to 6 months (20, 23).
On the basis of their review of the literature, demonstrating encouraging 2-year local control rates, Ippolito et al. emphasize that patients selected for SBRT should have a better prognosis, similar to patients who would be considered for surgery. Selection would include patients with limited metastases (less than or equal to five lesions, disease confined to less than or equal to two organs) and a DFI of greater than 6 months (5).
As SBRT delivers very high-dose radiation, individual patient anatomy must also be considered. Depending on tumor size and nearby organs at risk (OARs), some patients may not be ideal candidates for SBRT. Dose constraints to OARs are discussed later in this chapter.
OUTCOMES USING STEREOTACTIC BODY RADIATION THERAPY FOR ADRENAL METASTASES
Reported outcomes in patients undergoing SBRT for adrenal metastases reveal promising survival results (Table 14.1). Oshiro et al. reported 1-year OS of 83%, and 2- and 5-year OS of 56% each for patients with metachronous adrenal metastases (21). This is comparable to patients undergoing adrenalectomy with 1- and 2-year survival rates of 80% and 52%, respectively (14). Similar findings are reported by Guiou et al. with an improved survival in patients with metachronous disease (18). OS rates ranged from 78% to 90% at 1 year and are comparable to the outcomes after adrenal metastasectomy reported in retrospective series (20, 22). Prospective studies comparing the efficacy and toxicity of adrenal SBRT with those of adrenal metastasectomy could provide essential data to assist in selecting the optimal modality on the basis of individual patient and disease characteristics.
IMAGING CHARACTERISTICS OF ADRENAL LESIONS
It is important to note the unique imaging characteristics of an adrenal tumor not only for treatment planning but also for accurate assessment of metastatic disease. Nonfunctioning adenomas are the most common adrenal lesion, although metastases are the most common malignancy of the adrenal gland (25), and it is thus imperative to accurately discern metastases from benign adenomas. On an unenhanced CT scan, a value of equal to or lesser than 10 Hounsfield units (HU) is indicative of a benign lesion with 71% sensitivity and 98% specificity (25). Figure 14.1 displays a right adrenal metastasis. Washout on a contrasted CT scan can also be used to differentiate between benign adenoma and metastatic disease. An absolute washout of 60% or higher is 86% to 88% sensitive and 92% to 96% specific for an adrenal adenoma (25). Adrenal metastases often also present as large (>4–6 cm) masses of the adrenal gland with interval change in size, irregular shape, necrosis, and invasion of nearby structures. The likelihood of such a lesion to represent metastatic disease increases greatly when a patient has a known primary cancer (26). PET-CT can also be used to assess 18-F fluorodeoxyglucose (FDG) avidity suggestive of adrenal malignancy (27) (Figure 14.2).
217
218
FIGURE 14.1 Axial, contrast-enhanced CT showing a right-sided adrenal tumor.
ACC is more easily distinguished from benign disease because these tumors are typically large at the time of initial imaging, often ranging from 4 to 25 cm (28). Common imaging characteristics of ACC include irregular tumor margins, central intratumoral necrosis or hemorrhage, heterogeneous enhancement, invasion into adjacent structures, venous extension (renal vein or inferior vena cava), and calcification (29). On MRI T1-weighted images, ACC is typically iso- to hypointense as compared with the liver (28). MRI is more useful than CT in determining invasion into adjacent structures and vasculature involvement (28). At the time of radiation planning, use of any available diagnostic imaging, including PET-CT, contrast-enhanced CT, and/or MRI, is important for accurate tumor assessment and contour delineation.
FIGURE 14.2 Axial PET-CT demonstrating right-sided adrenal tumor with FDG avidity.
FDG, fluorodeoxyglucose.
