Bone tumors
Metastases
Myeloma
Lymphoma
Enchondromatosis (including variants)
Multiple hereditary exostoses
Langerhans cell histiocytosis
Multifocal osteomyelitis
Hyperparathyroidism with brown tumors
Soft tissue tumors
Neurofibromatosis
Schwannomatosis
Myxomas (Mazabraud syndrome)
Angiomatosis
Lipomas (including hereditary lipomasosis)
Desmoid tumors
Initial Tumor Evaluation
The radiograph remains the initial imaging study for evaluating both bone and soft tissue lesions. For osseous lesions, it is almost invariably the most diagnostic. The radiograph accurately predicts the biologic activity of a bone lesion, which is reflected in the appearance of the margin of the lesion, and the type and extent of accompanying periosteal reaction. In addition, the pattern of associated matrix mineralization may be a key to the underlying histology (e.g., cartilage, bone, fibro-osseous) [2–5]. Although other imaging modalities (MR and CT) are superior to radio — graphs in staging a bone lesion, the radiograph remains the best modality for establishing a diagnosis, for formulating a differential, and for accurately assessing the biologic activity (separating benign from malignant lesions). In many cases, as in patients with fibroxanthoma (nonossifying fibroma), fibrous dysplasia, osteochondroma or enchondroma, radiographs may be virtually pathognomonic, and no further diagnostic imaging is required. In other cases, despite not having an unequivocal diagnosis, a benign-appearing, asymptomatic lesion may require only continued radiographic follow-up in order to document long-term stability.
Radiographs are typically considered unrewarding in the assessment of soft tissue masses; however, a recent study of 281 patients showed calcification in 27%, bone involvement in 22% and fat in 11% of cases [6]. Such features may be essential in establishing an appropriate imaging diagnosis, establishing a differential, and in assessing malignant potential. Radiographs may also be diagnostic of a palpable lesion caused by an underlying skeletal deformity (such as exuberant callus related to prior trauma) or exostosis, which may masquerade as a soft tissue mass. The soft tissue calcifications and/or ossification identified on radiographs can be suggestive, and at times highly characteristic, of a specific diagnosis. For example, they may reveal the phleboliths within a hemangioma, the juxta-articular osteocartilaginous masses of synovial chondromatosis, the peripherally more mature ossification of myositis ossificans, or the characteristic bone changes of other processes with associated soft tissue involvement. When not characteristic of a specific process, soft tissue calcification can suggest certain diagnoses. For example, nonspecific dystrophic calcifications within a slowly growing lower extremity mass in an adolescent or young adult should suggest a synovial sarcoma as the diagnosis of exclusion.
Advanced Imaging Techniques
MR imaging is the preferred modality for evaluating soft tissue lesions. It provides superior soft tissue contrast, allows multiplanar image acquisition, obviates the need for iodinated contrast agents or for ionizing radiation, and is devoid of streak artifact commonly encountered with CT imaging [7–10]. When clinical findings are equivocal, MR imaging evaluation can confirm the presence of a soft tissue lesion or reassuringly identify a suspected „bump” or „mass” as normal tissue [11]. Whereas MR imaging has emerged as the preferred advanced imaging modality for the evaluation of soft tissue lesions, CT and MR imaging are often complimentary modalities for the evaluation of primary osseous tumors [10]. In a study by Tehranzadeh et al., the information obtained from CT and MR imaging was additive in 76% of malignant primary bone neoplasms [10]. CT scanning is superior to MR imaging for the detection and characterization (osteoid or chondroid) of matrix mineralization, cortical involvement and periosteal reaction [9, 10]. Although some studies suggest that CT and MR are equivalent for the evaluation of the local extent of tumor [12], most studies note the superiority of MR imaging [9, 10, 13–15].
