Technical Aspects
Magnetic resonance imaging (MRI) has evolved significantly since its infancy, with major advances in hardware, software, coil developments, sequence development, and contrast agents. Industry and academia continue to advance the field with continued exciting emerging technologies. The discussion in this chapter focuses on the recent emergence of parallel imaging, 3.0-Tesla (T) clinical scanners with high field strength, dedicated contrast agents, perfusion and dynamic contrast-enhanced MRI, and diffusion weighted imaging techniques.
Parallel Imaging
Parallel MRI techniques have been introduced by most vendors. Parallel MRI methods allow for faster imaging. In parallel imaging, multiple independent receiver (phase-array) coils placed in a configuration around the subject enable acquisition of fewer data to avoid aliasing to generate an image. In addition to reducing scan time, the advantages of parallel imaging include higher spatial resolution, less motion artifact on single-shot sequences, and reduced specific absorption rate. Parallel imaging techniques can be particularly utilized on higher field strength magnets to reduce the specific absorption rate.
Clinical High Field Strength Imaging with 3.0-Tesla Imaging
In the past few years, there have been numerous installations of the latest 3.0-T clinical scanners with high field strength at various institutions around the world. In addition to research purposes, there has been increasing use of 3.0-T scanners in clinical settings. High field strength imaging with 3.0 T offers significantly higher signal-to-noise ratio in abdominal tissues, particularly when compared with currently widely available 1.5-T scanning. In 3.0-T imaging, there is approximately a twofold increase in signal-to-noise ratio. Many studies have examined the use of 3.0-T scanners in musculoskeletal and neurologic imaging applications.
Various technical issues are unique to 3.0-T imaging. For example, there is an increased specific absorption rate of approximately four times the radiofrequency deposition compared with 1.5 T. Chemical shift and susceptibility artifacts are also increased significantly. Given that there is increased field strength, the T1 relaxation time is also increased for most tissues. The T2* relaxation of tissues is shorter, and that of T2 is nearly identical. Studies are still ongoing in body imaging with high field strength and show significant promise.
Novel Tissue-Specific Contrast Agents
Liver-Specific Magnetic Resonance Imaging Contrast Agents
In recent years, liver-specific MRI contrast agents have been developed specifically targeted to hepatocytes or reticuloendothelial cells, providing either positive or negative enhancement after intravenous administration. Hepatocyte-specific contrast agents include mangafodipir trisodium and two gadolinium chelates: gadoxetic acid and gadobenate dimeglumine. Reticuloendothelial cell–specific agents include superparamagnetic iron oxides (SPIOs): ferumoxides and ferucarbon.
Mangafodipir Trisodium.
Manganese (mangafodipir trisodium [Mn-DPDP; Teslascan]) is similar to gadolinium and is strongly paramagnetic. Manganese is cleared from the body primarily by biliary or intestinal secretion. It appears to have an affinity for metabolically active tissues, including primarily the liver, and has been marketed with U.S. Food and Drug Administration (FDA) approval for liver lesion characterization. Mangafodipir trisodium contains Mn 2+ , a transitional element that exhibits paramagnetic properties because of five unpaired electrons and shortens the T1 relaxation time. Because of its chemical similarity to vitamin B 6 , it is specifically taken up by hepatocytes. However, evidence exists that metabolic products of this compound are also responsible for selective uptake into liver, pancreas, and cardiac muscle. This compound is administered through slow intravenous infusion over 2 to 5 minutes, and the effect is seen within a few minutes (~15 minutes) and lasts for 24 hours. The adverse events commonly reported include facial flushing and a sensation of heat. The prolonged imaging window makes this agent a good choice for imaging the liver. The signal intensity of normal liver parenchyma is increased, providing high lesion-to-liver contrast. This helps in detecting small liver lesions. This agent may be taken up by well-differentiated hepatocellular carcinoma and thus may mask the tumor. Mangafodipir trisodium is best used for the detection of metastases. During the later phases, it is excreted into bile and provides excellent details of the biliary ducts and aids in the diagnosis of various biliary pathologic processes such as biliary obstruction and bile leaks.
