Fig. 4.1
Normal right hip X-ray: femoral shaft axis (red line), lateral offset (blue line), body weight lever arm (green line)
4.3 Knee
Knee motion is more complex than simple flexion and extension; it occurs in flexion and extension, abduction, and adduction, rotation about the long axis of the limb, with a rollback of femoral condyles during flexion [4]. The main factor in the short survivorship and early failure of many knee prosthesis designs should be referred to poor attention to these complex knee motions and their attendant complex stresses. Knee motion is determined by both the joint geometry of the knee and the ligamentous restraints; important evaluations are addressed to the role of posterior cruciate ligament (PCL) in total knee arthroplasty.
The arguments in favor of PCL retention are the greater potential range of motion with effective femoral rollback and the advantage of ligament’s function as a restraint to translational displacement of the knee [5]. In PCL-substituting knee prostheses, displacement must be resisted by the prosthetic geometry, with resultant stress transferred to the bone–cement interface; it could suggest higher failure rates in PCL-substituting designs than in PCL-retaining implants because of aseptic loosening. Another argument in favor of PCL retention centers on preservation of PCL proprioceptive role; more physiological and symmetrical gait is observed in patients with PCL-retaining prostheses than with PCL-substituting designs. PCL sparing is also correlated to better function of the patellofemoral joint; PCL-retaining designs do not tolerate much variation in the level of the preoperative joint line while balancing the flexion and extension gaps. Joint line elevation may alter patellofemoral mechanics and result in knee pain and subluxation in postoperative period. Lower alterations are recorded in the relationship of the patella to the joint line with PCL-retaining prostheses. Compelling arguments support PCL substitution. Many authors argue that the PCL is diseased with various forms of contracture; it results in much more difficulties in ligamentous balance. It is unlikely to reproduce normal PCL strain and function in a PCL-retaining knee arthroplasty.
Obtaining a reliable balance of the PCL influences implant survivorship; a too tight PCL in flexion can limit postoperatively the extent of flexion and lead to excessive femoral rollback, which could accelerate posterior tibial polyethylene wear. On the contrary, a too loose PCL may not provide adequate femoral rollback during flexion. It is fundamental to underline the deleterious effect of excessive femoral rollback on component fixation. With knee motion and variation of the point of tibiofemoral, the tibial components alternately undergoes maximal compression anteriorly and posteriorly [6].
Polyethylene wear may be greater with PCL-retaining designs; the tibial articular surface of PCL-retaining implants must be less congruent to the femoral component in the sagittal plane in order to allow femoral rollback. This less-conforming geometry is responsible for higher tibial contact stresses in PCL-retaining prostheses; these could be responsible of accelerated polyethylene wear.
One more argument in favor of PCL substitution is that surgical exposure is simpler with PCL excision; significant deformities can be more easily corrected, especially in stiff knees.
Another factor, which affects knee arthroplasty survivorship, is the restoration of correct limb alignment; malalignment of total knee prostheses seems to be implicated in long-term complications, including tibiofemoral instability, patellofemoral instability, stiffness, accelerated polyethylene wear, and implant aseptic loosening. Normal anatomical axis of the femur and the tibia form a valgus angle of 6 ± 2°: Varus or valgus deformity can be determined on the antero-posterior roentgenogram. The mechanical axis of the lower limb is defined as the line on a standing long leg antero-posterior radiogram projecting from the center of the femoral head to the center of the talar dome. This mechanical axis typically projects through the center of the knee joint (neutral mechanical axis) (Fig. 4.2). The knee is in valgus alignment if mechanical axis lies to the lateral side of the knee center. In mechanical varus alignment, the mechanical axis of the limb lies to the medial side of the knee center.