Magnetic Resonance Imaging
While there is no single best tumor protocol, we feel MR imaging should be performed in at least two orthogonal planes, typically including both T1- and T2-weighted images in the axial plane, supplemented by sagittal and/or coronal images, depending on the location of the lesion. The long axis imaging should include both T1 and a water- sensitive sequence, such as short tau inversion-recovery (STIR) or fat-suppressed T2-weighted sequence. It has been our experience that spin-echo imaging is often most useful in establishing a specific diagnosis when possible, and is the most reproducible technique, and the one most often referenced in the tumor imaging literature. It is the imaging technique with which we are most familiar for tumor evaluation, and it has established itself as the standard by which other imaging techniques must be judged [16]. The main disadvantage of spin-echo imaging remains the relatively long acquisition times, particularly for double-echo T2-weighted sequences [16]. This is especially problematic in areas susceptible to respiratory motion. Some time savings have been obtained by increasing the number of echoes collected per excitation, in so-called turbo spin-echo or fast spin-echo (TSE/FSE) sequences, which have now generally replaced the traditional spin-echo sequences. Recent advances have made it possible to extend this concept so that an entire T2-weighted image set can now be obtained in a single breath-hold [17–19].
Rapid, fluid-sensitive imaging techniques comprise both spin-echo and gradient-echo design. Both approaches acquire all the echoes necessary for creation of a single image during the course of a single TR (i.e, after a single excitation pulse, or radio frequency). In a sense, then, these resemble CT, in that slices are obtained separately and sequentially, restricting motion artifact to only those slices acquired while motion is occurring. The term single-shot (SS) is commonly used to describe this approach. Of the SS techniques, the spin-echo-based sequences are the most commonly employed. Depending on the vendor, these are variously labeled HASTE, SSTSE or SS-FSE. Not only are these relatively motion insensitive, and fast enough to allow for breath-hold imaging, but they are exquisitely sensitive to the presence of fluid, improving contrast with soft tissue [20–22]. HASTE is an important part of the technical arsenal for T2-weighted body MR imaging, where it is, for example, the backbone of magnetic resonance cholangiopancreatography (MRCP) imaging [22]. Because of the modifications of the traditional spin-echo sequence required to shorten it to a breath-hold, a significant drawback of the HASTE-type sequence is a low signal-to-noise ratio (SNR). Sensitivity to the SNR issue is important to avoid starving the images of signal by, for example, over-r-eduction of slice thickness or field-of-view.
Although also easily acquired in a breath-hold, the gradient- echo (GRE) version of SS imaging (trueFISP/FIESTA/ balanced FFE), which is based on steady-state principles, provides a different kind of contrast. Immediately noticeable is the high signal intensity of flowing blood, compared with the usual signal void produced by a spin-echo sequence. This characteristic can be nicely exploited for unenhanced vascular imaging. By the same token, however, the concatenation of bright vessels and bright fluid collections can be visually daunting, and can at times obscure structures of interest. In addition, because trueFISP has mixed T1- and T2-weighting, tissue contrast is less than optimal. On the other hand, an important strength of the trueFISP-like sequences is its improved SNR, which can be exploited in the service of spatial resolution [22–25]. Furthermore, for motion studies, as in cardiac or pelvic floor evaluation, these sequences far outperform the spin-echo-based SS techniques [23]. When incorporating trueFISP or its cousins into a protocol, it is important to consider its GRE origin, since the technique does not perform as well in the presence of metal or air, with greater risk for magnetic susceptibility artifact.
GRE imaging techniques may be a useful supplement for demonstrating hemosiderin because of their greater magnetic susceptibility and, in general, susceptibility artifacts related to metallic material, hemorrhage and air are accentuated on GRE images [26]. STIR sequences are very useful in evaluating subtle marrow abnormalities and can be performed more quickly than T2-weighted spin-echo sequences [27], being frequently added to the conventional spin-echo sequences. Some prefer short TE/TR spin-echo and STIR sequences to typical T1- and T2-weighted spin-echo sequences. Although STIR imaging increases lesion conspicuity [27, 28], it typically has lower SNRs than spin-echo imaging and is also more susceptible to degradation by motion [16, 29]. Additionally, STIR and other fat-suppressed imaging techniques reduce the variations in signal intensities identified on conventional spin-echo MR imaging, variations that are most helpful in tissue characterization.
For suspected malignant extremity lesions, such as when osteosarcoma is a differential consideration, it is essential that at least one long axis sequence be performed through the entire involved bone in order to evaluate for the presence of skip metastases. Skip metastases represent a second site of disease in the same bone as the primary tumor but are separated by an area of normal marrow. The identification of skip lesions has clinical implications, and this additional imaging should be performed in appropriate cases.
