Scaffold
Manufacturer
Design
Drug-elution
Strut thickness (μm)
Resorption time (months)
Polymeric
Igaki-Tamai
Kyoto medical
Zigzag hoops
No
170
24–36
BVS1.0
Abbott vascular
Circumferential hoops
Everolimus
150
24–48
BVS1.1
Abbott vascular
In-phase zigzag hoops linked by bridges
Everolimus
150
36–60
DESolve gen 1
Elixir medical
In-phase zigzag hoops linked by bridges
Myolimus
150
12–25
DESolve gen 2
Elixir medical
In-phase zigzag hoops linked by bridges
Novolimus
150
12–25
REVA
Reva medical
Slide-and-lock
No
114–228
4–6
ReZolve
Reva medical
Slide-and-lock
Sirolimus
114–228
4–6
ReZolve2
Reva medical
Slide-and-lock
Sirolimus
114–228
4–6
ART
Arterial remodeling technologies
Zigzag hoops
No
170
18–24
IDEAL
Xenogenics
In-phase zigzag hoops linked by bridges
Sirolimus
200
Unknown
Xinsorb
Huaan biotech
Zigzag hoops
Sirolimus
160
12–24
ON-ABS
OrbusNeich
Zigzag hoops
Abluminal Sirolimus and luminal + CD34
150
Unknown
FORTITUDE
Amaranth
In-phase zigzag hoops linked by bridges
No
120
12–24
FORTITUDE gen 2
Amaranth
In-phase zigzag hoops linked by bridges
Sirolimus
120
12–24
Metallic
AMS
Biotronik
Tubular slotted
No
Unknown
4–6
DREAMS gen 1
Biotronik
Zigzag hoops
Paclitaxel
120
12
DREAMS gen 2
Biotronik
In-phase zigzag hoops
Sirolimus
140
12
13.2 Challenges for the Evaluation of Bioresorbable Scaffolds
Bioresorbable scaffolds should possess a variety of key characteristics that are set by metallic stents, such as widespread availability, storage under standard conditions in the catheterization laboratory, fast preparation of the device, wide ranges of available sizes and lengths, a low crossing profile, good visibility under X-ray and a controlled and predictable expansion range. Polymeric scaffolds have however different material characteristics than metallic stents. The first polymeric scaffolds had to be stored under special conditions and had a shorter shelf-life than metallic stents. Today’s bioresorbable scaffolds have similar storage conditions and shelf-lives to metallic stents.
Compared to metallic stents, bioresorbable materials are relatively rigid and non-elastic, resulting in the weakening of the polymeric scaffold by expansion and deformation, thus requiring careful sizing to the coronary artery dimensions. The majority of scaffolds have a balloon-expandable design, but there are also scaffolds with a self-expanding design (Igaki-Tamai) [2] or a slide-and-lock design (REVA), that is deployed by sliding open and is locked into place [3].
Another important difference between the stent types lies in the fact that polymers are invisible under X-ray and thus suffer from poor visualization by coronary angiography. Most of the scaffolds are equipped with radio-opaque markers on both ends of the scaffold or on both ends of the delivery balloon [2, 4, 5], whereas in the REVA bioresorbable scaffold a proprietary iodinated material is added to the polymer that allows visualization of the entire scaffold under X-ray [3].
Coronary angiography is limited to assess scaffold expansion or scaffold degradation over time due to the poor spatial resolution and the radiolucence of the scaffold’s polymer backbone. Furthermore, coronary angiography tends to underestimate the vessel dimensions (Fig. 13.1) [6, 7]. Intracoronary imaging techniques such as intravascular ultrasound (IVUS) or optical coherence tomography (OCT) can overcome these limitations [8, 9]. IVUS can provide real-time high-resolution cross-sectional images of the vessel wall and scaffold. IVUS, however, has a tendency to slightly overestimate vessel dimensions (Fig. 13.1) and is hampered by the echogenic blooming effect caused by the polymeric struts (Fig. 13.2). Additionally, the limited resolution of IVUS (80–150 μm) compared to OCT (10–15 μm) and the speckled echo signal from blood – that decreases the contrast between the lumen and vessel wall – make the assessment of scaffold expansion and especially the diagnosis of malapposition difficult by IVUS.