Gadolinium Chelates.
Two gadolinium chelates exhibit liver specificity owing to their selective uptake by hepatocytes through a carrier-mediated transport across the cell membrane. These include gadoxetic acid (Gd-EOB-DTPA; Primovist/Eovist) and gadobenate dimeglumine benzyloxy-propionic tetraacetic acid (Gd-BOPTA; MultiHance). These agents are excreted into the bile unaltered and are ultimately excreted through urine and feces. After intravenous administration, these agents have an initial intravascular phase similar to that of other gadolinium chelates and during the later phase they accumulate in the liver parenchyma and increase the signal intensity of the liver. Thus, the lesion-to-liver contrast is increased and these agents aid in the detection of small liver lesions. This phase is the hepatocyte phase, which is 10 to 20 minutes after administration of Gd-EOB-DTPA, or 40 to 120 minutes after administration of Gd-BOPTA. The initial intravascular phase helps in characterization of liver lesions similar to that obtained with any other gadolinium chelate. Similar to mangafodipir trisodium, these agents provide excellent details of the biliary ducts during the delayed phase.
Ferumoxides.
SPIO nanoparticles are the basis for agents known as ferumoxides (Feridex/Endorem). Ferumoxides are composed of a central iron oxide particle—Fe 2 O 3 —which is a superparamagnetic compound surrounded by a dextran coating. These agents have a greater magnetic susceptibility than conventional gadolinium-based contrast agents. Their dominant effect is preferentially on T2 and T2* shortening rather than T1 shortening. Water near these particles therefore becomes dephased by local inhomogeneities, resulting in signal loss and thus acting as a negative contrast agent.
Of note, these agents should be infused slowly over a period of 30 minutes to avoid cardiovascular effects and lumbar pain. Specificity of particles depends on the size of the particle because the amount of contrast depends on the degree of uptake by the Kupffer cells. For instance, SPIOs are preferentially taken up by Kupffer cells in the liver, whereas ultra-small iron oxide particles (USPIOs) are taken up by macrophages in lymph nodes, liver, lung, and spleen. Similar to mangafodipir trisodium, these agents provide prolonged periods for imaging after intravenous infusion, facilitating higher resolution thin-section imaging.
One agent, Feridex, approved by the FDA since 1996, acts as a negative contrast agent when imaging hepatic lesions and may enable detection of smaller lesions. Ferumoxtran-10 (Combidex) is another type of investigational SPIO-based agent that aims to differentiate normal lymph nodes from lymph nodes with metastatic cancer involvement. With potential applications for Combidex in breast cancer and prostate cancer, clinical studies have thus far demonstrated that uptake occurs preferentially in normal lymph nodes; as a result, any lymph node with retained signal intensity would be of concern for metastatic disease.
Ferucarbotran.
Similar to ferumoxides, ferucarbotran (Resovist) contains both a polycrystalline iron oxide core (Fe 2 O 3 and Fe 2 O4) and a carbodextran coating. Unlike ferumoxides, this agent can be safely injected as a bolus with significantly fewer adverse cardiovascular events or back pain. Ferucarbotran improves focal liver lesion detection on T2- and T2*-weighted sequences.
Blood-Pool Contrast Agents
Macromolecular contrast agents, namely, blood pool agents, also are being developed for vascular and perfusion imaging. Another dedicated contrast agent, gadobenate dimeglumine (Gd-BOPTA), is used in the imaging of liver malignancies. The structure of gadobenate dimeglumine is similar to that of the commonly used gadolinium diethylenetriamine pentaacetic acid (Gd-DTPA), with the addition of a benzene ring and short carbon chain, allowing the agent to be preferentially localized to hepatocytes. Gadobenate dimeglumine is typically seen to demonstrate uptake into hepatocytes and excretion into the biliary system at 30 to 120 minutes.
