Total
Males
Females
Age at initial diagnosis
O
O/E
EAR
O
O/E
EAR
O
O/E
EAR
All ages
185,407
1.14a
21
100,428
1.11a
22
84,979
1.17a
21
00–17
351
6.13a
15
176
6.44a
15
175
5.84a
15
18–29
1,401
2.92a
22
562
3.39a
22
839
2.67a
23
30–39
4,909
2.37a
39
1,530
2.88a
40
3,379
2.20a
38
40–49
13,537
1.61a
39
4,466
1.83a
52
9,071
1.52a
34
50–59
34,159
1.27a
32
15,957
1.33a
46
18,202
1.21a
24
60–69
62,286
1.13a
23
35,986
1.11a
25
26,300
1.14a
22
70–79
52,321
1.02a
4
32,419
1.00
0
19,902
1.05a
9
80–115
16,443
0.92a
−19
9,332
0.92a
−26
7,111
0.93a
−14
Notable differences were apparent by age at first cancer diagnosis with 6-fold relative risks for survivors of childhood cancer (O/E = 6.13) (Table 1), 2- to 3-fold increased risks for patients diagnosed as young adults (ages 18–39 years), and 1.2- to 1.6-fold elevated risks for ages 40–59 years. However, the largest burden of new malignancies in general was experienced by patients initially diagnosed with cancer at ages 30–49 years, with an EAR of 39 per 10,000 person-years.
For all cancers considered together, almost 14 % of SEER patients were diagnosed with a second cancer by 25 years of follow-up (cumulative incidence of 5.0, 8.4, 10.8, and 13.7 % at 5, 10, 15, and 25 years, respectively). Cumulative incidence of second cancer also varied by age at first cancer diagnosis, and was highest among those diagnosed between 50 and 69 years of age (16.4 % at 25 years of follow-up). Although children (ages <18 years) demonstrated a 3.5 % cumulative incidence of second cancer at 25 years, it is expected that risk will continue to rise as these patients enter the age period of increasing cancer incidence.
3.3 Overall Patterns of Multiple Primary Cancers
Tobacco and Alcohol. The risk of subsequent cancers following initial primary cancers that have been typically associated with tobacco and/or alcohol (e.g., cancers of oral cavity/pharynx, esophagus, larynx, and lung) is shown in Table 2. It should be noted that over 11,000 of the 24,688 subsequent cancers among these patients similarly developed at these sites (O/E = 3.62). Curtis et al. (2006) pointed out that tobacco/alcohol-related cancer sites accounted for more than 35 % of the total excess cancers occurring in the SEER Program. The rate of excess cancers (EAR) was estimated at 114 cases per 10,000 person-years. Curtis and colleagues also reported a differential influence of tobacco or alcohol when the initial cancers were evaluated by histologic type (e.g., squamous cell carcinoma versus adenocarcinoma of the esophagus), by cancer subsite (e.g., renal pelvis versus renal parenchyma), or by earlier age at initial diagnosis (e.g., age <70 versus older ages). Further, the known impact of tobacco and alcohol on second cancer risk is also shown by the risk reductions that have been observed with cessation of exposures following the initial cancer diagnosis (Do et al. 2004).