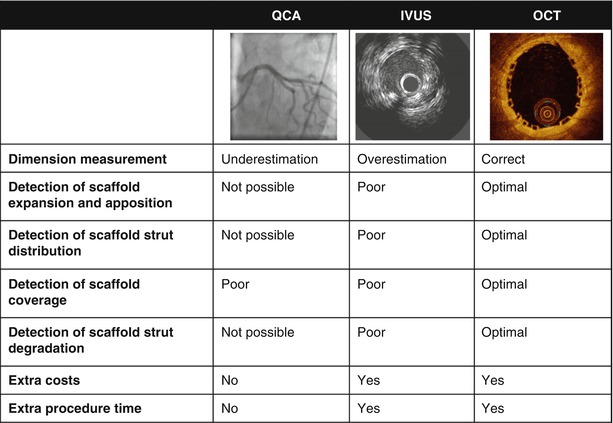
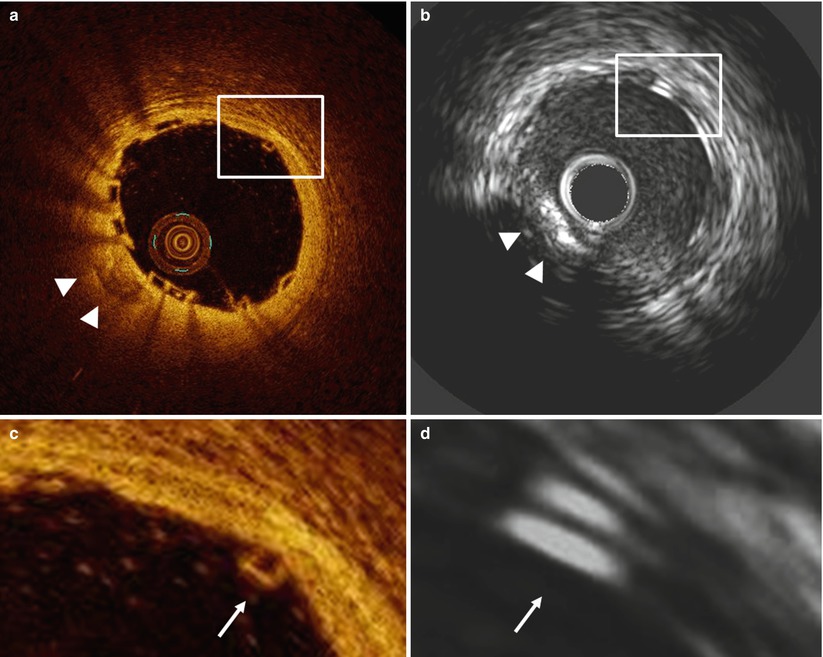
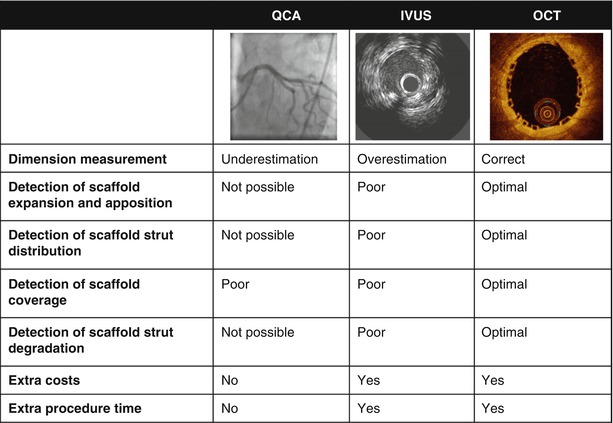
Fig. 13.1
Advantages of optical coherence tomography (OCT)
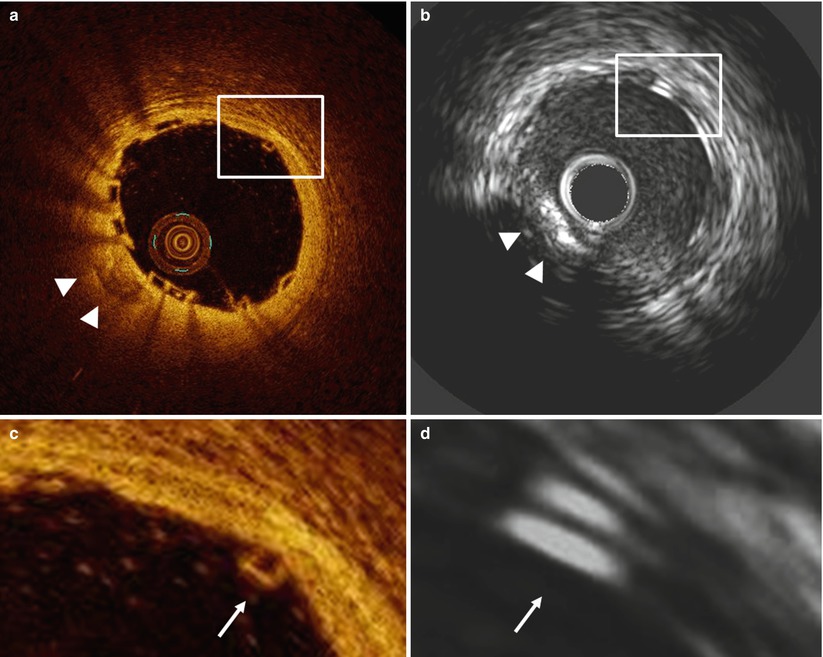
Fig. 13.2
OCT and IVUS immediately after Absorb BVS-implantation in a patient with stable angina. The OCT cross-section (a) and magnification (c) demonstrate a box-shaped appearance of the scaffold struts (arrow), while in the IVUS cross-section (b) and magnification (d) the scaffold struts have a double-strut appearance due to the echogenic blooming effect of the polymeric struts of the Absorb bioresorbable vascular scaffold (Abbott Vascular, Santa Clara, USA). The arrowheads indicate a calcified region visible in both the OCT (a) and IVUS (b) cross-section
The high resolution of intracoronary OCT and the high contrast between lumen and vessel wall (Fig. 13.2) offers advantages for the assessment of atherosclerotic plaque [10], scaffold apposition (Fig. 13.3) and tissue coverage [11, 12] and demonstrated consistently high accuracy and reproducibility in the assessment of coronary stents and scaffolds, irrespective of the analysis method or software used [13–18].