Because gadobenate meglumine circulates in the intravascular space for a longer time, there is a significant reduction in T1 relaxation time of blood containing the agent. Therefore, magnetic resonance angiography has become a useful application for this contrast agent. Although these agents do not diffuse through normal capillary wall, they can diffuse through defective capillaries commonly found in tumor vasculature, possibly playing a role in measuring the permeability of tumor microvasculature. For example, Gadomer-17 is a polymeric compound with a high molecular weight that because of its hydration properties has already demonstrated utility in measuring myocardial perfusion and in tumor imaging. Interestingly, although not yet ready for clinical use, novel agents involving the chelation of ligands such as antibodies to gadolinium for targeted imaging are undergoing active preclinical development.
Lymph Node–Specific Magnetic Resonance Imaging Contrast Agents
Lymph node–specific contrast agents also are actively being developed. Although not yet approved, these agents demonstrate significant promise in the evaluation of lymph node involvement in the setting of malignancies. For example, much promise has already been demonstrated in differentiating benign from malignant lymph nodes in prostate cancer using USPIO agents. The smaller size of USPIOs compared with SPIOs as well as their hydrophilic coating result in longer circulation in the intravascular space and rapid accumulation in the reticuloendothelial system. Therefore, as these particles are phagocytosed by macrophages, they accumulate in the lymphatic system, resulting in signal drop on T2* images in normal lymph node tissue. However, malignant involvement is devoid of macrophages, resulting in a higher signal intensity. Typically, it takes 24 to 36 hours for the contrast agent to accumulate in lymph nodes, and thus postcontrast imaging is usually obtained 24 hours after its administration. The currently available contrast agent in this group for research use is ferumoxtran/AMI-227. Another agent also under investigation is gadofluorine-M.
Diffusion Weighted Imaging in the Abdomen
Diffusion weighted imaging (DWI) has been implemented mainly in brain imaging and has made a significant impact on imaging in stroke patients. Because of its susceptibility to motion, the application of DWI to the abdomen has until recently faced numerous obstacles. However, with the advent of the echoplanar technique minimizing the effect of physiologic motion such as respiration and cardiac movement, DWI has become applicable to the abdomen. DWI has been suggested as a tool to distinguish different tissue compartments and detect changes in cellular tissue structures and viability. DWI has been discussed as a cancer biomarker in a consensus meeting and a publication on consensus and recommendations for DWI as a cancer biomarker has been published recently highlighting the potential of this promising technique in cancer patients. For example, DWI was applied to differentiate benign from malignant lesions in the liver and was also reported to enable early assessment of treatment response in hepatocellular carcinoma. Malignant liver tumors, probably the result of increased cellular density and altered nuclear-to-cytoplasmic ratios, exhibit restricted water molecule diffusion and therefore reduced apparent diffusion coefficients (ADCs) compared with benign lesions. Because of the variation of sequences available by vendors and the extremely high sensitivity of DWI measurements to motion, this technique is extremely operator dependent.
Perfusion Imaging and Dynamic Contrast-Enhanced Magnetic Resonance Imaging
Whereas standard cross-sectional imaging techniques solely focus on morphology, another technique that moves beyond anatomy and into physiologic or functional assessment includes the use of dynamic contrast-enhanced MRI (DCE-MRI). In the literature and various investigations, this technique has been applied to the evaluation of tumor angiogenesis. Kinetic modeling of imaging data plays an important role for DCE-MRI, because quantitative parameters allow for the characterization of physiologic data such as blood volume, vascular permeability, and vessel density. DCE-MRI holds significant promise in monitoring the effect of antiangiogenic agents because morphologic changes in tumor size often lag behind physiologic changes, which can be better characterized by enhancement characteristics.
