Congenital heart disease (CHD) can be a challenge for the imagers because it requires a profound knowledge of the morphological and functional characteristics of a broad range of heart defects. Moreover, complex CHD often involves palliative or corrective surgical intervention that can significantly alter the anatomy and blood flow patterns. Therefore complete knowledge of these surgical palliations and repairs is essential to accurately identify associated problems and complications. There has been an increasing use of cardiac magnetic resonance imaging (CMR) and multidetector computed tomography (CT) in the evaluation of these patients. Hence there is an increasing interest among radiologists to understand and learn the various nomenclatures and classifications in place to describe these lesions.
Segmental Approach to Congenital Heart Disease
A standardized approach to address the countless variations in complex CHD is necessary to foster understanding of these lesions, along with promoting a common language across disciplines to optimize management of these patients. In 1972, Richard Van Praagh pioneered the segmental approach to CHD. This method involves dividing the heart into three major segments (atria, ventricles, and great arteries) and two interconnecting segments (atrioventricular [AV] junction and conus arteriosus) and then sequentially describing the spatial organization (situs) of each of these three segments using Latin terminology ( , ). The major groundwork for the segmental approach was laid by Maurice Lev, who coined the morphological method of chamber identification and described the key morphological features of various major segments. Meanwhile an alternate approach was later proposed by Robert Anderson. This involved describing the arrangement of the three major components (atria, ventricular, and arterial components) and emphasizing the two connecting segments (AV and ventriculoarterial connections) using English terminology ( Fig. 3.1 ). The connecting segments can be designated as being either concordant or discordant ( ; ).
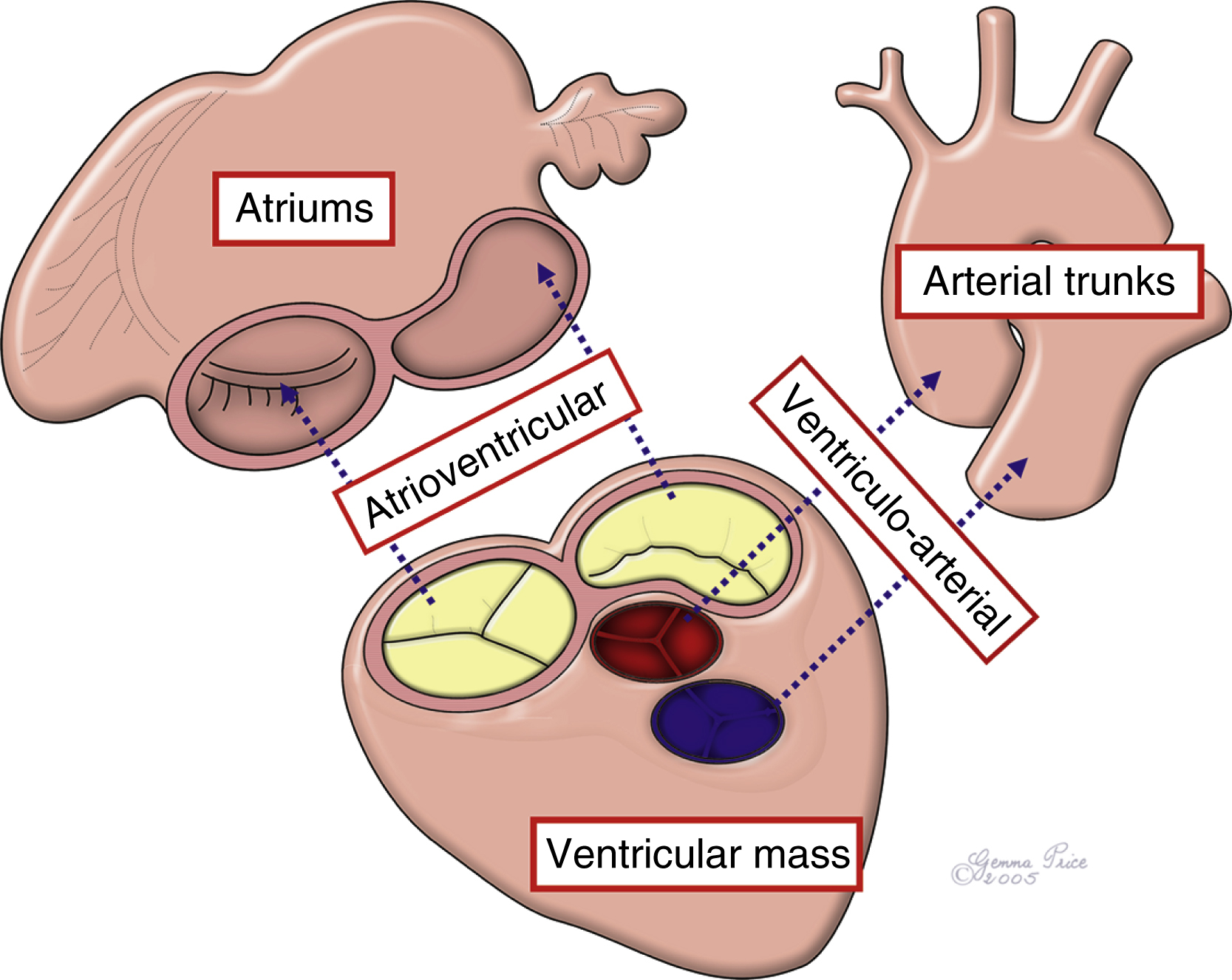
The sequential segmental approach aims to address the following:
- 1.
Perform morphological identification of the three major cardiac segments (atria, ventricles, great arteries).
- 2.
Describe the relationship of the interconnecting segments (AV and ventriculoarterial connections).
- 3.
Describe any associated anomalies involving the valves, atrial and ventricular septum, the great vessels, and the systemic and pulmonary veins.
For example, using Anderson terminology, one would describe the normal heart as having usual atrial arrangement with concordant AV and ventriculoarterial connections and right hand ventricular topology.
The approach taken by Van Praagh uses a three-letter notation within braces to describe the relationships of the three segments, mentioning the connections only if abnormal, with additional notations before or after to describe important associated cardiac anomalies. The spatial relationship of the three main cardiac segments is indicated within the braces: {visceroatrial situs, ventricular situs or looping, great arterial relationship}, with the normal cardiac arrangement stated as {S, D, S}, indicating normal visceroatrial situs solitus, D-looped ventricles, and arterial situs solitus (normally related great arteries). Any ventriculoarterial misalignment, such as transposition of the great arteries (TGA) or double-outlet right ventricle (DORV), may be notated before the braces. Any AV malalignments and associated cardiovascular malformations may be notated after the braces, such as ventricular septal defects (VSDs) or hypoplastic right ventricle (RV). The cardiac position within the chest or position of the cardiac apex may be notated before the braces if abnormal. For instance, dextroposition refers to the situation when the heart is predominantly positioned within the right hemithorax, and mesoposition when positioned predominantly in the center of the thorax. Dextrocardia refers to the situation where the cardiac apex points rightward, and mesocardia when midline ( ; ). Although there are differences to both the Van Praagh and Anderson approaches, there are many similarities to their approaches. Important to this, a recent consensus document of terminology related to the nomenclature of CHD was published by the International Society for Nomenclature of Paediatric and Congenital Heart Disease for the 11th iteration of the International Classification of Diseases (ICD-11; refer to this document and to the ICD-11 listing on the World Health Organization website [ https://icd.who.int/dev11/f/en ] for a detailed listing) ( ).
In addition, it is important to recognize that historical cardiac terminology named the attitudinal description with the heart removed from the body and placed on its apex in the Valentine position. This becomes confusing to the cardiac imager who now views the heart as it lies within the body. A review by describes the detailed normal cardiac anatomy in attitudinally correct fashion as it lies within the body, as revealed by CT three-dimensional reconstructions.