Table 2
Risk of subsequent primary cancers following first primary cancers that are strongly related to tobacco and/or alcohol exposure (oral cavity and pharynx, esophagus, larynx, lung, and bronchus), by sex, SEER 1973–2000
Total | Males | Females | |||||||
---|---|---|---|---|---|---|---|---|---|
Subsequent primary cancer | O | O/E | EAR | O | O/E | EAR | O | O/E | EAR |
All subsequent cancers | 24,688 | 1.64a | 114 | 17,491 | 1.58a | 120 | 7,197 | 1.82a | 105 |
Oral/pharynx, esophagus, larynx, and lung/bronchus | 11,593 | 3.62a | 99 | 8,184 | 3.20a | 105 | 3,409 | 5.33a | 90 |
Oral/pharynx | 2,510 | 9.04a | 26 | 1,742 | 7.78a | 28 | 768 | 14.29a | 23 |
Larynx, lung/broncus | 8,084 | 2.95a | 63 | 5,704 | 2.62a | 66 | 2,380 | 4.26a | 59 |
Esophagus | 999 | 5.49a | 10 | 738 | 4.74a | 11 | 261 | 9.94a | 8 |
Bladder, renal pelvis, ureter, and kidney parenchyma | 1,772 | 1.44a | 6 | 1,449 | 1.39a | 8 | 323 | 1.71a | 4 |
Bladder, renal pelvis, ureter | 1,325 | 1.42a | 5 | 1,116 | 1.38a | 6 | 209 | 1.68a | 3 |
Kidney parenchyma | 447 | 1.48a | 2 | 333 | 1.40a | 2 | 114 | 1.78a | 2 |
Pancreas | 531 | 1.36a | 2 | 346 | 1.28a | 1 | 185 | 1.55a | 2 |
Cervix uteri | 60 | 1.16 | <1 | – | – | – | 60 | 1.16 | <1 |
Stomach | 474 | 1.39a | 2 | 395 | 1.44a | 2 | 79 | 1.17 | <1 |
All other cancers | 10,258 | 1.05a | 5 | 7,117 | 1.03a | 4 | 3,141 | 1.08a | 8 |
Therapeutic Effects. Based on the systematic review of all SEER program data, Curtis et al. (2006) concluded that cancer treatment among older adults did not appear to be linked with a sizable excess of subsequent cancers. In contrast, children and young adults appeared to be especially susceptible to the carcinogenic effects of intensive treatment, as reviewed earlier (Travis et al. 2008). In the SEER program, 5-year survivors of childhood cancer given radiotherapy initially had the largest risks of solid tumors, consistent with the long latency period associated with radiogenic solid cancers. Radiotherapy appeared to contribute to the heightened risk for solid tumors arising in areas in which high-dose, large-field radiotherapy had been used in the past, in particular, for second cancers of breast, lung, and other sites among patients with Hodgkin lymphoma, as reported previously (Travis et al. 2002, 2003, 2005, 2008; Gilbert et al. 2003). Elevated risks of acute leukemia were observed after radiotherapy for cancers of cervix and uterine corpus. Among patients treated with radiotherapy for breast cancer, excesses cancers of the lung and esophagus, as well as sarcomas, occurred.
Dietary Factors and Hormones. Information reported to the SEER program does not include lifestyle factors (such as obesity, physical inactivity, and diet) or reproductive information that may influence on cancer risk. However, these variables likely played a role in the increased risk of new malignancies among patients with cancers of the female breast, reproductive organs, and the upper and lower digestive tract. In particular, Curtis et al. (2006) pointed out that, in addition to tobacco and alcohol, a low intake of vegetables and fruits may have had an impact on the excesses of multicentric tumors along the upper aerodigestive tract. Moreover, it was suggested that physical inactivity, excess caloric intake, obesity, and reproductive variables likely influenced the patterns of hormone-dependent tumors (e.g., breast, uterine corpus, ovary, and prostate), as well as colon cancer (Curtis et al. 2006).
Genetic Predisposition. Several patterns of multiple primary cancers in the SEER Program appeared consistent with previously reported familial cancer syndromes. In children and young adults, the association of breast cancer, sarcoma, and other cancers was likely reflective of Li-Fraumeni syndrome, which has been mainly linked with germline mutations of p53 (reviewed in Travis et al. (2006)). Excess subsequent cancers of uterine corpus, ovary, bile ducts, small intestine, and renal pelvis observed among patients with early-onset colon cancer were consistent with hereditary nonpolyposis colon cancer (Lynch syndrome), due to inherited mutations in mismatch repair genes [reviewed in Travis et al. (2006)]. The sizable risks of contralateral breast and ovarian cancer among younger women with breast cancer are consistent with heritable syndromes associated with germline mutations of BRCA1/2.
Infection and Immunosuppression. Several constellations of cancers may have reflected the influence of viruses [e.g., human papillomavirus (HPV), human immunodeficiency virus, human herpesvirus 8, Epstein-Barr virus, hepatitis B and C, and Helicobacter pylori, together with immune dysregulation and inflammation (Hisada and Rabkin 2005; Morgan et al. 2006)]. For example, cancers of cervix and anogenital tract likely reflected a role of HPV infections. The immune dysfunction inherent to chronic lymphocytic leukemia probably contributed to the excess risks of cutaneous melanoma, as reported earlier (Travis et al. 1992).