Computed Tomography Scanning
As previously noted, CT scanning is superior to MR imaging in the detection and characterization of matrix mineralization (osteoid or chondroid), cortical involvement and periosteal reaction [9, 29]. CT is uniquely suited to assess internal matrix when such matrix is obscured by lesion marginal sclerosis and not adequately evaluated by radiographs [30]. We find CT especially useful in the assessment of both bone and soft tissue lesions in those areas in which the osseous anatomy is complex, such as in the spine or small bones of the hands and feet, or where the radiographic osseous detail is obscured by overlying soft tissue, such as in the pelvis.
Although initial investigations maintained that CT is superior to MR imaging in detecting destruction of cortical bone [9, 10], more recently it has been suggested that these two modalities are comparable in this regard [31]. It has also been our experience that nonmetallic foreign bodies may be difficult to identify on MR imaging. In such cases, imaging may show the changes associated with the foreign body, although the foreign body itself may have no signal and may be difficult to identify when small.
Contrast Enhancement
Contrast administration may be a useful adjunct in the assessment of both bone and soft tissue lesions; however, its use is usually dictated by the objectives of the examination. For example, in the CT evaluation of osseous lesions, it is typically not required to assess the presence or character of matrix mineralization, periosteal reaction or marginal sclerosis. On MR imaging, many lesions are well assessed without contrast. As a rule, we find it of little value in the assessment of lipoma or the usual atypical lipomatous tumor (atypical lipoma). In some cases, contrast administration can also cause confusion, blurring the distinction of tumor from peritumoral edema and normal from abnormal marrow [14, 32]. Nevertheless, it may provide essential information and we find contrast imaging especially important in the assessment of lesions containing hemorrhage, myxomatous areas, or necrotic or cystic regions. Only vascularized tissue enhances; therefore, contrast enhanced imaging may be quite useful in directing biopsy to the solid, enhancing portions of a lesion, the portion of the lesion that harbors the diagnostic tissue, as opposed to the cystic, necrotic or hemorrhagic nondiagnostic components. Additionally, the pattern of enhancement may also provide clues to the diagnosis, as in the characteristic peripheral and septal enhancement pattern seen with hyaline cartilage neoplasms.
Contrast-enhanced imaging is not without a price. The use of intravenous contrast increases the length and cost of the examination. Although contrast-enhanced MR imaging may provide additional information, it usually does not increase lesion conspicuity nor replace the diagnostic value of T2-weighted imaging [33]. While MR contrast agents are safer than those used with CT, there is a small, but real, incidence of untoward reactions including hypotension, laryngospasm, bronchospasm and anaphylactic shock [34–36]. Most recently, the association of nephrogenic systemic fibrosis (NSF) with the use of gadolinium-based contrast agents has focused greater attention on its routine administration [37–39]. NSF was first reported in 2000 as a scleroderma-like fibrotic skin disorder in patients with renal insufficiency [38–40]. In a large 10-year study of 8,997 patients receiving gadolinium- based contrast, 15 (0.17%) developed NSF, all of whom had renal failure with glomerular filtration rates of less than 30 mL/min [37]. In a study at four US university tertiary care centers involving over 216,000 patients, NSF was found to be more than 15 times more common with gadodiamide (Omniscan™) than gadopentetate dimeglumine (Magnevist®) [38]. The development of NSF has also been associated with high cumulative doses of gadolinium-based contrast, leading to the recommendation that half-strength contrast be given to patients with glomerular filtration rates of less than 60 mL/min [37, 41–43]. Consequently, contrast-enhanced imaging should be reserved for those cases in which the results influence patient management.
Bone Scintigraphy
Bone scintigrapy with 99mTc phosphate compounds remains the modality of choice for the identification of multifocal osseous disease. Positron emission tomography (PET) has established itself as the gold standard for metabolic imaging, and while it has not replaced skeletal scintigraphy, it has several intrinsic advantages. It has inherently superior spatial resolution and routinely includes tomographic images with multiplanar capability. Additionally, unlike skeletal scintigraphy, which primarily evaluates only the skeletal system, PET detects disease in both the osseous structures as well in the soft tissues, allowing detection of not only the primary tumor, but pulmonary, as well as nodal, metastasis [44]. Moreover, PET detects the presence of tumor directly, by reflecting its metabolic activity, rather than indirectly as with conventional scintigraphy, by demonstrating tumor involvement due to its increased bone mineral turnover [44].