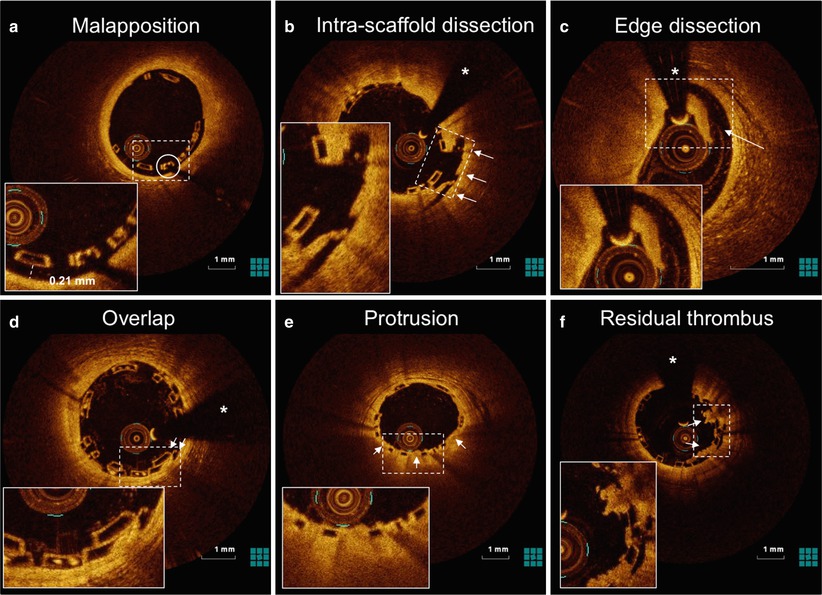
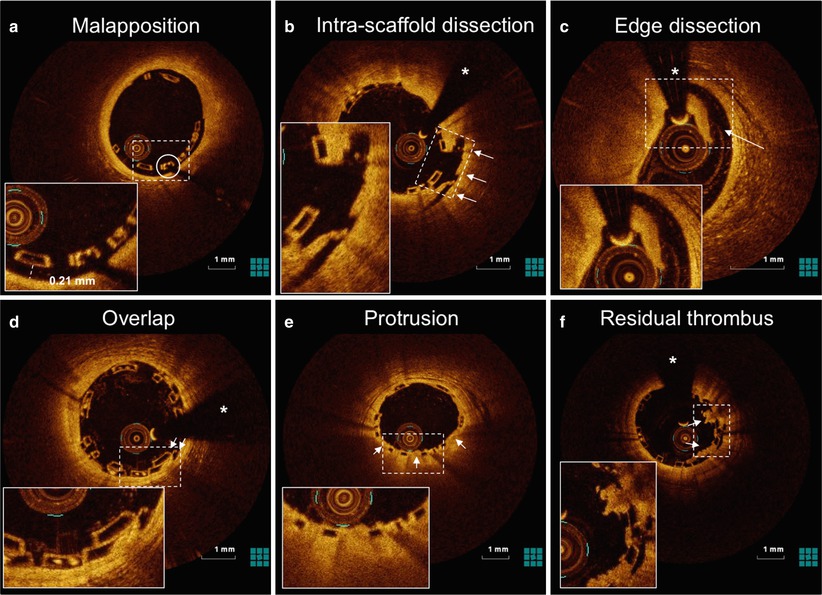
Fig. 13.3
OCT can accurately detect scaffold apposition and peri-procedural vessel injury. OCT cross-sections of Absorb BVS demonstrate mild malapposition (a), intra-scaffold dissection (b; arrows), edge dissection (c; arrow), scaffold overlap (d; arrows), tissue protrusion between the scaffold struts (e; arrows) and residual thrombus on top of the struts (f; arrows) after implantation of an Absorb BVS for ST-elevation myocardial infarction. The asterisk indicates the guidewire artefact, the circle indicates the platinum marker of the scaffold strut. The dotted rectangles indicates the magnification
13.3 Intracoronary OCT Imaging of Bioresorbable Scaffolds
Intracoronary OCT enables high-resolution, in–vivo, serial imaging of the scaffold and has proven beneficial for the straight-forward detailed evaluation of atherosclerotic lesions and their composition, the vascular injury caused by the implantation procedure, the expansion, the apposition (Fig. 13.3) and mechanical integrity of the scaffold over time, the effect of the underlying plaque on the performance of the scaffold, and the position of the scaffold relative to the side branches [19–23].
13.3.1 OCT Can Reliably Guide Treatment Strategy
Currently available bioresorbable scaffolds have relatively thick struts (114–228 μm), resulting in a relatively high crossing profile. This means that adequate lesion preparation, e.g. by Pre-dilatation, or another appropriate technique, is crucial to pass the device for implantation. Accurate determination of the lumen size and lesion characteristics prior to bioresorbable scaffold implantation is important to ensure appropriate sizing of the device. For instance, the Absorb bioresorbable vascular scaffold (BVS) (Abbott Vascular, Santa Clara, CA) allows for a maximum over-expansion of 0.5 mm compared to the nominal scaffold diameter. Post-dilatation with balloons inflated >0.5 mm above the indicated scaffold diameter increases the risk of fracture. OCT can accurately determine lumen and vessel dimensions as well as lesion characteristics and thus guide treatment strategy, in terms of the selection of an appropriate balloon and scaffold diameter and length [6].