Morphological Identification of the Major Cardiac Segments
Recognizing important morphological characteristics of the various cardiac chambers and great arteries is important in classifying the segmental anatomy, because the description of an atrium or ventricle as being “right” or “left” does not refer to its spatial orientation but its morphological identification. Although some of these features are not readily identifiable by current modes of cardiac imaging, with improving technology, most of these features can be distinguished. The morphological identification of these chambers and great arteries is then used to assess their respective connections, which help systematically describe the cardiac morphology.
Atrial Identification
The atria are readily distinguished by assessing the inferior vena cava (IVC) and coronary sinus connection, the relation of the primary atrial septum to its “secondary septum,” and the atrial appendage morphology, including the extent of pectinate muscle. The right atrium (RA), or systemic venous atrium, receives systemic venous return from the IVC and coronary sinus drainage. Even in the setting of an interrupted IVC, a suprahepatic segment of the IVC tends to drain into the RA, making this a more reliable feature. The RA appendage is broad and triangular shaped, with pectinate muscle extending from the atrial appendage to the vestibule of the RA ( Fig. 3.2A ). Although the pectinate muscle is difficult to assess by current echocardiographic imaging, it is increasingly detected by CMR and CT imaging.
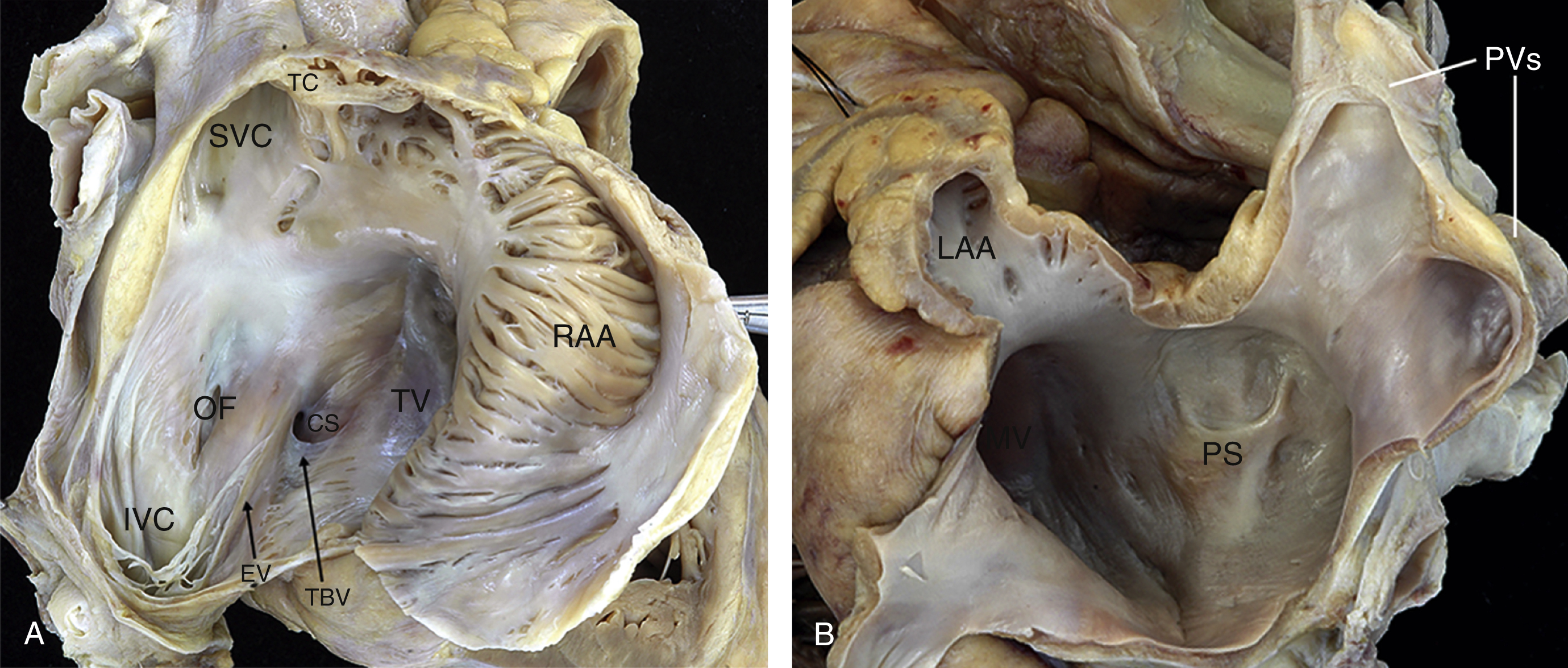
The left atrium (LA) normally receives all of the pulmonary venous return, although this is highly variable and an unreliable marker for morphological identification. The flap valve of the foramen ovale attaches to the left atrial side of the “secondary septum,” the latter of which is technically not a true septum but instead an interatrial infolding that encircles the inferior, posterior, and superior portions of the oval fossa. More reliable is the assessment of the left atrial appendage, which appears long, thin, and “finger-like,” with the pectinate muscle contained within the appendage (see Fig. 3.2B ).
Visceroatrial Arrangement or Situs
When we assess atrial arrangement or situs, we are assessing the visceral relationship to the respective atrium, or visceroatrial situs. Usual visceral and atrial arrangement or visceroatrial situs solitus {S,-,-} refers to the normal visceral arrangement (right-sided liver, single left-sided spleen, three-lobed right lung with an eparterial bronchus, and two-lobed left lung with a hyparterial bronchus) with a right-sided morphological RA. Mirror image visceroatrial arrangement or visceroatrial situs inversus refers to the mirror image of the normal arrangement described earlier and is denoted as {I,-,-}. The stomach, superior vena cava (SVC), pulmonary venous connections, and cardiac apex position are not reliable markers for determining visceroatrial situs. Visceroatrial situs ambiguous {A,-,-} is used to denote when the atria cannot be morphologically distinguished as right and left, as is commonly the case in heterotaxy syndromes and common atrium ( Fig. 3.3 ). Using Andersonian terminology in a patient with heterotaxy syndrome, one would describe the patient as having right or left atrial appendage isomerism, and the details of the visceral arrangement would be described ( Figs. 3.3 and 3.4 ).