Staging
The staging of musculoskeletal tumors is one of the primary functions of imaging. Accurate staging is essential for appropriate planning of therapy and establishing of prognosis. Simply stated, the purpose of a staging system is to provide a standard manner in which to readily communicate the state of a malignancy. Accurate staging is essential to (a) incorporate the most significant prognostic factors into a system that describes progressive degrees of risk to which a patient is subject, (b) delineate progressive stages of disease that have specific implications for surgical management, and (c) provide guidelines to the use of adjunctive therapies [45]. The staging systems most commonly used are the Enneking system and the staging system of the American Joint Committee. Staging requires a knowledge of the histologic grade of the lesion, as well as its anatomic extent, and can only be completed following biopsy.
Enneking Saging System
The surgical staging system most commonly used in the evaluation of musculoskeletal tumors is that of Enneking [45]. The Enneking staging system is based on the surgical grade of a tumor (G), its local extent (T), and the presence or absence of regional or distant metastases (M) [45]. The Enneking staging system was designed for the evaluation of musculoskeletal mesenchymal tumors and was not intended for use with lesions derived from marrow or the reticuloendothelial system, because of their different natural history, surgical management and response to treatment.
Lesions are divided into two grades: low (G1) and high (G2), on the basis of histologic appearance. In general, low-grade lesions are well-differentiated and have a low potential for the development of metastatic disease. In contrast, high-grade lesions are in general poorly-differentiated, with a high mitotic rate and aggressive clinical course. Local extent is divided into lesions that are intracompartmental (T1) and extracompartmental (T2). The designation of intracompartmental indicates the lesion remains confined to the compartment of origin. For osseous lesions, each bone is considered to be a distinct compartment. When a lesion is designated as extracompartmental, this indicates it has extended beyond the compartment of origin. For example, an osteosarcoma is extracompartmental when it has extended from the bone into the adjacent soft tissue or joint. The presence (M1) or absence (M0) of regional or distant metastases is the third and final component of staging. On the basis of these considerations, lesions are staged as shown in Table 1.
Table 1
Enneking surgical staging of musculoskeletal sarcomas
Stage | Grade | Site |
---|---|---|
IA | Low (G1) | Intracompartmental (T1) |
IB | Low (G1) | Extracompartmental (T2) |
IIA | High (G2) | Intracompartmental (T1) |
IIB | High (G2) | Extracompartmental (T2) |
III | Any (G) | Any (T) with metastases |
American Joint Committee on Cancer Staging System
The Enneking system is well-suited for the evaluation of extremity lesions because of its emphasis on compartmentalization. It does not, however, consider tumor size or distinguish between regional lymph node and distant metastases. In addition, the division of all lesions into either high- or low-grade may not be sufficient to be applicable to the wide range of all sarcomas. An alternative staging system is that of the American Joint Committee on Cancer (AJCC), which is based on the tumor, node and metastasis (TNM) classification [46]. This system is more complex, with four stages and several subclassifications.
The AJCC staging system addresses the surgical grade of a tumor (G), its size and local extent (T), the presence or absence of nodal involvement (N), and the presence or absence of distal metastasis (M) [46]. Box 2 and Table 2 list the classification criteria and subsequent surgical staging.