13.3.2 OCT Can Precisely Assess Scaffold Expansion and Strut Apposition
The major concern in inadequate scaffold expansion and incomplete strut apposition is that it can cause non-laminar and turbulent blood flow that can trigger platelet activation, thrombosis or restenosis [24, 25]. OCT allows for the assessment of scaffold expansion in relation to the distal and proximal reference segments and to side branches, as well as strut apposition against the vessel wall. The accurate evaluation of scaffold expansion and apposition decreases the risk of incomplete scaffold expansion, recoil, fracture or thrombosis [26, 27].
13.3.3 OCT Can Reliably Assess Scaffold Strut Distribution
Scaffold strut distribution can influence the concentrations of drug reached within the vessel wall, the radial support of the vessel wall and the access to side branches. Therefore, assessment of the scaffold strut distribution is important. Non-uniform strut distribution, which is hypothesized to be caused by calcified deposits occupying >75° of the vessel circumference [28], may cause non-uniform drug-concentrations within the vessel wall, potentially affecting long-term outcome [29]. OCT allows for detailed analysis of the composition of the atherosclerotic lesion, including the size and extent of calcium, and of the strut distribution pattern, including the assessment of the maximum circular unsupported surface area as well as the assessment of side branch access through the scaffold struts and the impact of struts on side branch patency [30–33].
13.3.4 OCT Can Accurately Assess Scaffold Strut Coverage
The absence and the exaggerated development of neointimal coverage, potentially with the subsequent development of neoatherosclerosis, have been related to late (drug-eluting) stent thrombosis [34, 35]. OCT can reliably detect early and very thin layers of coverage on top of scaffold struts and permits the quantification of the coverage with high reliability [27, 36]. OCT allows the assessment of scaffold area, neointimal area and symmetry as well as lumen area [37–39] and permits the qualitative characterization of neointimal tissue [40, 41].
13.3.5 OCT Allows for Detailed Assessment of Scaffold Strut Degradation
Scaffold strut degradation is of growing clinical interest. OCT can visualize with detail the appearance of the scaffold struts and changes in the strut-appearance over time [4]. In the first-in-man evaluation of the Absorb bioresorbable vascular scaffold (BVS), OCT demonstrated changes in the optical properties of the scaffold struts.
Preclinical observations in coronary arteries of healthy swine demonstrated that OCT can accurately diagnose individual struts as well as the strut/vessel wall interaction acutely (Fig. 13.4) and over time. At the 4 year follow-up, OCT demonstrated absence of struts in the vascular wall, with complete degradation of the scaffold confirmed by histology. It is important to note that at the mid-term follow-up (2 years) OCT demonstrated a box-shaped appearance of the scaffold strut that can be associated with strut replacement by proteoglycan [42].
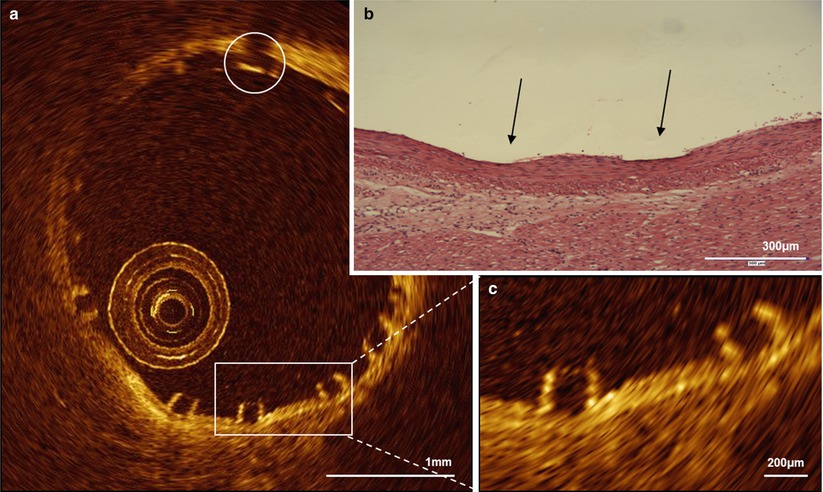
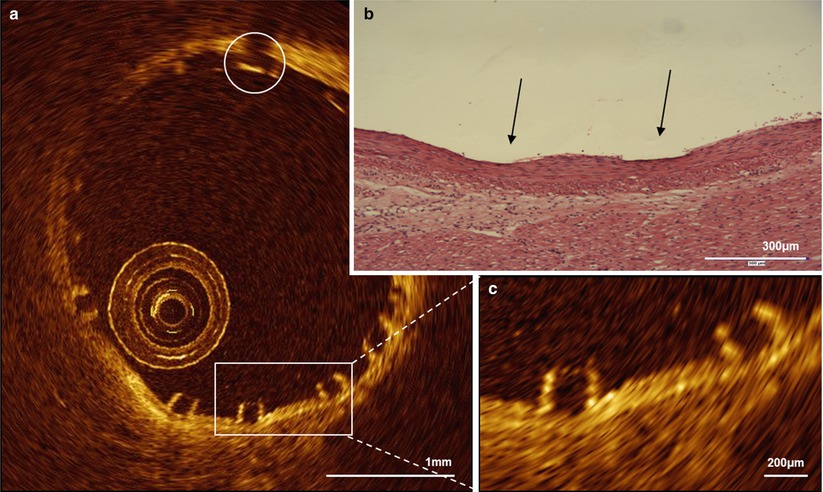
Fig. 13.4