4 Second Malignancies Among Survivors of Selected Adult Cancers
4.1 Hodgkin Lymphoma
Given the high curability of HL and the generally young age at diagnosis, a considerable body of work has documented the large risk of subsequent malignancies among these patients. The largest excesses of therapy-related leukemias in HL patients occur in the first decade after treatment (van Leeuwen and Travis 2005), with the initial reports dating back to the early 1970s (Arseneau et al. 1972). The elevated risk is largely accounted for by the antecedent alkylating agent chemotherapy, for which strong dose–response relationships have been documented (Kaldor et al. 1990; van Leeuwen et al. 1994). Data with regard to splenectomy for HL as well as the addition of radiation therapy to chemotherapy as additional risk factors for therapy-related leukemia are conflicting (Henry-Amar 1992; Andrieu et al. 1990). The prognosis after a diagnosis of secondary leukemia among HL patients is poor, with a median survival of less than 1 year (Ng et al. 2002). The replacement of mechlorethamine, vincristine, procarbazine, and prednisone (MOPP) combination chemotherapy by adriamycin, bleomycin, vinblastine, and dacarbazine (ABVD) has substantially reduced the risk of leukemia (Schonfeld et al. 2006). Possibly leukemogenic agents, however, are still given during salvage therapy, and are often included in newer regimens, such as bleomycin, etoposide, adriamycin, cyclophosphamide, procarbazine, and prednisone (BEACOPP) (Diehl et al. 2003). Thus, excess secondary leukemias may eventually occur in subgroups of HL patients given selected new chemotherapy regimens.
Although an elevated risk of non-Hodgkin’s lymphoma (NHL) after HL has been reported (van Leeuwen and Travis 2005), the relationship with prior therapy is unclear. Patients with lymphocyte predominant HL have been shown to be at higher risk for developing NHL than those with other histologic types. The prognosis of NHL after HL appears to be comparable to patients with de novo advanced-stage NHL (Rueffer et al. 2001).
Solid tumors have emerged as the major type of second malignancy after HL, comprising up to 75–80 % of all cases (Hodgson et al. 2007). Radiotherapy-associated solid tumors usually develop after a considerably longer latency period (at least 5–9 years) from the time of primary treatment of HL than do secondary leukemias, with increased risks persisting for at least three decades (Hodgson et al. 2007). The majority of second primary solid cancer arise within or at the edges of prior HL radiotherapy fields, supporting an important role for antecedent irradiation. Recent studies have also reported significant relationships with radiation dose for HL to the site of second tumor occurrence for selected solid cancers. In a large international case–control investigation of women treated for HL before age 30 years who developed breast cancer (105 cases; 266 matched controls), radiation dose to the area of the breast where the tumor developed in the case (and a comparable area in matched controls) was estimated for each case–control set (Travis et al. 2003). Breast cancer risk increased significantly with increasing radiation dose to reach 8-fold for the highest category (median dose 42 Gy) compared to the lowest dose group (<4 Gy) (p trend for dose <0.001). In a separate Dutch study of women treated for HL before the age of 40 years (van Leeuwen et al. 2003), similar results were found, with most of the latter patients also included in the international investigation (Travis et al. 2003). In both studies (Travis et al. 2003; van Leeuwen et al. 2003), women who received both chemotherapy and radiation therapy had a significantly reduced risk (about 50 %) compared to women given with radiation therapy alone; moreover, the radiation-associated risks were decreased among women given alkylating agents and/or who received a radiation dose of 5 Gy or more to the ovaries. The Dutch study, in particular, clearly showed that the marked risk reduction associated with chemotherapy was secondary to the high number of women who developed premature menopause. Findings in both investigations indicated that ovarian hormones are an important influence in promoting tumorigenesis, with radiation producing an initiating event (Travis et al. 2003; van Leeuwen et al. 2003).