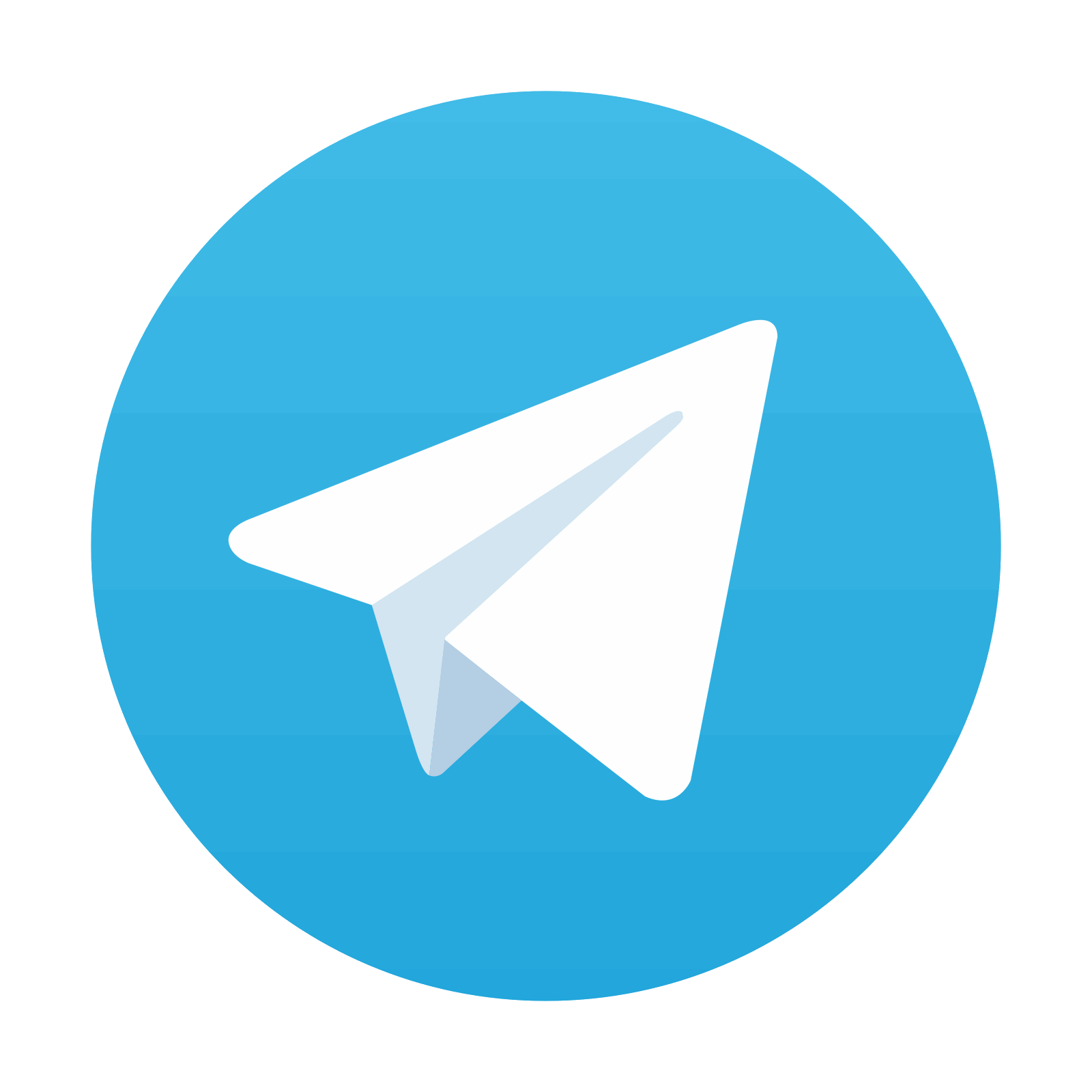
Stay updated, free articles. Join our Telegram channel

Full access? Get Clinical Tree
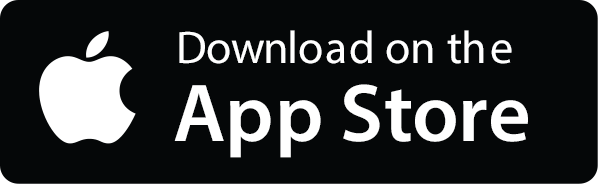
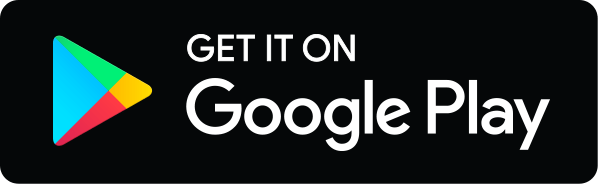