STEREOTACTIC BODY RADIATION THERAPY PLANNING AND DELIVERY CONSIDERATION
Motion Management
Patient immobilization is a critical aspect of SBRT planning and delivery and institution-specific devices should be implemented at the time of CT simulation. Abdominal organ motion must be considered when designing SBRT for adrenal lesions. Adrenal motion is primarily in the superior to inferior (SI) direction with usually less than 2 mm of motion in the anterior to posterior or transverse directions (30, 31). Wang et al. specifically evaluated the motion of adrenal gland tumors using fiducials that had been placed for CyberKnife treatment. Fiducials had the greatest motion distance in SI direction with a range of 5 to 12 mm (32). Furthermore, the study found that motion distance was independent of age, height, weight, and tumor size. The authors report that because of the presence of the liver, right-sided adrenal tumors have smaller motion amplitude in the SI direction. There are several mechanisms that can be applied to reduce tumor motion in the planning of SBRT, specifically motion related to respiration. Methods to account for respiratory motion include the use of motion-encompassing imaging 219(i.e., four-dimensional CT [4DCT], slow CT, inhalation and exhalation breath-hold CT), respiratory gating, breath-hold techniques, forced shallow breathing (FSB) with abdominal compression, and real-time tumor tracking (33). Typically, the use of abdominal compression is implemented if tumor motion/diaphragmatic excursion is found to be 5 mm or more at the time of simulation. Use of these methods is often institutionally determined, but tumor motion should ultimately be accounted for in contour delineation and motion should be managed with patient coaching techniques, patient devices, and/or real-time tumor tracking. If the tumor motion is relatively minimal, an internal target volume (ITV) can also be determined based on the 4DCT at simulation. This would encompass the target at its maximal dimensions throughout the breathing cycle. However, when choosing margin expansions necessary secondary to motion in SBRT planning, a treating physician should consider motion artifact in the simulation CT, respiratory cycle variations, and patient-specific tumor motion (33). An ITV was used in five of the 11 studies displayed in Table 14.1. The use of lorazepam to reduce liver motion has also been evaluated in a randomized controlled trial (34), which demonstrated that lorazepam at the time of simulation and treatment could further reduce tumor motion.
Fiducial markers can help to facilitate daily setup and can be used for intrafraction motion assessment to verify appropriate timing of delivery of RT when using respiratory gating. Although fiducial marker placement is not mandatory and routine use of fiducials is dependent on the ability to have interventional radiologists place them in a timely fashion, they can aid in the accuracy of SBRT delivery.
Target Delineation
To contour the target volume, available diagnostic imaging including PET-CT, CT, and/or MRI should be fused with the CT acquired at the time of simulation. The gross tumor volume (GTV) should be delineated on cross-sectional images from the planning CT scans acquired at the time of simulation fused with diagnostic CTs. In many of the studies, the clinical target volume (CTV) was often equal to the GTV with no additional margin (4, 15–17, 21, 24). In those that expanded the GTV to a CTV, a range of 2 to 3 mm was used. Finally, a planning target volume (PTV) is generated to account for daily setup error, breath-hold reproducibility, and internal motion (if an ITV is not defined; 33). In prior adrenal SBRT studies, a margin of 0 to 10 mm was applied as reported in Table 14.1. This margin should be determined institutionally as setup reproducibility is dependent on departmental positioning procedures, immobilization, frequency of portal imaging, and presence or absence of fiducial markers (33).
It is important to also contour OARs as it is imperative to constrain the dose to these critical structures. The critical organs that should be contoured include the spinal cord, kidneys, liver, duodenum, small and large bowel, and stomach. Consensus guidelines put forth by the Radiation Therapy Oncology Group (RTOG) exist for contouring upper abdominal organs and are available online to assist in delineating upper abdominal structures (35). Figure 14.3 displays an axial slice from the RTOG contouring atlas. Table 14.2 displays the contouring guidelines for abdominal organs with definitions from the aforementioned RTOG atlas as well as a current open clinical trial: “Phase 1 Study of Stereotactic Body Radiotherapy (SBRT) for the Treatment of Multiple Metastases” (NRG-BR001 Protocol; 36).
Dose Constraints to Critical Structures
The normal critical structures at risk include the spinal cord, kidneys, liver, duodenum, small and large bowel, and stomach. The dose constraints used in the published adrenal SBRT studies are listed in Table 14.3. Common constraints used in these studies for a five-fraction regimen to the bowel (stomach, duodenum, and small and large intestines) are as follows: maximum point dose less than 42 Gy; V38 Gy less than or equal to 5 mL; V32.5 Gy less than or equal to 15 mL; and V20 Gy less than or equal to 30 mL (15, 16). Per the NRG-BR001 Protocol, dose constraints to OARs are displayed in Table 14.4 for both three- and five-fraction SBRT (36).