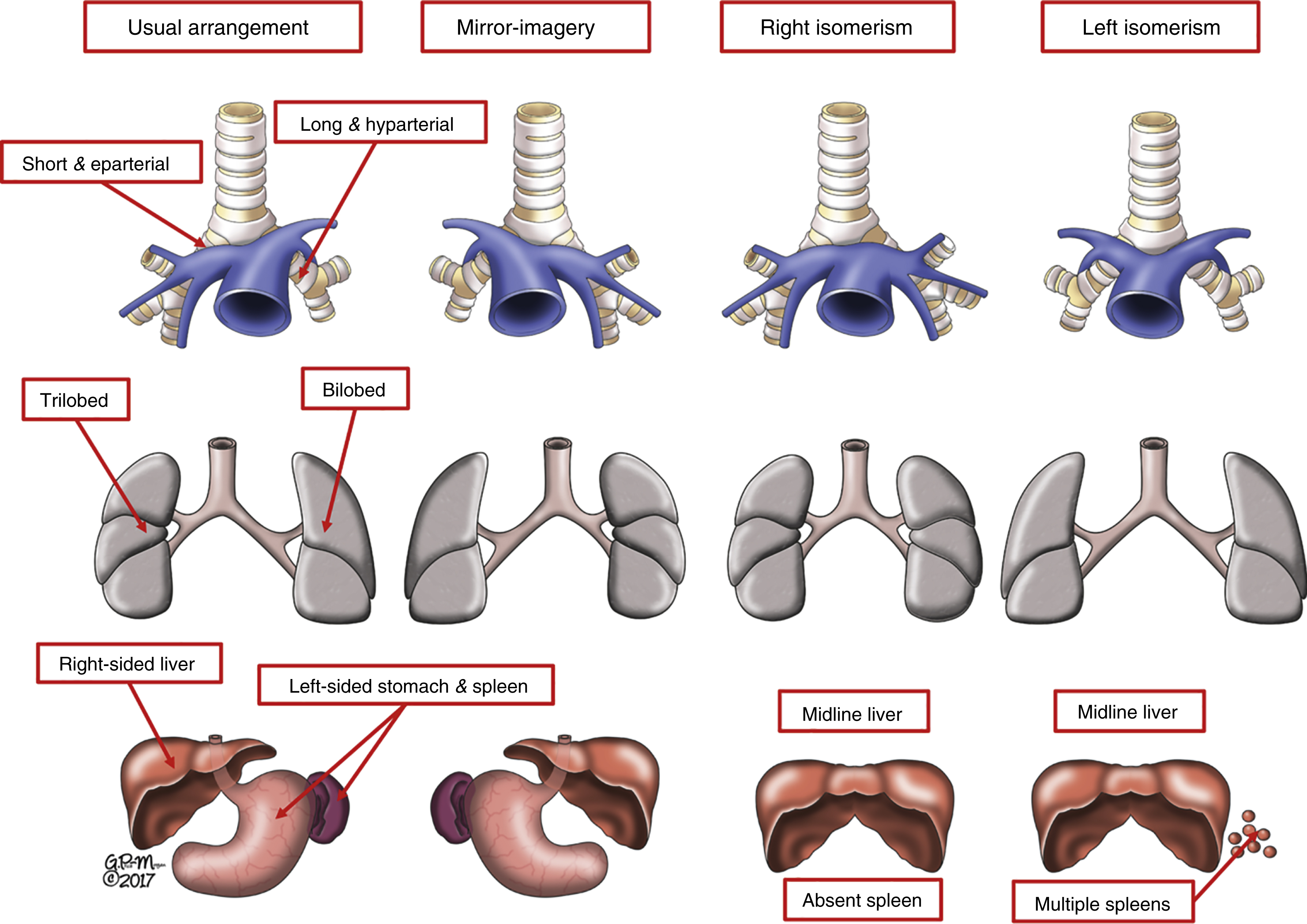
Ventricular Identification
The ventricles are distinguished by assessment of the corresponding AV valve, including valve attachments, the ventricular shape, and the extent and appearance of the muscular trabeculations within the ventricle. By definition, the tricuspid valve (TV) is associated with the morphological RV. The TV is typically trileaflet, composed of the septal, anterosuperior, and inferior leaflets. It contains prominent direct chordal attachments of its septal leaflet to the ventricular septum (referred to as “septophilic”) and tends to be more apically positioned relative to the mitral valve (MV). Papillary muscles arise from both the septal and free wall surfaces of the RV. The RV is triangular or pyramidal, and is filled with coarse, heavy trabeculations with a prominent muscular band (“moderator band”) coursing from the apical portion of the ventricular septum to the free wall, containing the right bundle branch of the conduction system. In the normal heart, the outlet portion of the RV contains a prominent muscular cone of tissue separating the TV from the pulmonary valve, commonly referred to as the conus or infundibulum. This is highly variable in abnormal cardiac arrangements and cannot be used to identify the RV ( Fig. 3.5A ).
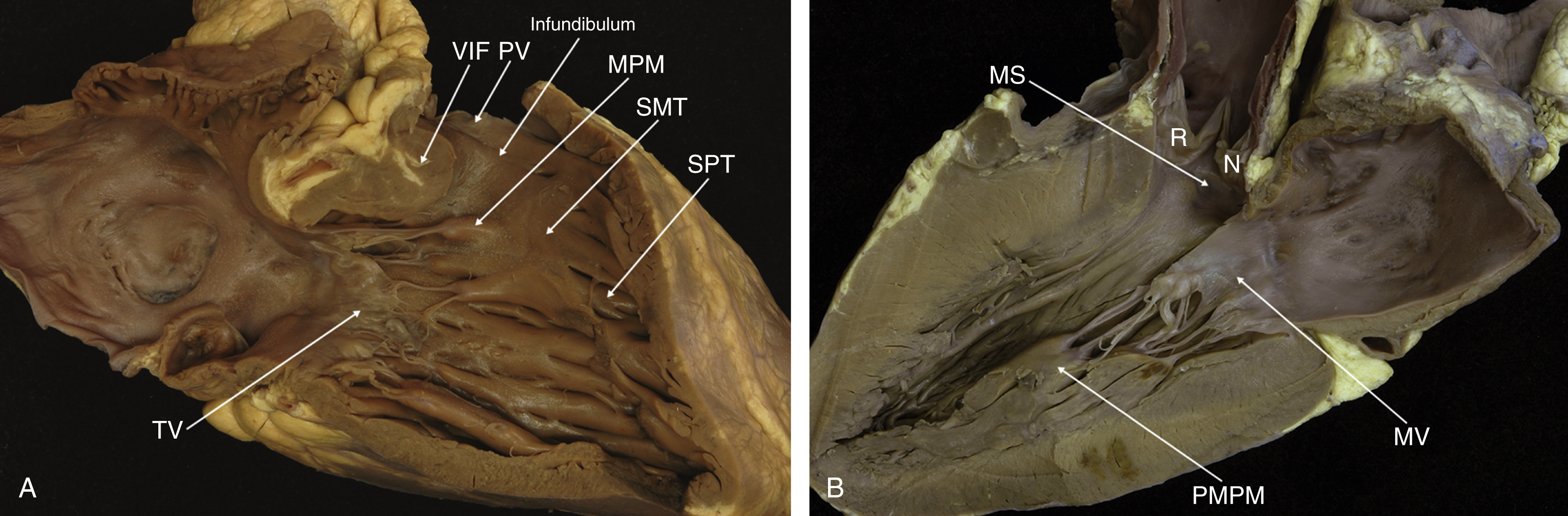
The left ventricle (LV) is associated with the MV, which is commonly composed of two leaflets, the anterior (“aortic”) and posterior (“mural”) leaflets. These two leaflets are attached by chordae to the two free wall papillary muscles, the anterolateral and posteromedial papillary muscles, which when related to the body are in fact positioned superolaterally and inferomedially, respectively. Given the lack of septal attachments of the MV, it is commonly referred to as “septophobic.” The MV is more cranially positioned compared with its adjacent AV valve. The LV is prolate ellipsoid or bullet shaped, with fine trabeculations within the apical trabecular component of the ventricle and a smooth septal surface (see Fig. 3.5B ). It should be noted that the shape of the ventricle can be an unreliable marker in both pressure- and/or volume-loaded ventricles, which is present in many cardiac defects. In the normal heart, there commonly is no conus or infundibulum present in the LV, resulting in mitral–aortic valve fibrous continuity. Again this is an unreliable marker in CHD.
Ventricular Topology or Looping
In early embryogenesis, the straight heart tube outgrows its containing sac resulting in looping of the tube either to the right (D-dextro-looped) as in normal development, or to the left (L-levo-looped). The “hand rule” is used to determine the topology or looping of any developed heart. The palm of one hand is placed on the ventricular septal surface of the RV with the thumb in the inflow and the fingers in the outflow. This can only be accomplished with one hand. If the right hand is used, then the ventricles are said to have right hand ventricular topology or to be D-looped ventricles {-,D,-}, as seen in normal development. In this arrangement the RV is anterior and to the right of the LV ( Fig. 3.6A ). If the left hand is used, then the ventricles are said to have left hand ventricular topology or to be L-looped ventricles {-,L,-}. In this arrangement the RV is anterior and to the left of the LV (see Fig. 3.6B ). X is used to denote when the ventricular morphology cannot be determined {-,X,-}, or it is referred to as indeterminate.