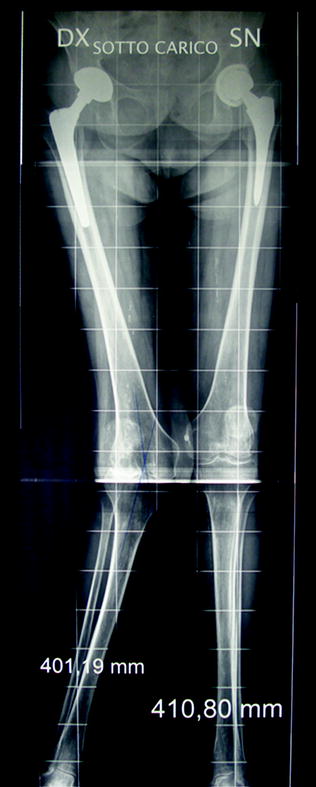
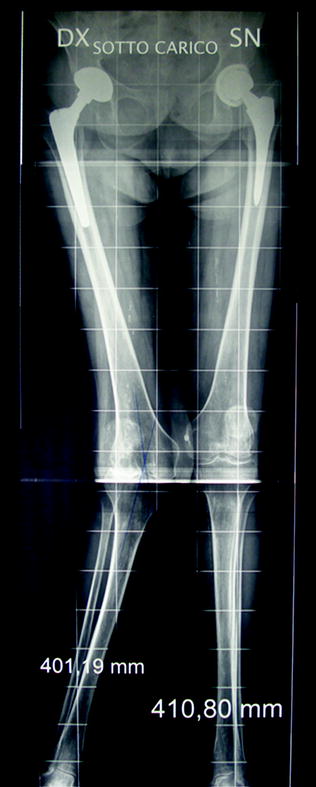
Fig. 4.2
Lower limbs radiograph showing severe right valgus knee deformity in a female patient who underwent bilateral total hip arthroplasty
In knee arthroplasty, the tibial components are implanted perpendicular to the mechanical axis of the tibia in the coronal plane, and the femoral component usually is implanted in 5–6° of valgus, in order to reestablish a neutral mechanical axis of the limb. The rotation of the femoral component influences not only the flexion space but also the patellofemoral tracking. [7]
The primary function of the patella is to increase the lever arm of the extensor mechanism of the knee, improving the efficiency of quadriceps contraction. The modern design of the trochlea in femoral components has been focused on the 1990s. In general, the trochleas have been deepened to resist patellar subluxation with greater areas of contact throughout the range of motion, and lateral flange of the trochlea has been made more prominent.
Patellofemoral stability is guaranteed by a combination of the articular surface geometry and soft tissue restraints. The Q-angle, described by Hvid, is the angle between the anatomical axis of the femur and the line between the center of the patella and the tibial tubercle. Knees with larger Q-angles have a greater tendency for lateral subluxation of patella. Lateral subluxation of the patella in early flexion is resisted primarily by the vastus medialis obliquus fibers because the patella does not contact strictly the trochlea. As the flexion increases, the trochlea and knee constraints play a dominant role in preventing subluxation.
Knee prostheses designs, as well as attention to components implant, reproduction of preoperative joint line height, have improved arthroplasties performances and have decreased significantly the rate of failure.
4.4 Tribology of Joint Prostheses
Tribology is defined as the science of interacting surfaces in relative motion and all effects related. The study of wear, friction, and lubrication belongs to tribology. Low friction, optimal motion, and good transmission of joint loads are important design objectives for prosthetic joints for different reasons. If large shear forces because of high friction are applied to the articular surfaces, the risk of loosening may increase. Increased frictional shear results in stresses which damage surface; it can produce higher release of wear debris to the surrounding tissue that also increases the risk of loosening.
4.5 Friction
Friction arises from the interaction between moving solids in contact. The friction force is proportional to the load applied to the surface and the coefficient of friction.
The coefficient of friction is the measure of the resistance offered in moving one object over another. It is influenced by different factors: materials and finish of the surfaces of the materials, the load applied on surfaces, the temperature and presence of a fluid as a lubricant.
Normal joints have a coefficient of friction estimated as 0.008–0.02. Several studies demonstrated that the coefficient of friction produced by metal on high-density polyethylene is approximately 0.02, metal-on-metal joints approximately 0.8, and ceramic on ceramic and ceramic on polyethylene are also low [8]. Instead, metal on ceramic produces higher coefficient of friction.
A frictional torque force is produced when the artificial joint moves through an arc of motion. It depends on the frictional forces and by the distance a point on the joint surface moves during an arc of motion. The frictional forces are influenced by the coefficient of friction and the joint surface contact area [9]. Low coefficient of friction is a fundamental element in modern prostheses: Frictional forces are transmitted to the acetabular cup and the femoral stem, the cement–prosthesis or implant–bone interface as forces that lead to destabilize the components. Increasing in frictional torque force should be involved in loosening of prosthesis and in high wear rate.
4.6 Lubrication
Friction can be reduced by lubrication: Interposition of a fluid material between two contacting solids can minimize interaction between them. The extent of fluid film plays an important role in the wear process of artificial joints in vivo. The effectiveness of a lubricant film is defined by film thickness which is dependent on the viscosity of the lubricant, the relative velocity between surfaces, the pressure across the interface, and the surface roughness.