Dose Fractionation for Treatment Planning
The studies listed in Table 14.1 have used a wide range of total doses (median dose range of 16–48 Gy) over a range of 1 to 10 fractions. The current clinical trial NRG-BR001 uses a prescription dose of 45 Gy delivered in three fractions with a decreased dose of 42 Gy in three fractions if dose-limiting toxicities are reported. In the single institutional, retrospective review of CyberKnife for adrenal tumors by Desai et al., biological equivalent dose (BED) was found to be the most important predictor for local control (12). The authors report that the duration of adrenal tumor control correlated with calculated mean BED of 76 Gy for local failures versus 96.76 Gy for those who achieved local control. Higher BED correlating with improved local control has been corroborated in other trials as well (16). Specifically, a BED of more than or equal to 100 Gy significantly improves local control in hypofractionated RT in patients treated with SBRT for Stage I NSCLC (37, 38). In the largest retrospective single-institution reported study of SBRT for adrenal metastases including 48 patients published by Casamassima et al., the majority of patients received a dose of 36 Gy delivered in three fractions prescribed to the 70% isodose with 17.14 Gy per fraction at the isocenter (17). The actuarial local control rate was 90% at 1 and 2 years. As reported in their manuscript, the maximum BED10 (biological effective dose for α/β = 10 Gy) value in 36 Gy in three fractions was 137.3 Gy. There were no reported cases of acute gastrointestinal (GI), hepatic, or renal toxicity. There was one reported case of adrenal insufficiency. When deciding upon prescribed dose, the critical structures nearby should be considered to mitigate toxicity. Certainly, hollow viscous organs such as bowel pose challenges in safe dose escalation to the adrenal gland.
220
FIGURE 14.3 Axial contrast-enhanced CT depicting normal upper abdominal structures (35).
Source: Jabbour SK, Hashem SA, Bosch W, et al. Upper abdominal normal organ contouring guidelines and atlas: a Radiation Therapy Oncology Group consensus. Pract Radiat Oncol. 2014; 4(2): 82–89. doi: 10.1016/j.prro.2013.06.004
TABLE 14.2 Contouring Guidelines for Abdominal Organs as per the RTOG Consensus Panel and NRG Oncology
Organ | Contouring Guidelines |
Liver (35) | Gallbladder should be excluded IVC should be excluded when discrete from liver PV should be included in liver contour when caudate lobe is seen to left of PV |
Pancreas (35) | Sits at level of L1 to L3 Pancreatic head sits to right of SMA Uncinate process, extension of head of pancreas, is posterior to SMV that abuts aorta posteriorly Pancreatic body is between celiac trunk and SMA where it lies anterior to aorta Pancreatic tail is to the left of the SMA and SMV |
Stomach (35) | Includes the following: cardia (begins at GEJ), fundus (most cephalad, abuts left hemidiaphragm, left and superior to cardia), body (central, largest portion), antrum (gateway to the pylorus) Oral contrast or water recommended for optimal delineation of gastric wall |
Duodenum (35) | First portion: Begins after pylorus, retroperitoneal after first ~5 cm where it is suspended by hepatoduodenal ligament Second (descending) portion: Starts at superior duodenal flexure, attached to head of pancreas, ~7.5 cm in length, located to right of IVC at levels L1 to L3 Third (transverse) portion: Crosses in front of aorta and IVC and is posterior to SMA and SMV, about 10 cm, marks end of C-loop of duodenum Fourth (ascending) portion: Travels superiorly until it is adjacent to inferior pancreatic body, ~2.5 cm long, lies anteriorly to the IMV until IMV moves medially at the transition to the jejunum |
Spinal cord (36) | The spinal cord will be contoured based on the bony limits of the spinal canal ending at L2 The spinal cord should be contoured starting at least 10 cm above the superior extent of the PTV and continuing on every CT slice to at least 10 below the inferior extent of the PTV221 |
Lung (36) | Both right and left lungs contoured as one structure Contouring should be carried out using pulmonary windows All inflated and collapsed lung should be contoured |
Kidney (36) | Both right and left kidneys, excluding renal pelvis/collecting system, should be contoured in their entirety (the renal cortex) |
Bowel (small and large) (36) | From the ileocecal area to include the ascending, transverse, descending, and sigmoid colon as one structure |
IVC, inferior vena cava; PV, portal vein; SMA, superior mesenteric artery; SMV, superior mesenteric vein; GEJ, gastro-esophageal junction; PTV, planning target volume.
