Fig. 6.1
Schematic description of ASL perfusion imaging. Labeled images are obtained when labeled arterial blood with inverted magnetization reaches the brain tissue in the imaging plane, while control images are obtained without effective labeling of blood. Perfusion-related signals can be obtained by subtracting the labeled images from the control images
6.2 Quantification of Regional Cerebral Blood Flow
Theoretically, ASL enables us to quantify regional cerebral blood flow (rCBF) [4]. In this quantification, the length of time after labeling needs to be taken into account, since inverted spins in the arterial blood will return to the steady state with a time constant of T1, which is approximately 1.4 s at 1.5 T. After a post-labeling delay much longer than the blood T1, the effect of inverted magnetization would be rapidly diminished. Higher magnetic field strength has advantages over conventional field strength in ASL not only due to its improved intrinsic signal-to-noise ratio but also its prolonged T1 of blood (approximately 1.6 s at 3 T), which in turn results in more durable inverted arterial blood spins as an intrinsic tracer.
Figure 6.2 shows a typical rCBF maps obtained using ASL. Previous studies have demonstrated that quantitative in vivo measurement of rCBF by ASL is feasible in healthy subjects, based on a comparison of this approach with the gold standard method of positron emission tomography (PET) using 15O-labeled tracers [5, 6]. ASL rCBF measurement is prone to failure in subjects with pathological conditions, especially those with steno-occlusive lesions of major cerebral arteries [7]. Major arterial steno-occlusive lesions result in a delayed arrival of the labeled blood at the brain tissue due to both lowered perfusion pressure and blood supply via collateral circulation. The transit time for the labeled blood to reach the imaging plane is called the arterial transit time (ATT). The prolonged ATT can profoundly affect the accuracy in rCBF measurement. In ASL, the labeled arterial blood must reach the brain tissue before a labeled image can be obtained. To meet this requirement, the labeled images are obtained after a certain post-labeling delay. Since the post-labeling delay cannot be much longer than the T1 of the arterial blood, the typical delay setting for clinical 3 T scanners ranges from 1.5 to 2 s. These post-labeling times are long enough for healthy young individuals in whom ATT is relatively short. However, in patients with steno-occlusive lesions in the major cerebral arteries, in whom ATT will be much longer, a post-labeling delay of 1.5–2 s would be inadequate. Consequently, the labeled images are scanned before the labeled blood reaches the brain tissue, which in turn results in underestimation of rCBF. Under these conditions, the labeled blood stays in the arterial vessels at the time of imaging. This labeled blood within the arteries can create spotty areas of high blood flow in the final blood flow maps, and these artifactual hyperperfused areas may coexist with the underestimated rCBF, completely degrading the rCBF mapping. Figure 6.3 shows a typical example. To minimize the effect of residual intra-arterial-labeled blood, ASL pulse sequences are often implemented with crusher gradients, which selectively attenuate signals from flowing blood [8]. The normal range of ATT is known to be longer in the elderly population. Since dementia is a geriatric disease, it should be noted that underestimation of rCBF due to prolonged ATT can occur among healthy elderly individuals, especially in the vascular border zones [9], where ATT is always longer than the other regions (Fig. 6.4).