OCT and histology of the Absorb BVS immediately after implantation in a healthy, young swine. OCT (a, c) can accurately visualize the vessel/ wall interaction after implantation of an Absorb bioresorbable vascular scaffold (Abbott Vascular, Santa Clara, CA, USA) in a coronary artery of a healthy, young swine. In the magnification the typical box-shaped appearance of the scaffold struts can be appreciated, which slightly compress the media (c), as confirmed by the histological cross-section (b). The circle indicates the platinum marker of the scaffold strut. The arrows indicate the indentation of the scaffold on histology
13.3.6 Advantage of Three-Dimensional OCT
The unique ability of OCT to reconstruct three-dimensional (3D) images allows better visualization of the scaffold surface and can provide additive information on the result of PCI, particularly in complex lesions [43, 44]. The application of 3D OCT within the coronary bifurcation seems promising, as the visualization of the complex anatomy of the bifurcation and the effects of intervention are difficult and not always reliable with two-dimensional imaging [32, 45]. 3D-OCT can identify the configuration of overhanging struts in front of the side branch ostium and can be helpful to assess and correctly position the guide wire.
13.4 Clinical Experience
Numerous bioresorbable scaffolds have been investigated in humans [46]. Some have demonstrated disappointing first findings [47], while others, e.g. the ABSORB trials, have proven more encouraging findings [4].
13.4.1 Polymeric Bioresorbable Scaffolds
13.4.1.1 Igaki-Tamai
The Igaki-Tamai (Igaki Medical Planning Co., Kyoto, Japan) represents the first fully biodegradabable scaffold tested in humans in the 1990’s [2]. It was a self-expanding, poly-l-lactic acid (PLLA) based, non drug-eluting scaffold with a zigzag design and a strut thickness of 170 μm. The scaffold was compatible with 8 F guide catheters and required storage and expansion at a pre-specified temperature.
A clinical trial in 15 patients proved feasibility and demonstrated remarkable results with a target lesion revascularization rate per patient of 6.7 % at 6 months [2]. Six-month intravascular imaging demonstrated no degradation of the scaffold and the absence of scaffold shrinkage. At the 10-year follow-up, similar major adverse cardiac event (MACE) rates to those of bare metal stents (MACE-free survival was 50 % at 10 years) were documented [48] and OCT demonstrated that just the scaffold markers were still visible (Fig. 13.5). Despite the good results, however, further use of the scaffold for treatment of coronary disease was put on hold, mainly because of the pre-specified temperatures required for storage and expansion.
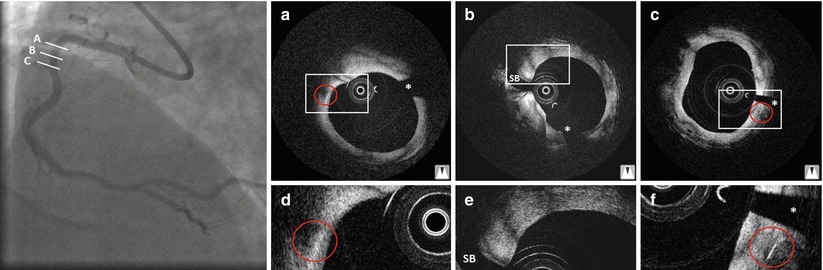
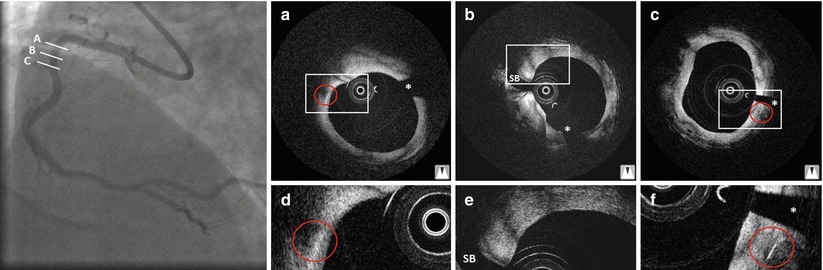
Fig. 13.5
Angiography and OCT 10 year after implantation of an Igaki-Tamai scaffold in a patient with stable angina. Optical coherence tomography cross-sections (a–f) demonstrate that only the markers of the scaffold are still visible (a, c), while the remaining struts are not. The asterisk indicates the guidewire artifact, SB side branch. The rectangles indicate the magnifications on the bottom and the red circles indicate the markers of the scaffold
13.4.1.2 Absorb BVS
The Absorb bioresorbable vascular scaffold (Abbott Vascular, Santa Clara, CA) represents the scaffold with the vastest clinical experience. The scaffold is constructed of a poly-L-lactic acid (PLLA) backbone coated with poly-D,L-lactic acid (PDLLA) that contains the antiproliferative drug everolimus and controls its release (Fig. 13.4) [49].