222TABLE 14.4 Dose Constraints for Organs at Risk as per the NRG-BR001 Protocol
| Organ | Dose Constraint |
Three fractions | ||
| Spinal cord | V22.5 Gy <0.03 cm3 |
|
| V13 Gy <1.2 cm3 |
| Stomach | V30 Gy <0.03 cm3 |
|
| V22.5 Gy <10 cm3 |
| Duodenuma | V24 Gy <0.03 cm3 |
|
| V15 Gy <10 cm3 |
| Bowela | V34.5 Gy <0.03 cm3 |
|
| V24 Gy <20 cm3 |
| Kidney, ipsilateral | V12.3 Gy <130 cm3 |
| Kidney, total | V15 Gy <200 cm3 |
| Renal hilum/vascular trunk | V19.5 Gy < 15 cm3 |
| Liver | V17.1 Gy < 700 cm3 |
Five fractions | ||
| Spinal cord | V28 Gy <0.03 cm3 |
|
| V22 Gy <0.35 cm3 |
|
| V15.6 Gy <1.2 cm3 |
| Stomach | V35 Gy <0.5 cm3 |
|
| V26.5 Gy <5 cm3 |
| Duodenuma | V30 Gy <0.5 cm3 |
|
| V18.3 Gy <5 cm3 |
| Bowela | V40 Gy <0.03 cm3 |
|
| V28.5 Gy <20 cm3 |
| Kidney, ipsilateral | NR |
| Kidney, total | V18 Gy <200 cm3 |
| Renal hilum/vascular trunk | V23 Gy <15 cm3 |
| Liver | V21 Gy <700 cm3 |
aAvoid circumferential radiation.
NR, not reported.
Treatment Planning
Planning can be delivered via different platforms. Linear-accelerated-based treatment planning is common and is discussed here. Planning techniques can include three-dimensional conformal radiation therapy (3DCRT)–SBRT or intensity-modulated radiation therapy (IMRT)–SBRT approach. Typically, 7 to 13 static radiation fields are used with equal weighting. The prescription isodose line covering 95% of the PTV is generally 80% to 90% (range of 60%–90%), whereas the maximum dose is 100% (36). This will result in a “hotspot” within the PTV that is greater than the prescription dose. This “hotspot” should be placed within the tumor itself away from OARs (36). Dynamic conformal arcs can also be used to deliver SBRT. Scorsetti et al. used five to seven noncoplanar arcs of 30° (23). Tissue heterogeneity corrections should be used. Patients are usually treated every other day for three or five fractions (18, 36), although treatment has been delivered on consecutive days (16). Image-guided radiation therapy (IGRT) is essential in the delivery of SBRT. Daily imaging should be used to ensure proper positioning and treatment delivery. This can be done using two-dimensional kV imaging to evaluate fiducial marker positions or with a three-dimensional cone-beam CT (CBCT). Acquired pretreatment images should be reviewed by a radiation oncology physician prior to treatment 223delivery. As an example, Figure 14.4 displays a volumetric-modulated arc therapy (VMAT) plan treating a right adrenal metastasis to a dose of 27 Gy in three fractions.