Box 2
American Joint Committee on Cancer (AJCC) staging of bone sarcomas
The AJCC staging system utilizes the TMN system, described below, to assess the anatomic extent of disease: | |
T | Extent of primary tumor |
N | Absence or presence of regional nodal involvement |
M | Absence or presence of distant metastasis |
Histologic grade (G): | |
G1 | Well-differentiated |
G2 | Moderately well-differentiated |
G3 | Poorly differentiated |
G4 | Undifferentiated |
Primary site (T): | |
T1 | Tumor 8 cm or less in greatest dimension |
T2 | Tumor more than 8 cm in greatest dimension |
T3 | Discontinuous tumors in the primary bone site |
Nodal involvement (N): | |
N0 | No regional lymph nodal metastases |
N1 | Regional lymph nodal metastases |
Distant Metastasis (M) | |
M0 | No distant metastasis |
M1a | Lung metastasis present |
M1b | Metastasis other distant sites, including lymph nodes |
Table 2
American Joint Committee on Cancer (AJCC) staging of bone sarcomas
Stage | T | N | M | Histologic grade (G) |
IA | T1 | N0 | M0 | G1,2 Low grade |
IB | T2 | N0 | M0 | G1,2 Low grade |
IIA | T1 | N0 | M0 | G3,4 High grade |
IIB | T2 | N0 | M0 | G3,4 High grade |
III | T3 | N0 | M0 | Any G |
IVA | Any T | N0 | M1a | Any G |
IVB | Any T | N1 | Any M | Any G |
Any T | Any N | M1b | Any G |
Diagnosis: Bone Tumors
As previously noted, the radiograph remains the most diagnostic imaging study. While more advanced imaging provides the information needed for accurate staging, diagnosis (or differential diagnosis) begins with the radio — graph and is based on the morphology of the lesion, the location of the lesion and the age of the patient. Morphology characterizes the presence and character of the lesion’s margin and periosteal reaction; features which are a function of the interaction between the lesion and the host, and are a key to biologic behavior. Morphology also includes an analysis of matrix. If present, a mineralized matrix may be a key to the lesion histology
The location of a lesion is a major consideration in establishing a diagnosis and structuring a differential diagnosis. There are two components to the location of an osseous lesion: the anatomic location (which bone) and the lesion’s location within the bone (epiphysis, meta — physis, metadiaphysis or diaphysis). The latter is critical in assessment of long bone lesions and is an essential principle of diagnosis pictorially presented in Fig. 1. Age is also a critical consideration, since specific lesions tend to occur in specific age groups (Tables 3, 4) [47]. For example, Langerhans cell histiocytosis localized to bone typically occurs in children and adolescents, while osseous lymphoma is most often encountered in mature adults, usually in the sixth and seventh decades. The final diagnosis, or differential, is then based on the integration of all of the above factors, taken in consideration of the prevalence of tumors within the population.