In the ABSORB Cohort A trial, a prospective, multi-center registry in 30 patients with a single, de–novo target lesion in a native coronary artery, the first-generation BVS (BVS1.0) was investigated [4]. The majority of patients received a 3.0 × 12.0 mm scaffold, two patients received a 3.0 × 18 mm scaffold. The BVS1.0 was designed with a crossing profile of 1.4 mm in circumferential hoops and a strut thickness of 150 μm that were either directly joined or linked by straight bridges. The scaffolds had to be kept at a minimum temperature of −20 °C to prevent physical aging of the polymer and to ensure device stability.
This first-in-man-trial trial demonstrated the feasibility of the everolimus-eluting BVS1.0. Procedural success was 100 %, and device success 94 % [4, 50, 51]. At 1 year, the MACE-rate was 3.3 % of 30 patients. One patient suffered a non-Q wave myocardial infarction. There were no late stent thromboses. At 2 years vasomotion restored, and the scaffold was clinically safe without cardiac death, ischaemia-driven target lesion revascularisation, or stent thrombosis [4, 50]. Long-term results up to 5 years confirmed favourable clinical outcomes, without any cardiac death or scaffold thrombosis and ischaemia-driven MACE rate remained unchanged (3.4 % of 29 patients) [52]. Furthermore, late luminal enlargement (Fig. 13.6) without adaptive vessel remodelling was observed up to 5 year follow-up [53–56].
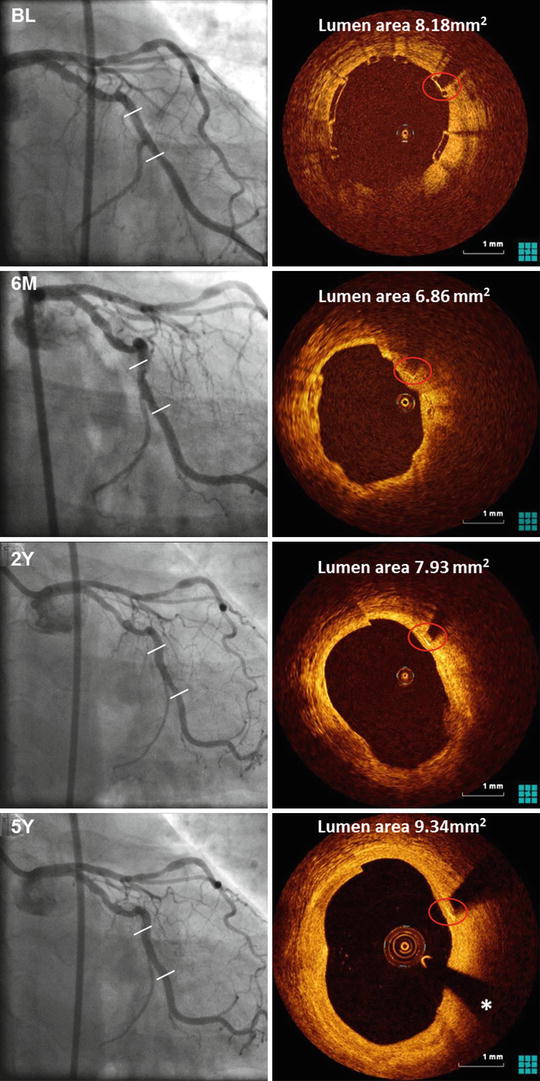
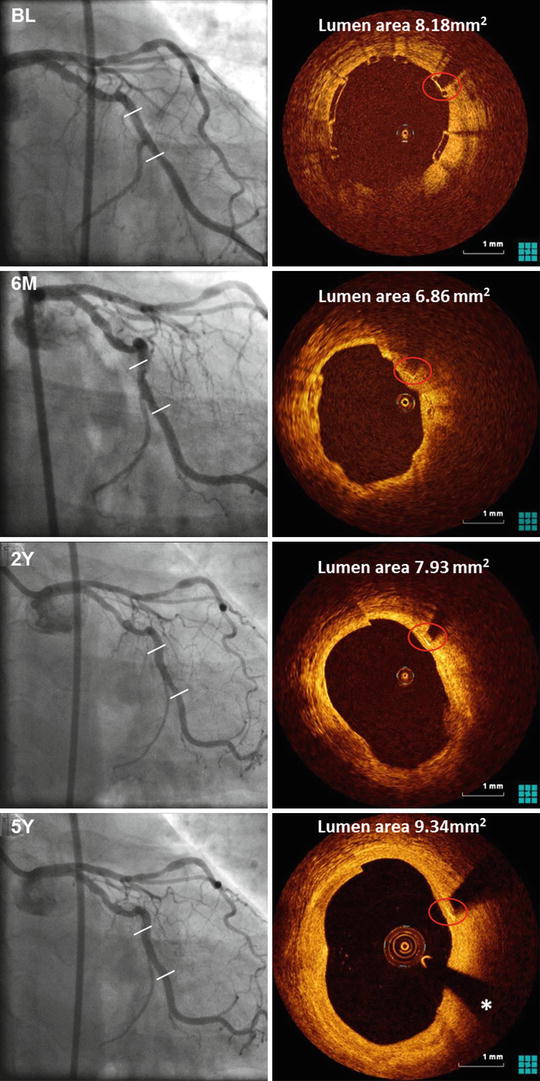
Fig. 13.6
Angiography and OCT of an Absorb BVS immediately after, 6 months and 2 and 5 year after implantation in a patient with stable angina. The distal and proximal edge of the scaffold are depicted by white lines in the coronary angiogram (left). The box-shaped appearance of the scaffold struts can be clearly visualized by OCT immediately after implantation. At 5 year follow-up, the marker of the scaffold strut is visible (red circle), while the remaining scaffold struts are not. The asterisk indicates the guidewire artefact
The OCT substudy of this first-in-man trial, including 13 patients, described changes of the strut appearance at follow-up that were thought to be associated with degradation of the scaffold over time.