PATIENT FOLLOW-UP
Follow-Up Imaging Protocol
Patients should be followed up for both treatment response and toxicity assessment after a course of SBRT. Holy et al. evaluated patients 6 weeks after completion of SBRT and every 3 months thereafter (19). Alternatively, Rudra et al. evaluated patients 2 to 4 weeks after completion of therapy, then monthly for two additional visits, and then every 3 months until disease progression or death (22). Scorsetti et al. evaluated patients initially every 3 to 4 months with CT scans and clinical exams for the first year, and then every 6 months for years 2 and 3 (23). In the current NRG trial, patients are seen in follow-up initially 35 to 45 days after completion of SBRT with a physical exam and then every 3 months with physical exam and diagnostic imaging (at the discretion of the treating radiation oncologist) for a total of 2 years. Tumor response is commonly evaluated using the Response Evaluation Criteria in Solid Tumors (RECIST) on a contrast-enhanced abdominal CT and/or PET scan (4, 17–19, 21–23).
Acute and Long-Term Toxicity
SBRT for tumors of the adrenal gland has proven to be well tolerated. The most common acute side effects include nausea, vomiting (controlled with antiemetics), and fatigue, all scored as less than grade 3 (4, 18, 19, 22). In one study, gastric ulcers were noted on endoscopy in one patient 2 weeks after delivery of SBRT and gastric and duodenal ulcers in another patient were found 4 weeks after completion of therapy (19). A BED of 72 Gy was applied in both cases. These were effectively treated with oral proton pump inhibitor medications. A grade 2 duodenal ulcer was found in one patient treated to 30 Gy in three fractions with a maximum duodenal dose of 30 Gy (21). This was successfully treated with a histamine-2 receptor antagonist. Late toxicities in reported studies have also been rare. No late toxicities were noted in the studies by Casamassima et al., Chawla et al., Guiou et al., and Scorsetti et al. (4, 17, 18, 23). In the study by Ahmed et al. (n = 13), late toxicities included 224grade 2 fatigue (n = 1), grade 2 abdominal pain (n = 1), grade 2 nausea (n = 1), and grade 1 nausea (n = 1) (15). Rudra et al. evaluated 10 patients undergoing SBRT and reported one late grade 2 adrenal insufficiency approximately 2.5 years after completion of therapy (22). It was noted that this patient was receiving cortisone injections for back pain, but this was scored as an SBRT-related toxicity. Casamassima et al. also reported one case of adrenal insufficiency (17).
FIGURE 14.4 Stereotactic body radiation therapy plan for a right adrenal metastasis from an esophageal primary: VMAT plan using 10-MV photons treating a right adrenal metastasis to a dose of 27 Gy with 100% dose to 95% of the PTV with a maximum hot spot of 115% delivered in three fractions. The dose constraints to OARs were as follows: spinal cord, V8 Gy <1 cm3; liver, D50% <12 Gy, and V15 Gy <700 cm3; stomach, small bowel, and duodenum, V27 Gy <1 cm3, V20 Gy <3 cm3, and V15 Gy <9 cm3; large bowel, V27 Gy <5 cm3, V20 Gy <10 cm3, and V15 Gy <15 cm3; kidneys, each V15 Gy <35%; and heart, V24 Gy <15 cm3.
OAR, organ at risk; PTV, planning target volume; VMAT, volumetric-modulated arc therapy.
In the recent guidelines issued by the Multinational Association of Supportive Care in Cancer (MASCC) and the European Society for Medical Oncology (ESMO), radiation treatment to the upper abdomen poses a moderate emetic risk and a recommendation was made for the use of prophylactic 5-HT3-receptor antagonist medications (39). Another acute or subacute toxicity includes GI ulceration (typically stomach or small bowel). Thus, some practitioners consider prophylactic treatment with a proton pump inhibitor or histamine-2 receptor antagonist to reduce this occurrence.
CONCLUSIONS
The use of SBRT, though infrequent, is an effective and safe treatment option for tumors of the adrenal gland when delivered appropriately. Available data report excellent local control and minimal toxicity. SBRT is a good alternative for patients who are considered poor surgical candidates or who decline surgical adrenalectomy for adrenal metastases. Nonetheless, SBRT is a highly technical treatment modality and should be planned and delivered by experienced radiation oncologists, physicists, dosimetrists, and therapists. Patient immobilization, respiratory/motion management, image guidance, and careful dosimetry are imperative for safe delivery of SBRT. There are still limited data on the use of SBRT in the setting of primary ACC and SBRT may not be appropriate in most cases given the large size of these tumors and the fact that RT for ACC is primarily in the adjuvant setting. SBRT should continue to be explored and used in the treatment of patients with adrenal metastases, and further investigations should be performed to evaluate combining SBRT with novel approaches such as immunotherapy to further improve local control and potentially even impact on systemic control in patients with adrenal metastases.