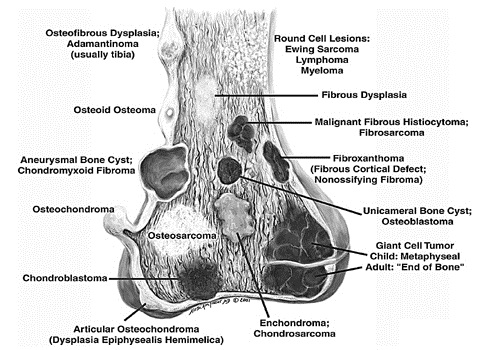
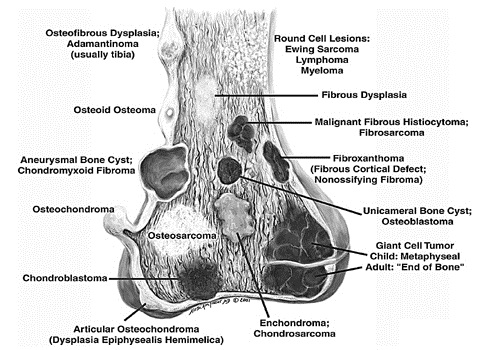
Fig. 1
Classic sites for bone tumors
Table 3
Age distribution of malignant osseous tumors
Tumor type | Overall incidence (%) | Incidence by decade (%) | ||||||||
---|---|---|---|---|---|---|---|---|---|---|
1 | 2 | 3 | 4 | 5 | 6 | 7 | 8 | 9+ | ||
Osteosarcoma | 29.2 | 4.6 | 46.0 | 17.2 | 8.8 | 7.6 | 6.5 | 6.7 | 2.4 | 0.3 |
Myeloma | 14.4 | 0.1 | 1.2 | 5.2 | 15.7 | 29.2 | 29.2 | 15.8 | 3.4 | |
Chondrosarcoma | 15.8 | 0.6 | 4.8 | 12.3 | 21.4 | 20.3 | 20.1 | 14.0 | 5.6 | 0.8 |
Lymphoma | 12.3 | 2.7 | 8.9 | 10.8 | 10.2 | 14.7 | 20.3 | 17.5 | 12.0 | 2.7 |
Ewing sarcoma | 9.5 | 16.2 | 59.6 | 16.8 | 4.7 | 2.0 | 0.8 | |||
Chordoma | 6.3 | 1.1 | 4.2 | 6.2 | 13.5 | 18.5 | 24.4 | 20.5 | 9.3 | 2.2 |
Fibrosarcoma | 4.5 | 3.1 | 11.4 | 13.3 | 19.2 | 14.5 | 16.7 | 12.9 | 6.3 | 2.4 |
Chondrosarcoma, dedifferentiated | 2.1 | 1.7 | 2.5 | 6.7 | 18.3 | 32.5 | 17.5 | 16.7 | 4.2 | |
Malignant fibrous histiocytoma | 1.5 | 1.2 | 15.7 | 10.8 | 13.3 | 19.3 | 9.6 | 21.7 | 6.0 | 2.4 |
Osteosarcoma, parosteal | 1.2 | 17.4 | 39.1 | 27.5 | 10.1 | 5.8 | ||||
Chondrosarcoma, mesenchymal | 0.6 | 17.6 | 32.4 | 29.4 | 11.8 | 2.9 | 2.9 | |||
Adamantinoma | 0.6 | 2.9 | 29.4 | 44.1 | 5.9 | 5.9 | 5.9 | 5.9 |
Table 4
Age distribution of benign osseous tumors
Tumor type | Overall incidence | Incidence by decade (%) | ||||||||
---|---|---|---|---|---|---|---|---|---|---|
1 | 2 | 3 | 4 | 5 | 6 | 7 | 8 | 9+ | ||
Osteochondroma | 34.9 | 11.8 | 47.8 | 18.5 | 10.6 | 5.4 | 3.6 | 1.4 | 0.9 | |
Giant cell tumor | 22.8 | 0.5 | 15.1 | 37.0 | 24.6 | 13.3 | 6.2 | 2.5 | 0.9 | |
Chondroma (enchondroma) | 13.4 | 10.7 | 21.8 | 17.0 | 17.0 | 17.3 | 8.1 | 6.6 | 1.2 | 0.3 |
Osteoid osteoma | 13.3 | 13.6 | 51.4 | 21.8 | 9.1 | 1.5 | 0.9 | 1.2 | 0.6 | |
Chondroblastoma | 4.8 | 2.5 | 58.8 | 14.3 | 10.9 | 4.2 | 8.4 | 0.8 | ||
Hemangioma | 4.3 | 3.7 | 9.3 | 13.9 | 15.7 | 25.9 | 16.7 | 11.1 | 3.7 | |
Osteoblastoma | 3.5 | 6.9 | 41.4 | 32.2 | 10.3 | 3.4 | 3.4 | 1.1 | 1.1 | |
Chondromyxoid fibroma | 1.8 | 11.1 | 24.4 | 31.1 | 13.3 | 8.9 | 8.9 | 2.2 |
Diagnosis: Soft Tissue Tumors
Despite the superiority of MR imaging in delineating soft-tissue tumors, it remains limited in its ability to precisely characterize them, with most lesions demonstrating a nonspecific appearance [48, 49]. There are instances, however, in which a specific diagnosis may be made or strongly suspected on the basis of MR imaging features (Box 3). This is usually done on the basis of lesion signal intensity, pattern of growth, location and associated „signs” and findings. The MR imaging appearance of these lesions has been well reported, and is not reviewed here.