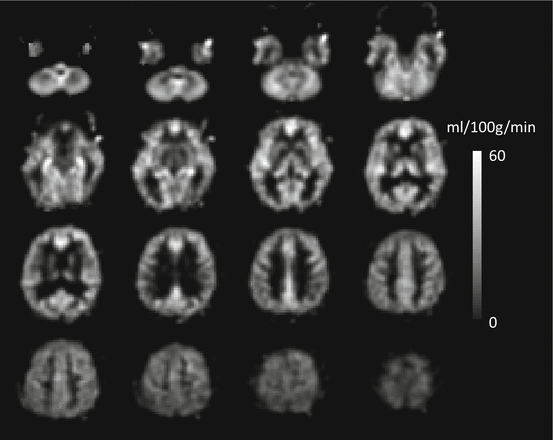
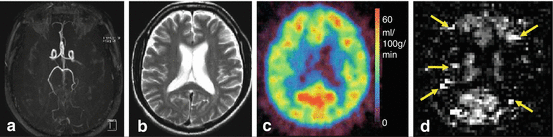
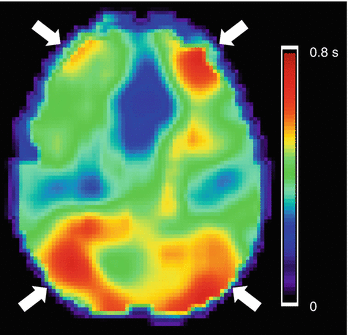
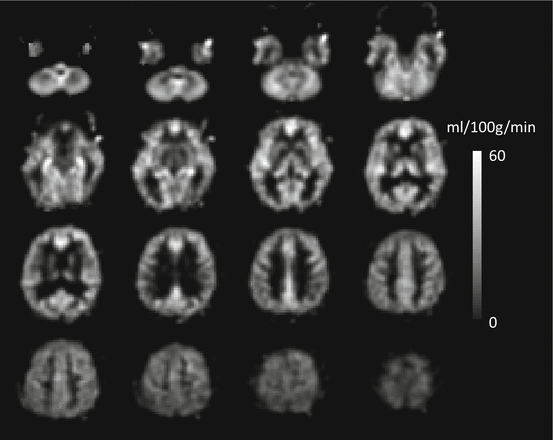
Fig. 6.2
rCBF maps of a healthy subject
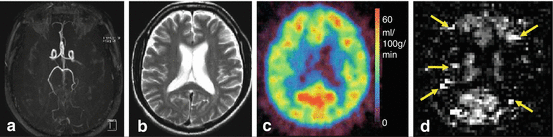
Fig. 6.3
Effect of ATT prolongation on ASL images. A patient with bilateral middle cerebral artery occlusion on MR angiography (a) but without obvious infarction on a T2-weighted image (b) underwent pulsed ASL imaging and 15O PET. PET (c) reveals preserved blood flow, presumably due to collateral blood supply. In the ASL image (d), a complete deficit of blood flow is observed in the bilateral middle cerebral artery territories, likely due to ATT prolongation. Spotty hyperperfused areas (arrows) are seen in the periphery of the perfusion deficits, indicating labeled blood in the arteries
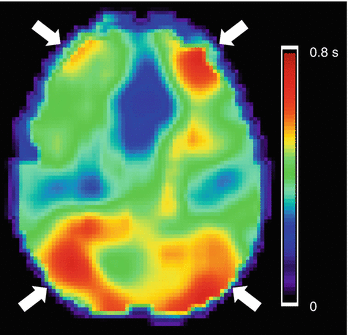
Fig. 6.4
ATT map of a healthy subject. Note that ATT is prolonged in the bilateral border zones (arrows)
In clinical imaging, ASL measurement can be deteriorated by several other factors. Patients with cognitive disorders often find it difficult to stay still during the scan. As ASL rCBF measurement includes subtraction between the labeled and control images, the patients’ head motion can seriously degrade the rCBF measurement. Magnetic field inhomogeneity due to air-filled paranasal sinuses and mastoid air cells, skull base bones, or implanted metallic devices may result in failure of ASL, especially when echo planar imaging sequence is employed. A metallic stent in the neck where labeling inversion pulses are applied can also cause problems.
6.3 ASL Pulse Sequences
There are two distinct types of ASL pulse sequences, pulsed ASL (PASL) and continuous ASL (CASL). In PASL, labeling of arterial blood is carried out in short duration over a relatively thick labeling slab, whereas in CASL a continuous labeling pulse is applied over a thinner labeling slab. Compared to CASL, PCASL is easier to implement in clinical MR scanners. However, PASL sequences generally suffer from lower SNR and higher sensitivity to ATT prolongation. To mitigate the latter issue, sophisticated PASL sequences with multiple inversion time sampling have been proposed [13, 14]. On the other hand, the longer labeling in CASL results in a larger number of labeled spins, thereby improving the signal-to-noise ratio in rCBF measurement. Moreover, CASL has an advantage over PCASL in terms of its lower sensitivity to ATT prolongation. However, due to the longer labeling pulse, CASL tends to deposit higher amounts of energy, often exceeding the regulatory limit on the specific absorption rate. This issue of CASL has been solved by using a series of short labeling pulses in a scheme called pseudo-continuous ASL or pulsed continuous ASL (PCASL) [15, 16]. PCASL has been combined with 2-D and 3-D acquisition and is fastly becoming the standard ASL method [17].