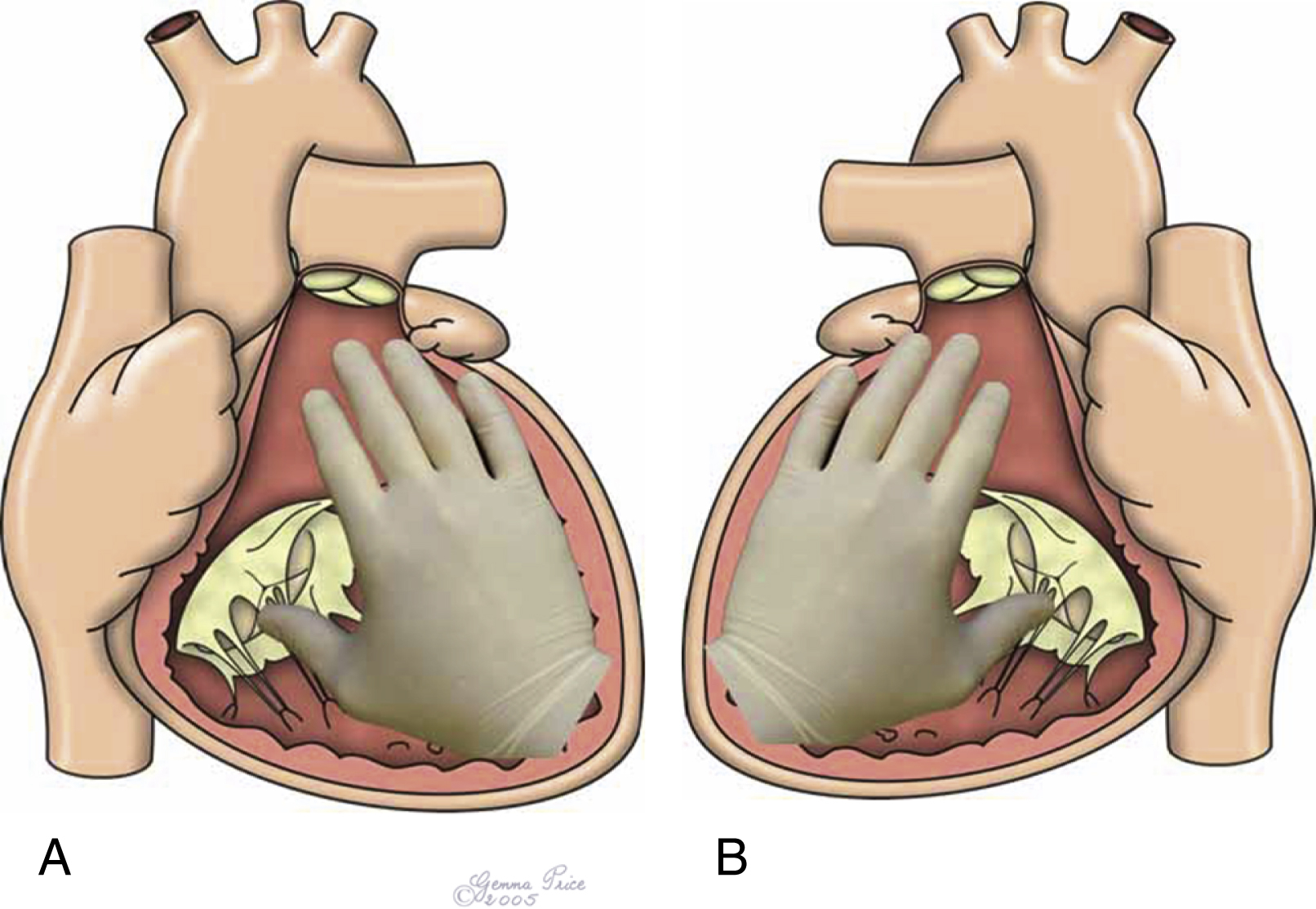
Great Arterial Identification
The artery that gives rise to the branch pulmonary arteries is the pulmonary trunk or main pulmonary artery. The aorta usually gives rise to the coronary arteries, the aortic arch, and its brachiocephalic arteries. A truncus arteriosus, or common arterial trunk, occurs when a common vessel arises from the ventricles and gives rise to the coronary, brachiocephalic, and branch pulmonary arteries. A solitary arterial trunk is defined when there is absence of the intrapericardial pulmonary arteries, and one cannot determine, had they been present, whether the pulmonary trunk and its branches would have originated separately from a ventricle or from a common arterial trunk ( Fig. 3.7 ). As described previously, the conus or infundibulum separates the TV from the pulmonary valve in the normal heart. This is absent in the LV, allowing mitral–aortic valve fibrous continuity. All hearts can have one of the following: bilateral infundibulum (subaortic and subpulmonary), subpulmonary infundibulum (as in the normal heart), subaortic infundibulum only (as usually seen in transposition), or bilaterally absent infundibulum (as can be seen in double-outlet LV). This is highly variable and should be noted on morphological assessment.
Great Arterial Arrangement or Situs
In normally related great arteries (NRGAs) the aorta is aligned with the LV and the pulmonary trunk is aligned with the RV. The subpulmonary infundibulum lifts the pulmonary root superiorly and leftward in relationship to the aortic root, and mitral–aortic valve fibrous continuity is commonly present. In the normal heart (usual atrial arrangement with concordant AV and ventricular arterial connections with NRGAs, or {S,D,S}), the aortic root lies posterior and to the right of the pulmonary root. In I-inversus-NRGAs in a patient with situs inversus of the atria {I,L,I} the aortic root lies posterior and to the left of the pulmonary root. All other great artery relationships are abnormal, with variations in underlying infundibulum. The denotation for abnormal great artery relationships, which is labeled in the third position in the segmental diagnosis, includes L, D, and A, describing the relationship of the aortic valve to the pulmonary valve as being leftward, rightward, or anterior, respectively. Andersonian terminology would simply describe the spatial relationship. Describing this detail is important to fully understand the given morphology. For example, the denotation {-,-,D} could describe an aortic root that is either rightward or rightward and anterior to the pulmonary root.
Assessment of the Cardiac Segment Connections
After identifying the morphology of the three major cardiac segments and their spatial arrangement, the intersegmental connections must be assessed. Further mention of the cardiac chambers in this chapter will reference the morphological identification rather than the spatial arrangement. Normal or concordant connections conclude that the RA is connected to the RV, which is in turn aligned to the pulmonary trunk. Similarly, the LA is connected to the LV, which is aligned to the aorta. Abnormal connections may occur at both the AV connection and the ventriculoarterial connection.
Atrioventricular Connections
In biventricular AV connections, commonly they may be either concordant, in which the RA drains into the RV and LA drains into the LV ({S,D,-} or {I,L,-}), or discordant, in which the RA drains into the LV and the LA drains into the RV ({S,L,-} or {I,D,-}) ( Fig. 3.8 ). Less commonly, abnormal AV connections include straddling of the AV valve in which there are chordal attachments crossing over to the opposite ventricle through an interventricular communication, overriding of the AV valve in which the AV valve annulus crosses the interventricular septum lying partially over the opposite ventricle, and a balanced common atrioventricular septal defect (AVSD). Univentricular AV connections can include an atretic AV valve, double inlet LV, or less commonly, RV, and left or right dominant unbalanced common AVSD. In heterotaxy syndrome, whether right or left atrial appendage isomerism, there are mixed AV connections. Any abnormal AV connection should be noted after the braces when describing a lesion using the Van Praagh terminology.