SUMMARY OF DOSE CONSTRAINTS (TABLE 14.5)
TABLE 14.5 Dose Constraints for Adrenal Tumors Using SBRT
Adrenal | 42–45 Gy in Three Fractions |
Critical Structures | Suggested Dose Limits |
Stomach Duodenum Bowel | Dmax <4 Gy; V38 <5 mL, V32.5 <15 mL, V20 <30 mL |
Spinal cord (canal) | Dmax <15 Gy (three fractions) |
Liver | V17.1 Gy ≤700 mL |
Kidney (bilateral) | V15 Gy <200 mL |
Kidney (ipsilateral) | V12.3 Gy <130 mL |
Kidney (ipsilateral) | V12.3 Gy <130 mL |
SBRT, stereotactic body radiation therapy.
REFERENCES
1. Soffen EM, Solin LJ, Rubenstein JH, et al. Palliative radiotherapy for symptomatic adrenal metastases. Cancer. 1990;65(6): 1318-1320. PubMed PMID: 2155051.
2. Short S, Chaturvedi A, Leslie MD. Palliation of symptomatic adrenal gland metastases by radiotherapy. Clin Oncol (R Coll Radiol). 1996;8(6):387-389. PubMed PMID: 8973857.
3. Zeng ZC, Tang ZY, Fan J, et al. Radiation therapy for adrenal gland metastases from hepatocellular carcinoma. Jpn J Clin Oncol. 2005;35(2):61-67. doi: 10.1093/jjco/hyi020
4. Chawla S, Chen Y, Katz AW, et al. Stereotactic body radiotherapy for treatment of adrenal metastases. Int J Radiat Oncol Biol Phys. 2009;75(1):71-75. doi: 10.1016/j.ijrobp.2008.10.079