6.4 Analysis of ASL rCBF Maps
Imaging of rCBF during the resting state is known to be useful in diagnosing neurodegenerative dementias such as Alzheimer’s disease. It is conceivable that rCBF parallels the functional activity of the neural tissue. Thus a deficit in rCBF could reflect a functional failure representing either a degeneration of local brain tissue or its transneuronal effects in remote regions. The rCBF in dementia has been evaluated using nuclear medicine techniques such as PET utilizing 15O-labeled water and single-photon emission tomography (SPECT). In clinical practice, SPECT has been the most widely available modality (see Chap. 11: Brain perfusion SPECT in Alzheimer’s disease). Although SPECT is an established technique, ASL has several key advantages over SPECT. Most importantly, ASL does not use radiation or contrast administration and is completely noninvasive. In addition, ASL is superior to SPECT in terms of spatial resolution. Finally, ASL can be easily incorporated into a clinical MR imaging protocol, thereby allowing simultaneous morphological evaluation. This could lead to improved cost-effectiveness.
With these theoretical strengths in mind, researchers have investigated the feasibility of using ASL in the diagnosis of dementia, especially Alzheimer’s disease [18, 19]. The rCBF maps obtained with ASL can be evaluated both qualitatively and quantitatively. Quantitative evaluation is popular among the research community, since it provides more objective information. In the past reports, ASL rCBF maps have been analyzed with almost the same technique as used for SPECT rCBF maps. The quantitative analysis of ASL rCBF maps may include statistical methods, such as statistical parametric mapping (SPM). In these analyses, the rCBF map of each individual patient is normalized according to a common template of a standard anatomical space (a predefined “average brain”) so that the rCBF of different individuals can be compared on a voxel-by-voxel basis. Alternatively, mean rCBF values are measured in anatomically labeled brain regions on each patient’s native rCBF map using region-of-interest (ROI) templates defined in the standard space which are subsequently warped according to the native space.
Since ASL theoretically allows for quantitative rCBF measurement, it is possible that analysis of absolute rCBF maps is meaningful. However, rCBF values are often normalized, especially for the diagnosis of dementia. Normalization of rCBF could effectively eliminate variations in absolute rCBF measurement, which may originate from both physiological variability of global CBF and scaling error in absolute rCBF measurement. The normalization results are critically dependent on the choice of reference region: the reference regions should be among those least affected by the disease. In the past literature, localized reference regions such as the sensorimotor (or motor) and calcarine cortices were used.
ASL has better spatial resolution than SPECT. Nevertheless, it is still not high enough to clearly separate the white matter (WM), cortical gray matter (GM), and surrounding cerebrospinal fluid (CSF), and thus a substantial partial volume effect (PVE) in voxels involving the cortical GM is unavoidable. Many dementing diseases are associated with cortical volume loss, which can result in a decreased GM volume fraction in an imaging voxel. This in turn results in underestimation of the CBF of local GM. Correction for the PVE has been often implemented in the generation of rCBF maps in which corresponding high-resolution 3D anatomical MR images, which can distinguish the three compartments, are coregistered to the rCBF maps and used as a reference [20].
6.5 ASL of Alzheimer’s Disease and Other Dementias
Previous nuclear medicine studies reported that the hypoperfusion related to Alzheimer’s disease typically involves several brain regions, including the posterior cingulate gyrus and precuneus, as well as the association cortex in the parietal and temporal lobes [21, 22]. Perfusion abnormality on ASL is seen in essentially the same brain regions as those on PET and SPECT [19, 20, 23–25] (Fig. 6.5), as shown in a few previous studies that compared ASL hypoperfusion with results of PET studies using the same subjects for validation [26–28].