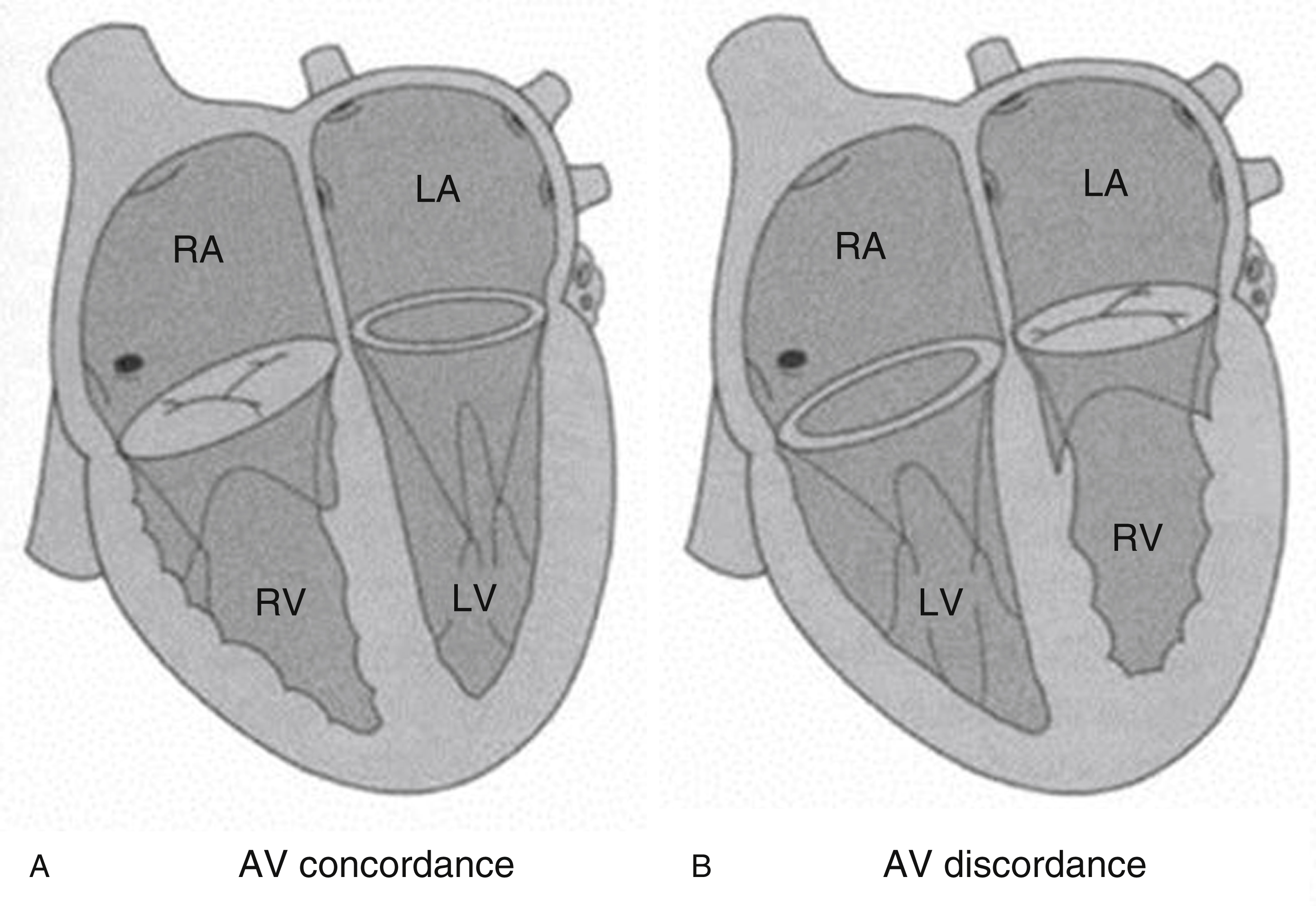
Ventriculoarterial Connections
Normally the pulmonary trunk is aligned with the RV and the aorta with the LV (concordant ventriculoarterial connections), with the presence of subpulmonary infundibulum, and the absence of subaortic infundibulum. The variation in subarterial infundibulum generally determines the relative position of the great arteries, because the presence of the subarterial infundibulum tends to position the respective great artery superiorly and anteriorly. Any other scenario apart from that in NRGAs or anatomically corrected malposition (better described as concordant ventriculoarterial connections with bilateral subarterial infundibula and parallel arterial trunks) comprises discordant ventriculoarterial connections. In TGA the aorta is aligned with the RV and the pulmonary trunk with the LV. DORV often, but not always, occurs when there are varying degrees of infundibulum under both great arteries, resulting in both great arteries arising from the RV. This is always associated with a VSD to act as an outflow for the LV. Common arterial trunk or truncus arteriosus, and the rare cases of a solitary arterial trunk with absence of intrapericardial pulmonary arteries, were both previously described. When using the Van Praagh approach, any ventriculoarterial connection (the “conotruncal” diagnosis) should be noted before the braces when describing a lesion.
Other Associated Lesions
When using the Van Praagh approach, following the “conotruncal” diagnosis, segmental diagnosis, and any associated AV alignment abnormality, any other associated defect should be listed. These include but are not limited to VSDs, atrial septal defects (ASDs), semilunar valve abnormalities, aortic arch abnormalities, and patent ductus arteriosus (PDA). When using the Anderson approach, applicable lesions are listed sequentially as they relate to the three major segments and two connecting segments, with septal defects often stated last ( ; ; ; ).
Septal Defects
Atrial Septal Defects and Interatrial Communications
Interatrial communications makes up to 10% of CHDs in children, with an estimated incidence of 100 per 100,000 live births. The most common of these is a deficiency in the floor of the oval fossa, referred to as an oval fossa defect or secundum ASD. The only other form of a true deficiency in the atrial septum is a deficiency found in the anteroinferior muscular buttress, the so-called vestibular defect ( ; ). This underappreciated defect is often mistaken for a secundum ASD; however, careful interrogation will demonstrate that it is not found within the confines of the floor of the oval fossa. The remaining interatrial communications are not related to a deficiency in the atrial septum and allow interatrial shunting. These include the sinus venosus defects, which are often found at the mouth of one of the caval veins and are related to a retained venovenous bridge with associated anomalous drainage of a pulmonary vein, and the coronary sinus defect, which is caused by the absence of the walls that usually separate the coronary sinus from the LA. A patent foramen ovale (PFO), which is a normal interatrial communication present in the fetus, remains patent into adulthood in approximately 25% to 30% of healthy adults and is considered a normal variant. The “primum ASD” is not technically an ASD, but instead an AVSD with exclusively atrial-level shunting. In this form of an AVSD the common AV junction is often divided into a right and left AV valve by a tongue of tissue between the superior and inferior bridging leaflets, which is firmly attached to the crest of the ventricular septum. This defect will be discussed in more detail in the Atrioventricular Septal Defects section ( ; ).
Morphology and Pathophysiology
The most common type of ASD, a secundum ASD, results from a deficiency in the primary atrial septum, which normally forms the floor of the oval fossa. The vestibular defect results from a deficiency in the anteroinferior muscular buttress. The sinus venosus defect results from a retained venovenous bridge between the respective systemic and pulmonary veins, allowing indirect communication from the LA to the RA. The coronary sinus defect results from deficiency in the walls that usually separate the coronary sinus from the LA, allowing indirect communication from the LA to the RA. This defect is commonly associated with a persistent left SVC draining into the coronary sinus.
Irrespective of the type of interatrial communication, the degree of left-to-right shunting is determined by the size of the defect and relative compliance of the ventricles. During the first few months of life, RV compliance improves, resulting in increasing right-sided volume overloading. Into adulthood, LV compliance tends to worsen, with further increase in right-sided volume overloading. This left-to-right shunting leads to RA, RV, and pulmonary arterial dilation with pulmonary overcirculation, which eventually may lead to pulmonary artery hypertension and pulmonary vascular obstructive disease. In severe pulmonary vascular obstructive disease, reversal of atrial level shunting, or Eisenmenger syndrome, may occur. It should be noted that smaller secundum ASDs (≤5–8 mm) may become smaller or even close spontaneously in the first few years of life, but thereafter tend to become larger with time ( ).
Clinical Presentation
The majority of children tolerate this initial left-to-right shunting well and remain asymptomatic into early adulthood, with only up to 5% of patients developing significant pulmonary vascular disease leading to pulmonary hypertension before 20 years of age. However, the incidence may increase fourfold in the third and fourth decades of life. The majority of children with an interatrial communication are diagnosed after evaluation for a murmur or incidentally after evaluation with electrocardiogram, chest x-ray (CXR), or echocardiogram. Adults may present with exercise intolerance, atrial arrhythmias, or paradoxical emboli. If Eisenmenger syndrome develops, patients may present with cyanosis or syncope with exertion.