A highly significant dose–response relationship with radiation has similarly been shown for the development of lung cancer after HL. In an international study by Travis et al. (2002), lung cancer risk increased with increasing radiation dose to the area of the lung in which cancer developed, even among HL patients who received 40 or more Gy (p trend with dose <0.001); risk reached 7- to 9-fold at doses of 30 or more Gy. All risks were calculated in relation to patients who received <5 Gy to the area of the lung in which cancer developed.
It should be pointed out that the data on solid tumors after radiotherapy for HL are based on patients treated in an era during which large treatment fields and very high radiation doses were used. In contrast, radiation treatment fields are now significantly smaller with the current standard consisting of involved-field radiation therapy given as part of combined modality therapy. Further, ongoing investigations are examining the effect of further reductions in radiation treatment dose for HL. Both these dose reductions and the trend toward the use of involved-node radiation therapy will result in additional reductions in the exposure of normal tissue to radiation (Girinsky et al. 2006). Thus, it is likely that HL patients who receive radiotherapy in the modern treatment era will incur a lower risk of solid tumors.
Given the historically important role of radiation therapy in the treatment of HL, there is a deficit of long-term data on late effects in those patients treated with chemotherapy alone. In a British survey of 1,693 HL patients given chemotherapy only, the relative risk of lung cancer was elevated 3-fold (RR = 3.3; 95 % C.I., 2.2–4.7) (Swerdlow et al. 2000). The increased risk of lung cancer was comparable in magnitude to HL patients who received either radiation therapy alone (RR, 2.9, 95 % C.I., 1.9–4.1) or combined modality therapy (RR, 4.3, 95 % C.I., 2.9–6.2). Most HL patients in this study were treated with alkylating-agent based chemotherapy. The important role of alkylating agents given for HL in the subsequent occurrence of lung cancer was confirmed in a case–control study by the same British group (Swerdlow et al. 2001), and in the NCI-international case–control study described above (Travis et al. 2002); both investigations demonstrated significant dose–response relationships between cumulative amount of alkylating agent chemotherapy and lung cancer risk.
Age is an important modifying factor in the risk of selected treatment-related second cancers after HL. Young age at mantle irradiation has consistently been shown to be associated with a significantly increased risk of breast cancer in patients with HL (Travis et al. 2003; Ng et al. 2002; Hodgson et al. 2007; van Leeuwen et al. 2000), which is not consistent with the known radiosensitivity of the breast in young women. In a recent population-based cohort study by Hodgson et al., the absolute risks of breast cancer in women diagnosed with HL at ages 15–25 were 34–47 per 10,000 person-years at 10 years, which was higher than the absolute risks of women in the general population between 50 and 54 years, the typical age at which screening mammography is recommended (Hodgson et al. 2007).