In the preclinical study of the BVS1.0 implanted in healthy young swine, however, the assessment of polymer degradation by chemical analysis demonstrated that the preservation of the box-shaped appearance did not exclude resorption of polymeric struts [42]. Two years after implantation, the polymer was no longer accurately quantifiable by chemical degradation analysis, but the structures observed with OCT represented regions corresponding to the location of the scaffold struts. At the 4 year follow-up, OCT demonstrated an absence of struts in the vascular wall, indicating its ability to assess complete degradation of the scaffold in–vivo.
In the ABSORB Cohort B trial, a prospective, multi-centre registry of patients with a single, de–novo target lesion in a native coronary artery, the second-generation Absorb BVS (BVS 1.1) was investigated. The BVS1.1 is similar to the BVS1.0 with the same polymer mass, strut thickness and drug coating. The manufacturing process of the BVS1.1 has however been modified to enhance the mechanical strength and durability of the struts [30]. The BVS1.1 has in-phase zigzag hoops linked by bridges that allow for more uniform strut distribution, reducing the maximum circular unsupported surface area and providing more uniform vessel wall support and drug transfer [33].
Clinical results demonstrated a low MACE-rate at 6 months (4.4 %), representing one myocardial infraction and one target lesion revascularization. No death or thrombosis occurred [57]. At 1 year, the MACE-rate was 7.1 %, representing two myocardial infarctions and 2 target lesion revascularizations, whereas no cardiac death occurred. At 3 years, the MACE-rate was 10.0 % without any scaffold thrombosis. The return of vasomotion was observed at 12 months and more pronounced at 24 months, suggesting a link between scaffold degradation and restoration of vasomotion in the treated segments [58].
The OCT substudy demonstrated preservation of the box-shaped appearance of the scaffold struts at 6 months [30], which is in line with preclinical observations in atherosclerotic swine [59] 6 months after Absorb BVS1.1 implantation (Fig. 13.7).
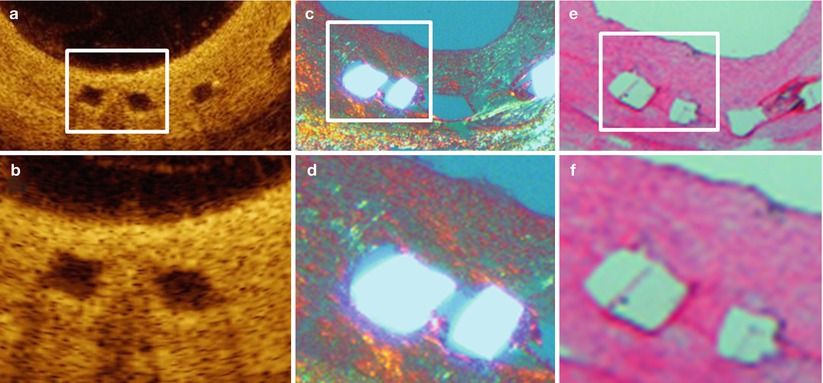
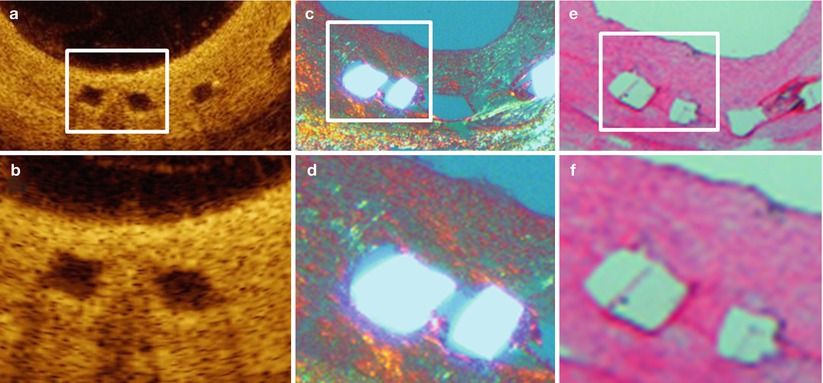
Fig. 13.7
OCT and histology 6 months after Absorb BVS1.1 implantation in an atherosclerotic swine. The OCT cross-sections (a, magnification in b) clearly demonstrate the box-shaped appearance of the scaffold struts. Preservation of the scaffold struts is confirmed by histology, demonstrating presence of the polymeric struts under polarization light microscopy (c, magnification in d) and of the strut voids on haematoxylin eosin staining (e, magnification in f)
In the ABSORB EXTEND trial, a non-randomized, single-arm, continued access trial, 800 patients with up to two de novo lesions in a native coronary artery were treated with BVS 1.1 to continue the assessment of the safety and performance of the BVS1.1 in a large and more complex population. The preliminary report of the 12-month clinical outcomes for the first 512 patients demonstrated that the composite endpoints of ischaemia-driven MACE and ischaemia-driven target vessel failure were low (4.3 and 4.9 %, respectively). The cumulative rate of definite and probable scaffold thrombosis was 0.8 % [60].