Imaging
Larger interatrial communications are readily detected in utero with fetal echocardiography; however, small-to-moderate secundum ASDs can be difficult to distinguish in utero from a normal foramen ovale. CXR may be normal during the first decade of life or in smaller interatrial communications. Moderate-to-severe shunting across an interatrial communication can lead to cardiomegaly on CXR as a result of RA and RV enlargement, with prominent main pulmonary artery and increased pulmonary vascular markings extending to the periphery.
Echocardiography is crucial in defining the interatrial communication type, size, degree of shunting, the presence of right chamber dilation, estimation of RV pressure, and any associated defects. Subcostal and high-right parasternal imaging are the primary images used to assess the atrial septum. Transesophageal echocardiogram (TEE) is often used perioperatively to better define the interatrial communication, especially in patients with poor acoustic windows during transthoracic echocardiogram (TTE). TEE additionally plays a crucial role in guiding percutaneous device closure of secundum ASDs. A PFO is differentiated from a small secundum ASD by the overlap of a flap valve on the left side and “secondary septum” or interatrial fold to the right of the interatrial septum, creating a tunneling communication. Intravenous (IV) contrast injection using agitated saline can be used during TTE or TEE imaging to confirm the presence of a PFO or ASD. Real-time three-dimensional echocardiography allows better morphological delineation of the secundum ASD and its surrounding structures, providing helpful information to determine candidacy for percutaneous device closure ( ).
CMR can be used in patients for whom echocardiography has failed to fully define the interatrial communication ( Fig. 3.9 ) and to better quantify the RV dilation and estimate the left-to-right shunt. To calculate the ASD shunting, the RV stroke volume provides an estimate for Qp (flow to the lungs that includes the shunt at the atrial level), while the LV stroke volume provides an estimate of Qs (systemic flow to the body). The difference in the ventricular stroke volume (RV − LV) in the absence of any valvar regurgitation provides an estimate of shunt volume. CMR phase contrast imaging also can be used to quantify left-to-right shunting by directly estimating Qp (main pulmonary artery flow) as opposed to Qs (ascending aorta flow). It is particularly useful in sinus venosus ( Fig. 3.10 ) and coronary sinus defects, where definition by echocardiogram can be difficult. CT and CMR is often preferred to assure accurate definition of the anomalous pulmonary venous