The increasing awareness of the substantial excesses of breast cancer after therapy for HL at a young age has prompted a need for informed counseling. Estimates of the cumulative absolute risk of breast cancer among young women treated for HL at age 30 years or younger, however, have varied widely ranging from 4.2 to 34 % at 20–25 years after treatment (Bhatia et al. 2003; Swerdlow et al. 2000; Aisenberg et al. 1997; Sankila et al. 1996). Most estimates have not taken into account the influence of alkylating agent therapy, which can reduce breast cancer risk (Travis et al. 2003; van Leeuwen et al. 2003), or the effect of competing causes of mortality (Gooley et al. 1999). Accurate projections of breast cancer risk, as available for women in the general population (Gail et al. 1989), are important to predict the disease burden among the growing population of HL survivors treated with past regimens and to facilitate the development of risk-adapted long-term follow-up recommendations. Estimates of the cumulative absolute risk of breast cancer for women treated for HL at age 30 years or younger were recently provided in terms of measures of radiation dose and chemotherapy recorded in medical charts (Travis et al. 2005
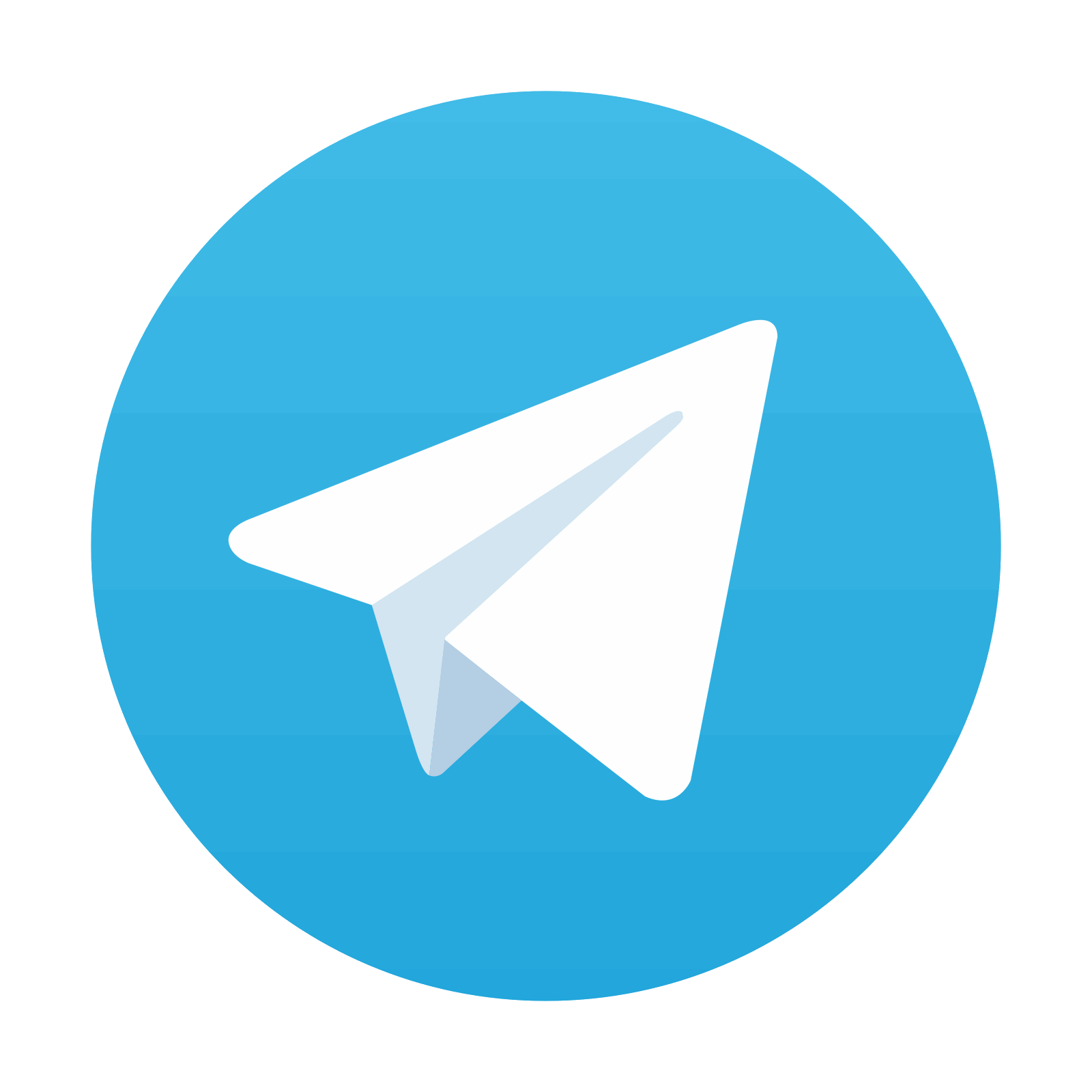
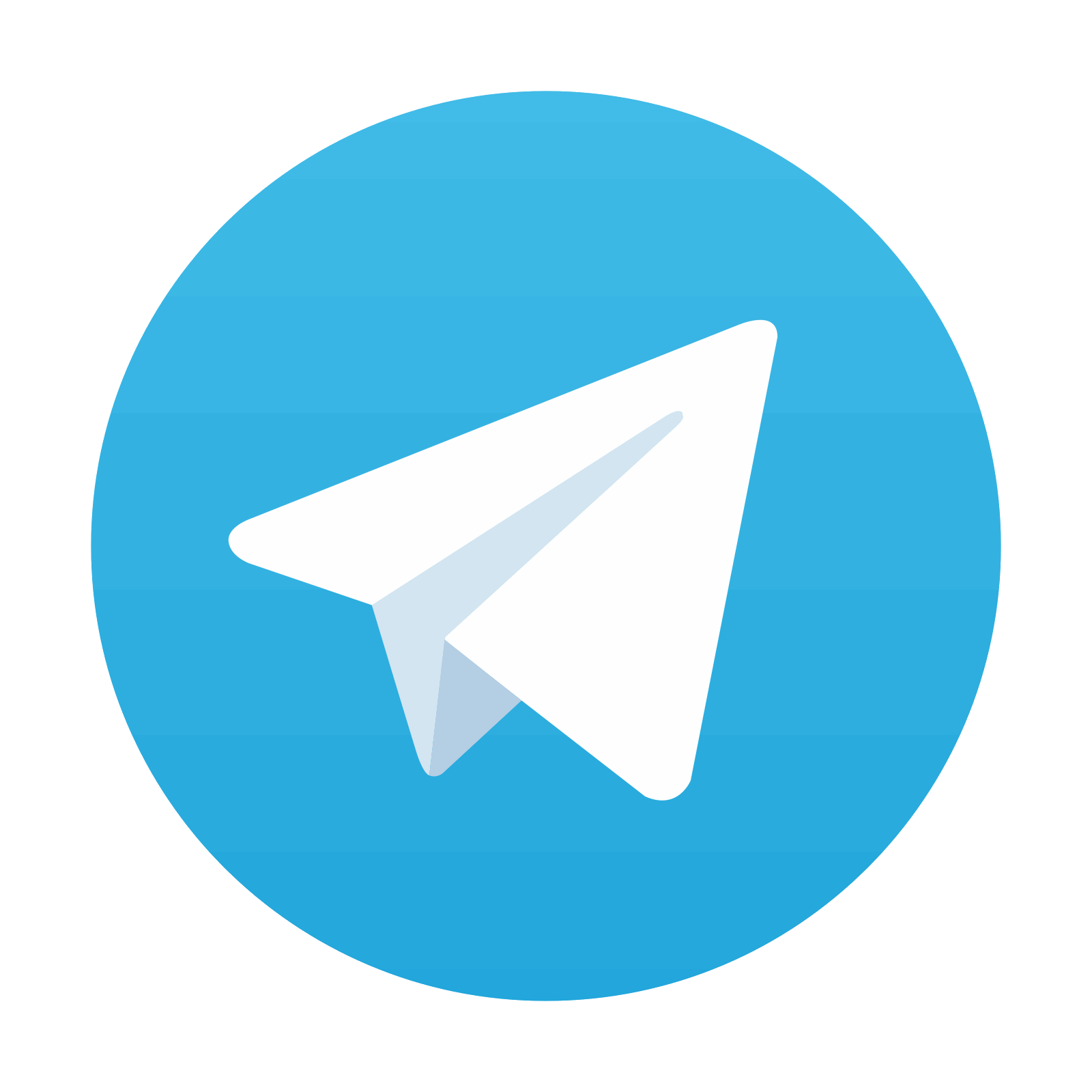
Stay updated, free articles. Join our Telegram channel

Full access? Get Clinical Tree
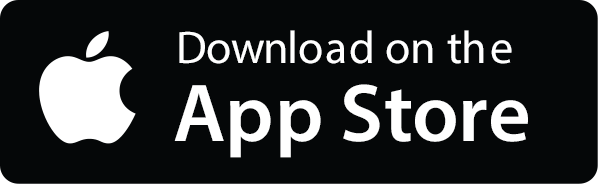
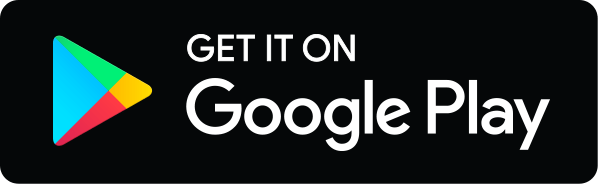