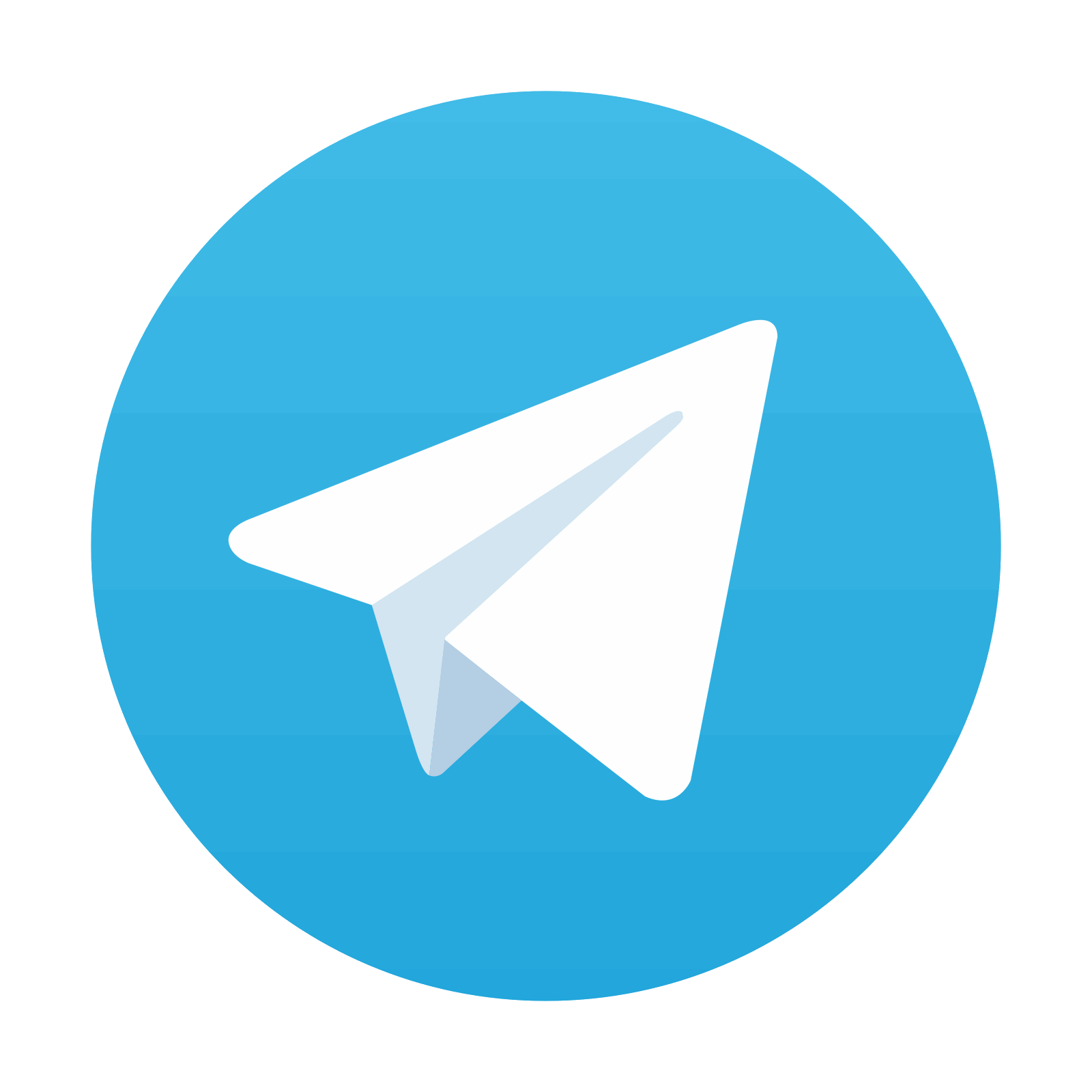
Stay updated, free articles. Join our Telegram channel

Full access? Get Clinical Tree
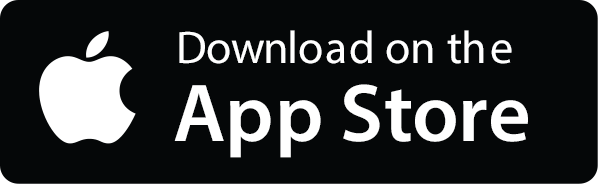
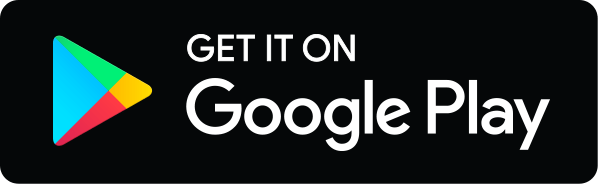