drainage into the SVC or IVC that is commonly associated with sinus venosus defects ( Fig. 3.11 ). In particular, an accurate delineation of anomalous pulmonary veins to the SVC, including the distance from the SVC–RA junction, is critical in surgical decision-making, with the options being direct baffling of the pulmonary veins to LA (pulmonary veins draining in close proximity to SVC–RA junction) as opposed to the Warden procedure (high drainage of pulmonary veins into the cranial SVC), which involves using the caudal SVC as a pulmonary vein baffle and the cranial SVC is surgically connected to the RA appendage. Echocardiography is often adequate when interrogating these structures in the proper imaging planes ( ).
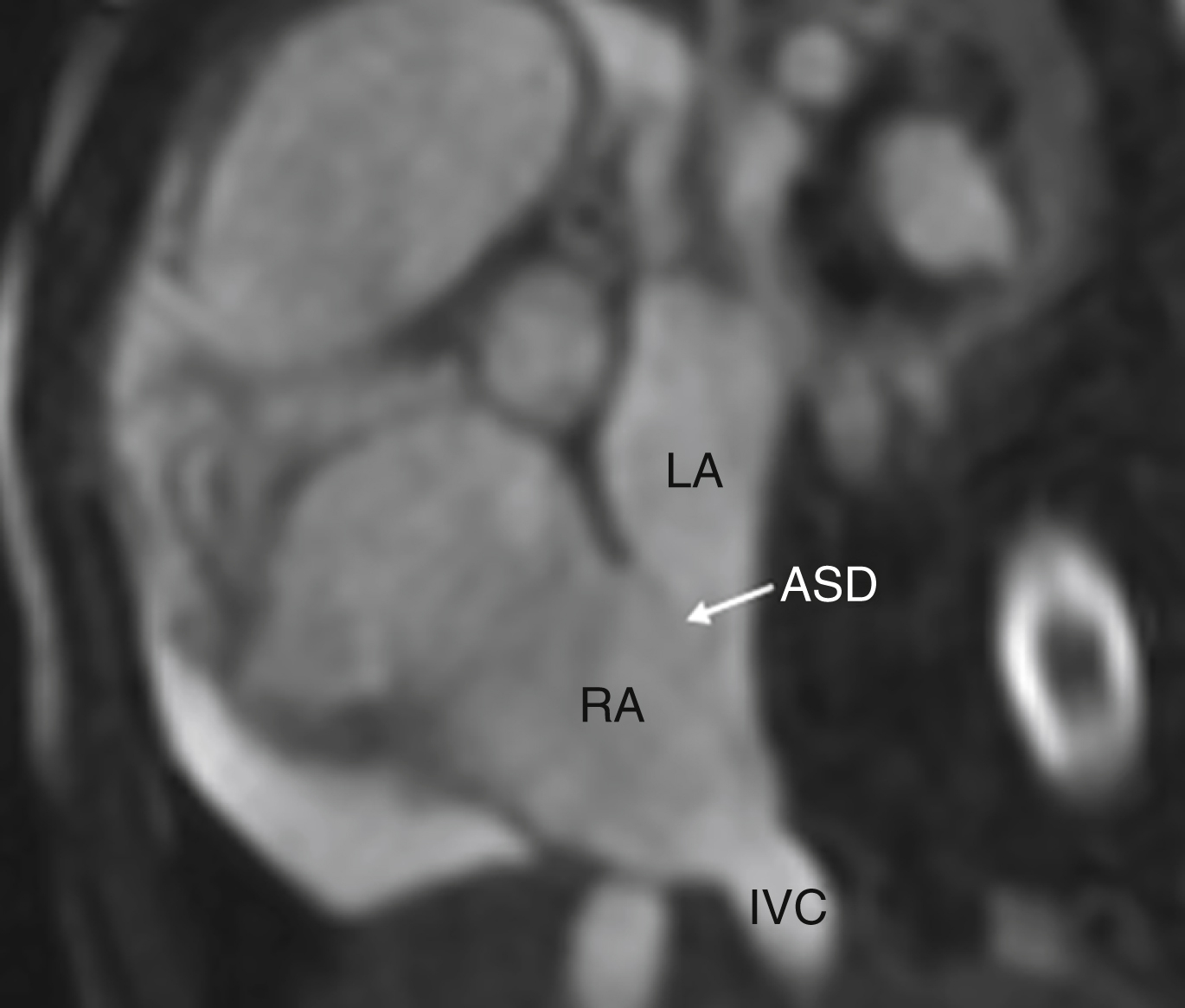
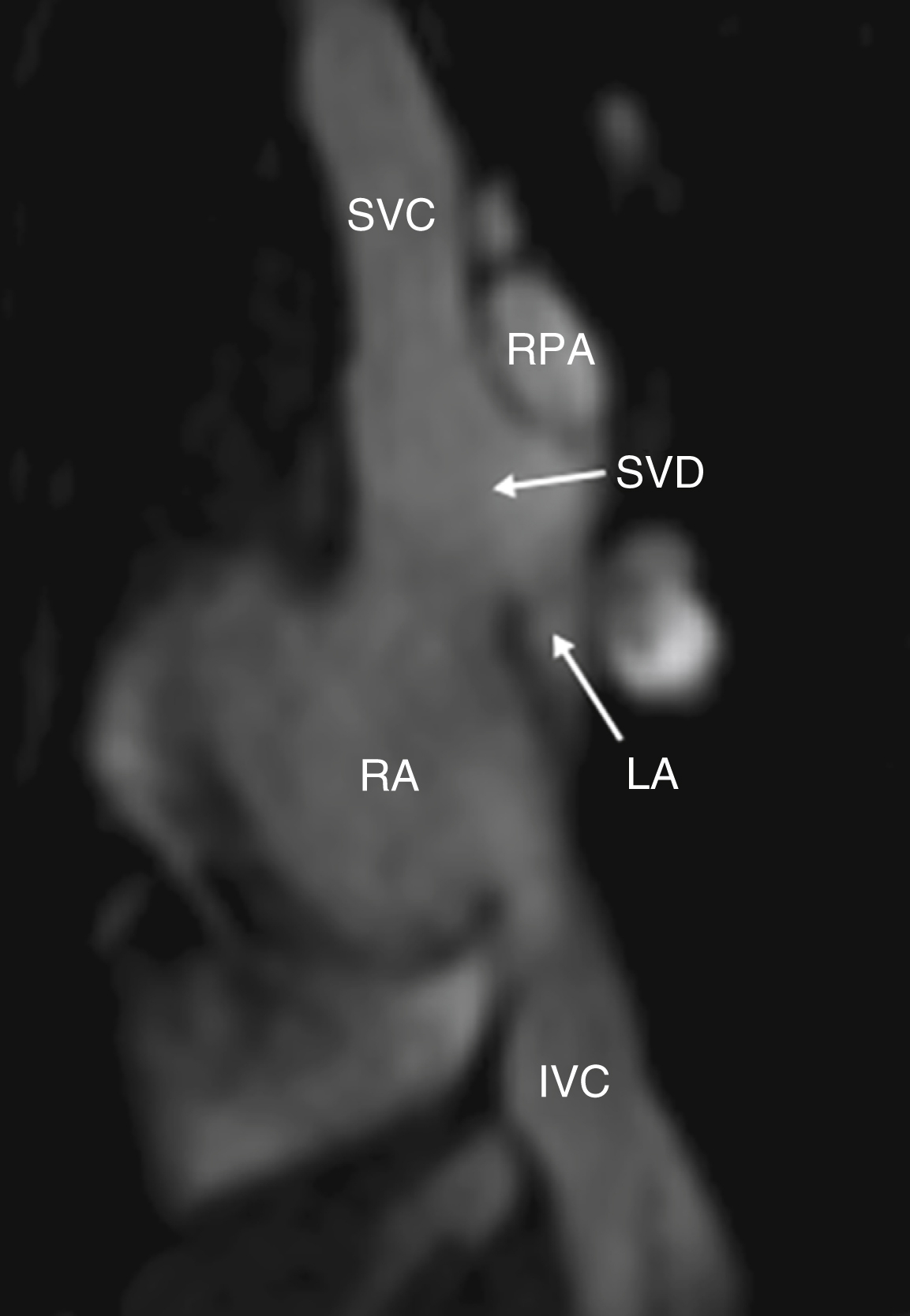
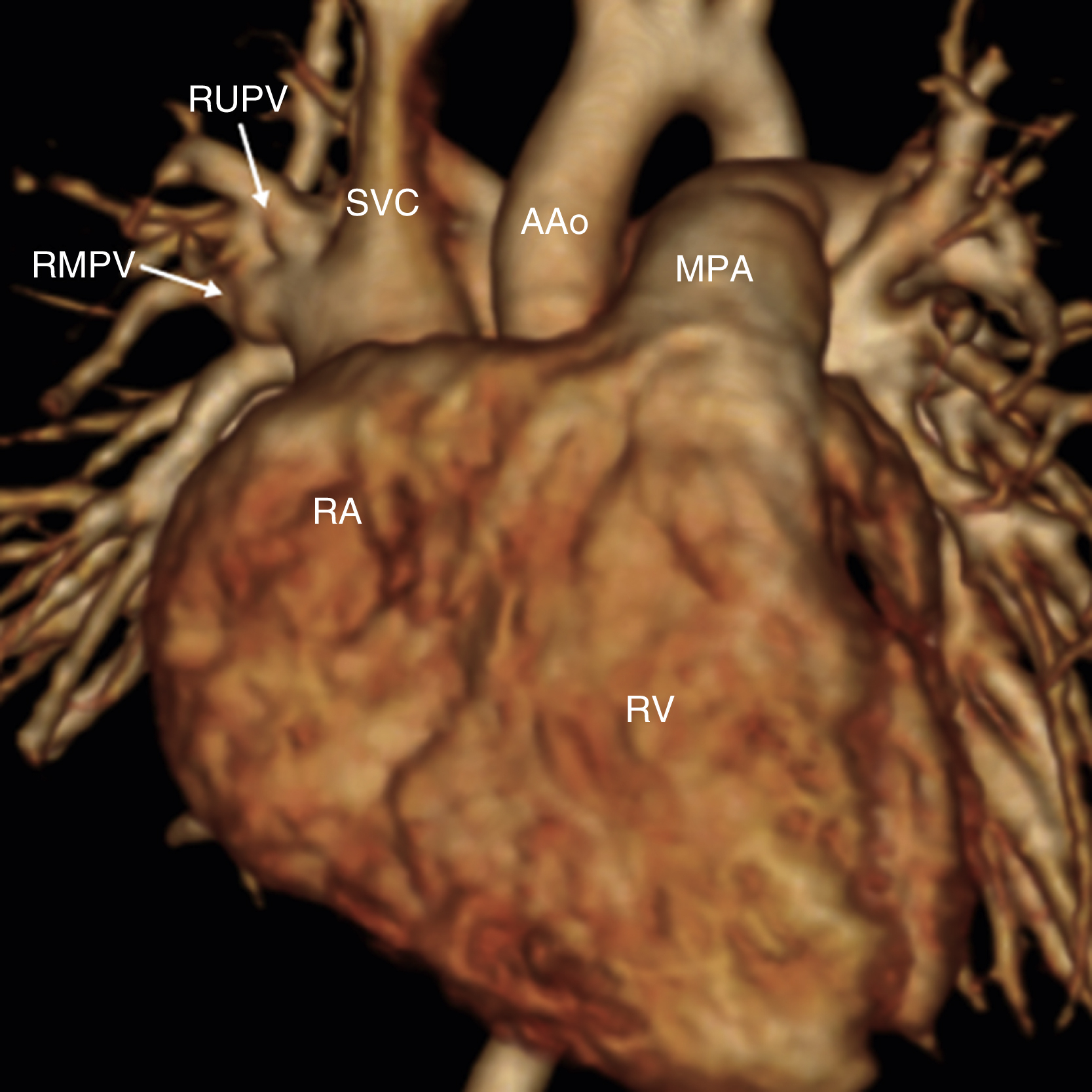
Treatment
Closure of an ASD is indicated if there is a large shunt, with Qp (pulmonary flow):Qs (systemic flow) greater than or equal to 1.5 or other evidence of right chamber enlargement. In asymptomatic children with a large shunt, elective closure is often performed between 2 and 5 years of age. In the secundum ASD, closure can be done surgically, or percutaneously with device closure if the rims surrounding the defect are deemed adequate to support a device. Closure is indicated in these scenarios to prevent long-term complications of atrial arrhythmias, paradoxical embolism, pulmonary hypertension, and severe RV dilation and dysfunction. Surgical closure is the only option for sinus venosus and coronary sinus defects. Anticongestive therapy can be initiated in symptomatic patients until closure is performed. As noted previously, smaller secundum ASDs may close spontaneously or at least get smaller in the first few years of life ( ; ).
Ventricular Septal Defects
VSDs makes up 20% to 30% of CHDs, with an estimated incidence of 5 to 50 per 1000 live births. The spontaneous closure rate of VSDs is approximately 30% ( ; ). Although the VSD is the second most common form of CHD, its description has become confusing and contentious, complicating its understanding. We support the newly proposed ICD-11 terminology, which describes both the borders of the defect (perimembranous, muscular, or doubly committed) and the geography related to the RV aspect of the ventricular septum (inlet, apical trabecular, central, and outlet). Malalignment between the atrial and ventricular septa or of the outlet septum is then additionally described when present. The reader is referred to the International Society for Nomenclature of Paediatric and Congenital Heart Disease consensus document specific to the description of VSDs for more details ).