Box 3
Specific diagnoses that might be made or suspected on the basis of magnetic resonance imaging
Vascular lesions: | Aneurysm and pseudoaneurysm |
Arteriovenous hemangioma (AVM) | |
Glomus tumor | |
Hemangioma | |
Hemangiomatosis (angiomatosis) | |
Lymphangioma | |
Lymphangiomatosis | |
Bone and cartilage forming lesions: | Extraskeletal chondroma |
Myositis ossificans | |
Panniculitis ossificans | |
Synovial chondromatosis | |
Fibrous lesions: | Elastofibroma |
Fibroma of tendon sheath | |
Fibromatosis coli | |
Musculoaponeurotic fibromatosis | |
Superficial fibromatosis | |
Lipomatous lesions: | Lipoma |
Lipoma arborescens | |
Lipoma of tendon sheath | |
Lipomatosis | |
Lipomatosis of nerve | |
Lipoblastoma | |
Lipoblastomatosis | |
Liposarcoma | |
Periosteal lipoma | |
Synovial lipoma | |
Tumor-like lesions: | Abscess |
Calcific myonecrosis | |
Cystic adventitial disease | |
Epidermal inclusion cyst | |
Fat necrosis | |
Ganglion | |
Granuloma annulare | |
Hematoma | |
Hydroxyapatite crystal disease | |
Intramuscular myxoma | |
Myonecrosis | |
Popliteal (synovial) cyst | |
Tophus | |
Tumoral calcinosis | |
Peripheral nerve lesions: | Morton neuroma |
Neurofibroma | |
Schwannoma | |
Traumatic (stump) neuroma | |
Synovial lesions: | Giant cell tumor of tendon sheath |
Nodular synovotis | |
Pigmented villonodular synovitis | |
Synovial chondromatosis | |
Synovial sarcoma |
DeSchepper et al. [50] performed a multivariate statistical analysis of ten imaging parameters, individually and in combination. These researchers found that malignancy was predicted with the highest sensitivity when lesions had high signal intensity on T2-weighted images, were larger than 33 mm in diameter, and had heterogeneous signal intensity on T1-weighted images. The signs that had the greatest specificity for malignancy included tumor necrosis, bone or neurovascular involvement, and mean diameter of more than 66 mm. In a recent study of 548 patients by Gielen and colleagues [51], in which imaging and clinical data were available, an accuracy of 85% was reported in differentiating between benign and malignant lesions.
When a specific diagnosis is not possible, it is often useful to formulate a suitably ordered differential diagnosis on the basis of imaging features, suspected biological potential and a knowledge of tumor prevalence based on the patient age and lesion anatomic location. This can be further refined by considering clinical history and radiologic features, such as pattern of growth, signal intensity and localization (subcutaneous, intramuscular, intermuscular, etc.). The most common malignant and benign lesions, by tumor location and patient age, are shown in Tables 5 and 6.
Table 5
Distribution of common malignant soft tissue tumors by anatomic location and age
Age (years) | Hand and wrist | No (%) | Upper extremity | No (%) | Axilla and shoulder | No (%) | Foot and ankle | No (%) | Lower extremity | No (%) |
---|---|---|---|---|---|---|---|---|---|---|
0–5 | Fibrosarcoma | 5 (45)a | Fibrosarcoma | 9 (29) | Fibrosarcoma | 9 (56) | Fibrosarcoma | 5 (45) | Fibrosarcoma | 24 (45) |
Angiosarcoma | 1 (9) | Rhabdomyosarcoma | 7 (23) | Rhabdomyosarcoma | 4 (25) | DFSP | 2 (18) | Rhabdomyosarcoma | 8 (15) | |
Epithelioid sarcoma | 1 (9) | Angiomatoid MFH | 3 (10) | Angiomatoid MFH | 1 (6) | MPNST | 2 (18) | Giant cell fibroblastoma | 5 (9) | |
Malignant GCT tendon sheath | 1 (9) | DFSP | 2 (6) | Chondrosarcoma | 1 (6) | Rhabdomyosarcoma | 2 (18) | MPNST | 5 (9) | |
DFSP | 1 (9) | Giant cell fibroblastoma | 2 (6) | MPNST | 1 (6) | Angiomatoid MFH | 3 (6) | |||
MPNST | 1 (9) | MPNST | 2 (6) | DFSP | 3 (6) | |||||
Rhabdomyosarcoma | 1 (9) | MFH | 2 (6) | Angiosarcoma | 2 (4) | |||||
Other 4 | (13) | Other | 3 (6) | |||||||
6–15 | Epithelioid sarcoma | 9 (21) | Angiomatoid MFH | 30 (33) | Angiomatoid MFH | 8 (21) | Synovial sarcoma | 11 (21) | Synovial sarcoma | 28 (22) |
Angiomatoid MFH | 7 (16) | Synovial sarcoma | 14 (15) | MFH | 5 (13) | DFSP | 9 (17) | Angiomatoid MFH | 22 (17) | |
Synovial sarcoma | 5 (12) | Fibrosarcoma | 8 (9) | Ewing sarcoma | 4 (10) | Rhabdomyosarcoma | 5 (9) | MFH | 13 (10) | |
MFH | 4 (9) | MPNST | 7 (8) | MPNST | 4 (10) | Angiosarcoma | 4 (8) | Liposarcoma | 11 (9)
![]() Stay updated, free articles. Join our Telegram channel![]() Full access? Get Clinical Tree![]() ![]() ![]() |