5. Ippolito E, D’Angelillo RM, Fiore M, et al. SBRT: A viable option for treating adrenal gland metastases. Rep Pract Oncol Radiother. 2015;20(6):484-490. doi: 10.1016/j.rpor.2015.05.009
6. Kebebew E, Reiff E, Duh QY, et al. Extent of disease at presentation and outcome for adrenocortical carcinoma: have we made progress? World J Surg. 2006;30(5):872-878. doi: 10.1007/s00268-005-0329-x
7. Else T, Kim AC, Sabolch A, et al. Adrenocortical carcinoma. Endocr Rev. 2014;35(2):282-326. doi: 10.1210/er.2013-1029
8. Bilimoria KY, Shen WT, Elaraj D, et al. Adrenocortical carcinoma in the United States: treatment utilization and prognostic factors. Cancer. 2008;113(11):3130-3136. doi: 10.1002/cncr.23886
9. Paton BL, Novitsky YW, Zerey M, et al. Outcomes of adrenal cortical carcinoma in the United States. Surgery. 2006;140(6):914-920. doi: 10.1016/j.surg.2006.07.035
10. Polat B, Fassnacht M, Pfreundner L, et al. Radiotherapy in adrenocortical carcinoma. Cancer. 2009;115(13):2816-2823. doi: 10.1002/cncr.24331
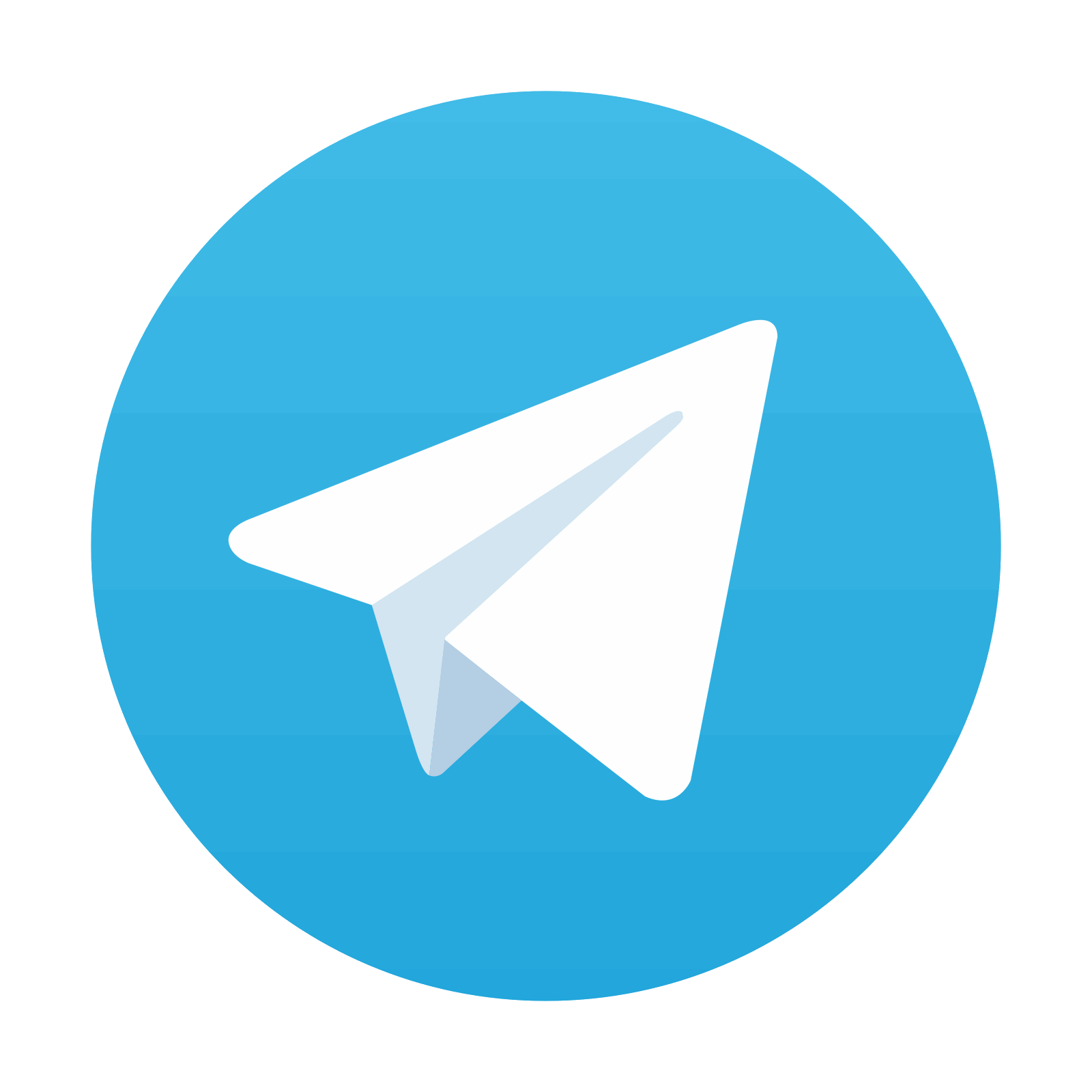
Stay updated, free articles. Join our Telegram channel

Full access? Get Clinical Tree
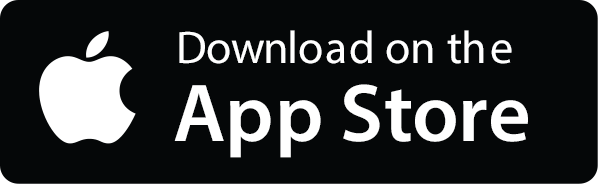
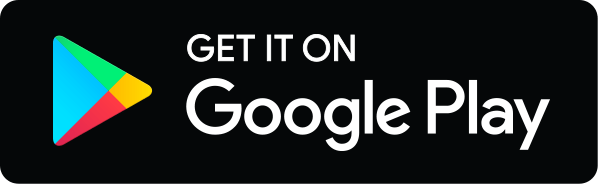