Morphology and Pathophysiology
Description of the VSD must include both the borders of the defect and the geography related to the RV. It is our preference to begin the description with the borders because this is more objectively defined and relates the margins of the defects to the conduction system. In addition, these three defects account for the three components of septation of the ventricles during development ( ). The borders of the defect can be perimembranous, muscular, or doubly committed ( Fig. 3.12 ). Note that a defect that is doubly committed can occasionally extend to also become perimembranous. The defects can then be subclassified based on their geography related to the RV aspect of the ventricular septum. The RV geography of the defect can be inlet, apical trabecular, central, or outlet. Note that it is erroneous to think of inlet, apical, and outlet components of the septum because a large portion of the inlet of the RV is septated from the outlet of the LV. In addition, a large portion of the outlet of the RV is lifted from the base of the heart by the infundibulum, with its medial wall separating the RV from the pericardial space. It then is important to describe the presence or absence of malalignment of the atrial and ventricular septa, or malalignment of the outlet septum associated with obstruction of either outflow tract.
The perimembranous VSD relates to deficiency in the interventricular portion of the membranous septum and often involves some degree of deficiency of the surrounding muscle. This defect is defined by a fibrous posteroinferior border to the defect. This always involves fibrous continuity between the AV valves and commonly also includes fibrous continuity between the aortic and TV. When small, these defects are located centrally adjacent to the membranous septum. When medium to large, they often extend to open to the inlet and/or outlet of the RV. In the perimembranous inlet VSD, rarely there is malalignment between the atrial and ventricular septa with resulting straddling and override of the TV. This is important to identify when present because the AV node is anomalously displaced posterior and inferiorly. This has historically been mistaken as a “VSD of the canal type”; however, this does not represent an AVSD, and there are separate right and left AV junctions with a bifoliate MV. In the perimembranous outlet VSD, there is often malalignment of the outlet septum either anteriorly or posteriorly, creating some degree of RV or LV outflow tract (LVOT) obstruction, respectively.
The muscular VSD results from incomplete coalescence of the trabeculations resulting in deficiency within the muscular septum. As the name implies, the borders of the muscular VSD are completely muscular. These defects may open to the inlet, apical trabecular, and outlet components of the RV. The muscular inlet defect is important to distinguish from the perimembranous inlet defect, because the conduction system courses anterior and remote to the margins in the former, and posteroinferior and adjacent to the margins in the latter. Therefore just describing it as an “inlet VSD” is insufficient. Likewise it is important to distinguish the muscular outlet defect from the perimembranous outlet defect because the conduction system courses adjacent to the posteroinferior border in the perimembranous form but is protected by the muscular posterocaudal limb of the septomarginal trabeculation along this same border in the muscular form. The muscular outlet VSD also can have malalignment of the outlet septum. Similarly, just calling the defect an “anterior malalignment” or “posterior malalignment VSD” is insufficient, and the borders of the defect must be described. The muscular defects opening to the apical trabecular portion of the RV can be further specified as apical, midventricular, or anterior.
The doubly committed VSD results from failure of development of the proximal outflow cushions and failure of their fusion with the crest of the muscular ventricular septum. Because of the failure of normal formation of the infundibulum and outlet septum, there is fibrous continuity between the aortic and pulmonary valves. This defect can occasionally also extend to become perimembranous when there is deficiency in the posterocaudal limb of the septomarginal trabeculation along the fibrous continuity described earlier for the perimembranous VSD. The relationship to the conduction system can therefore be similar to what was described in the muscular outlet VSD when the borders are only doubly committed, or as described in the perimembranous VSD when the defect additionally extends to become perimembranous. The doubly committed VSD opens to the outlet of the RV by default, and so the geography of the defect can be inferred. Occasionally there is a fibrous remnant of the outlet septum that can be malaligned anteriorly or posteriorly ( ).
Perimembranous VSDs are the most common, accounting for 80% of VSDs. Doubly committed VSDs, occur in 5% to 7% of VSD, more commonly in those of Asian descent. Both perimembranous and doubly committed VSDs pose risk to the development of aortic valvar regurgitation related to aortic leaflet prolapse through the defect. The perimembranous defect is also associated with risk for development of double-chambered right ventricular outflow tract (RVOT) and LVOT obstruction. Muscular VSDs occur in 5% to 20% of VSDs.
Although much of this section describes the morphology of the defect, outside of the presence of malalignment of the outlet septum, none of these features affect the hemodynamics and clinical presentation. The degree of left-to-right shunting, and hence clinical manifestations, is dependent on the size of the VSD and the relative pulmonary and systemic vascular resistances. The size of the VSD is commonly compared with the aortic valvar diameter (small is <33% aortic valve, moderate is >33% but <50% the aortic valve, and large is >50% the aortic valvar diameter). As the pulmonary vascular resistance decreases over the first few weeks of life, the amount of left-to-right shunting across the defect will increase, and in larger, unrestrictive defects, florid heart failure can ensue within the first few months of life. Pulmonary overcirculation will then lead to increased pulmonary venous return and LA and LV dilation. As pulmonary vascular disease and left atrial hypertension develop, pulmonary vascular resistance increases, leading to a reversal of ventricular level shunting, resulting in Eisenmenger syndrome. Smaller defects restrict flow, preventing equalization of ventricular pressures.
Clinical Presentation
The degree of pulmonary overcirculation dictates the timing and extent of symptoms. Patients with moderate-to-larger VSDs that allow significant shunting leading to pulmonary and subsequently left heart overcirculation can present with symptoms of heart failure within the first few weeks to months of life. These signs and symptoms include failure to thrive, poor feeding, diaphoresis with feeds, tachypnea, tachycardia, and hepatomegaly. The majority of infants are diagnosed after evaluation for a murmur and/or evaluation for signs and symptoms of heart failure. Again, if Eisenmenger syndrome develops, patients may present with cyanosis or syncope with exertion.
Imaging
Larger VSDs are readily detected in utero by fetal echocardiogram. In moderate-to-large VSDs, CXR may show cardiomegaly and a dilated pulmonary trunk with increased pulmonary vascular markings extending peripherally. CXR may also show upward deviation of the left main bronchus secondary to LA dilation.
Echocardiography is important for defining the location and size of the defect, assessing the degree of interventricular shunting and resulting pulmonary artery and left chamber dilation, estimating RV and pulmonary arterial pressures, and defining any associated cardiac defects. TTE often can fully define a VSD; however, TEE is used for intraoperative assessment to clarify anatomic and physiological details and to assess postoperative results.
Cardiac catheterization with angiography has been used not only in quantifying the volume of shunting and determining pulmonary vascular resistance but also in defining multiple smaller VSDs, such as in a “Swiss cheese defect.” Similarly, CT and CMR have been used to quantify ventricular size and function and assess shunt volume, along with defining the ventricular septum in the setting of multiple defects ( ). To calculate VSD shunting, the LV stroke volume provides an estimate for Qp (flow to the lungs returning from the pulmonary veins), while the RV stroke volume provides an estimate of Qs (the systemic flow returning from the systemic veins). The difference in the ventricular stroke volume (LV − RV) in the absence of any valvar regurgitation provides an estimate of shunt volume. CMR phase-contrast imaging also can be used to quantify left-to-right shunting by directly estimating Qp (pulmonary artery flow) as opposed to Qs (ascending aorta flow). Three-dimensional printing from CT or CMR imaging is an emerging technology that has the potential to better delineate the size and location of the VSDs, as well as define the proximity to adjacent structures, such as the papillary muscles, moderator band, and trabeculations/muscle bundles, which can significantly affect the management approach, especially in surgically remotely accessible muscular VSDs. These models further allow the interventionalist or surgeon to plan and even simulate the procedure before the actual procedure, hence helping to practice personalized medicine ( Fig. 3.13 ) ( ).