When the thickness of the fluid film is less than or equal to the average surface roughness of the articulating surfaces, boundary lubrication is achieved. This is not an ideal phase; the asperities of the articulating surfaces are in contact. It occurs in rough bearing surfaces, or as a result of third-body formation or protein deposition.
It is improved with better manufacture tolerances of the bearing surfaces. As the implant remain in situ and works, as the surface asperities decrease, as well thickness of the fluid film increases and the articulating surfaces become separated from each other.
The wettability of the material also plays a part in lubrication. This is essentially related to how hydrophobic or hydrophilic it is. The ceramics are the most wettable of the currently used bearings [10].
4.7 Wear
The wear can be defined as the loss of material from the surfaces of the prosthetic joint due to the motion; material is lost in particulate debris. Three types of wear are distinguished: (a) abrasive, the harder material produces furrows in the softer material; (b) adhesive, the softer material is transferred as a thin film onto the harder one, and (c) fatigue, in which repetitive loading produces subsurface cracks or sheets of material delaminated from the surface. The term “three-body wear” refers to a specific mechanism of abrasive wear caused by retention of debris particles between the sliding joint surfaces; these generate additional stress concentrations of the articulating surfaces [11].
Wear is actually considered one of the most significant factors limiting the longevity of total joints. The most important mechanism in total hip arthroplasties is abrasive and adhesive wear; fatigue wear appears more important in total knee arthroplasties.
The wear is determined by several factors: (a) the coefficient of friction of the materials, (2) the hardness of the materials, (3) the applied load, (4) the sliding distance for each cycle, depending on joint range of motion, and (5) the number of cycles that occur over time [9].
Modern prostheses have very smooth articular surface, but they still present grooves and peaks. The size of the asperities depends on the material and the surface finishing. When the two surfaces slide initially against each other under load, many of asperities are removed, producing high initial wear rate referred to as the “wearing-in” period. The contact area surfaces gradually adapt to each other and the wear rate decreases, reaching a steady state.
Particulate wear debris produces concerning biological consequences which can lead to implant failures because of potential adverse tissue response. Particles consistent with polyethylene debris are routinely identified in the membranes surrounding failed joint replacements. Many authors demonstrated the relationship between the depth of socket wear and the incidence of prostheses components loosening and migration. It is proved that loosening of prosthesis components is the direct result of wear debris macrophage-induced bone resorption at the implant–bone interface [12].
Some knowledge of the characteristics of the various materials and their effects to bone mass is essential for the orthopedic surgeon performing total joint arthroplasties.
Materials properties employed in the manufacturing of bearing surface in joint prostheses have a paramount role in implant performance and survivorship. These include corrosion resistance, strength, ductility, hardness, and particularly frictional characteristics. Frictional characteristics are a result of material wettability, manufacturing variables such as surface finish, and operating conditions such as lubrication. Frictional forces depend on both the material composition and roughness, because both chemical and mechanical interactions may occur.
Osteolysis is a significant cause of aseptic loosening and is cause for the majority of revision surgery. It is due to resorption of bone observed around the prosthetic components. There is a relation between wear and debris formation; osteolysis is reported more commonly in patients with high wear of their implants. These increased osteoclasts activity is induced by immunological response to different debris [13].
There has long been interest in developing bearing materials that exhibit friction and wear behavior similar to that of articular cartilage. Cartilage is an excellent compliant bearing capable of large deformation without failure and low-friction coefficient in synovial joints, less than 0.01.
It appears more than mandatory for physician to have essential knowledge of bearing materials properties in prosthetic implant.
4.7.1 Polyethylene
Polyethylene wear is particularly recognized as a major element limiting the survivorship of arthroplasties; current researches about materials are directed toward strategies to reduce wear [14]. The physical properties of polyethylene affect its rate of wear; molecular weight is correlated with the wear resistance. Manufacturing and sterilization methods may influence molecular weight; oxidation of the polymer before implantation and in vivo contributes to reducing molecular weight. Future improvements in fabrication and sterilization processes may aid in reducing wear rate of polyethylene. Sterilization by autoclaving or by irradiation in the presence of oxygen produces free radicals in the material, exposing the polyethylene to oxidation and making it more susceptible to wear. Actually, polyethylene sterilization is carried out by gamma radiation in an inert environment, typically in nitrogen gas or vacuum [15].
Considerable interest is oriented actually on the improved wear characteristics of highly cross-linked polyethylene [16]. Despite continuing research improving implant materials, the combination of metal articulating with ultrahigh molecular weight polyethylene (UHMWPE) remains the most commonly used.
It is a tough, extremely chemically inert plastic with good resistance to creep and no biological toxicity.
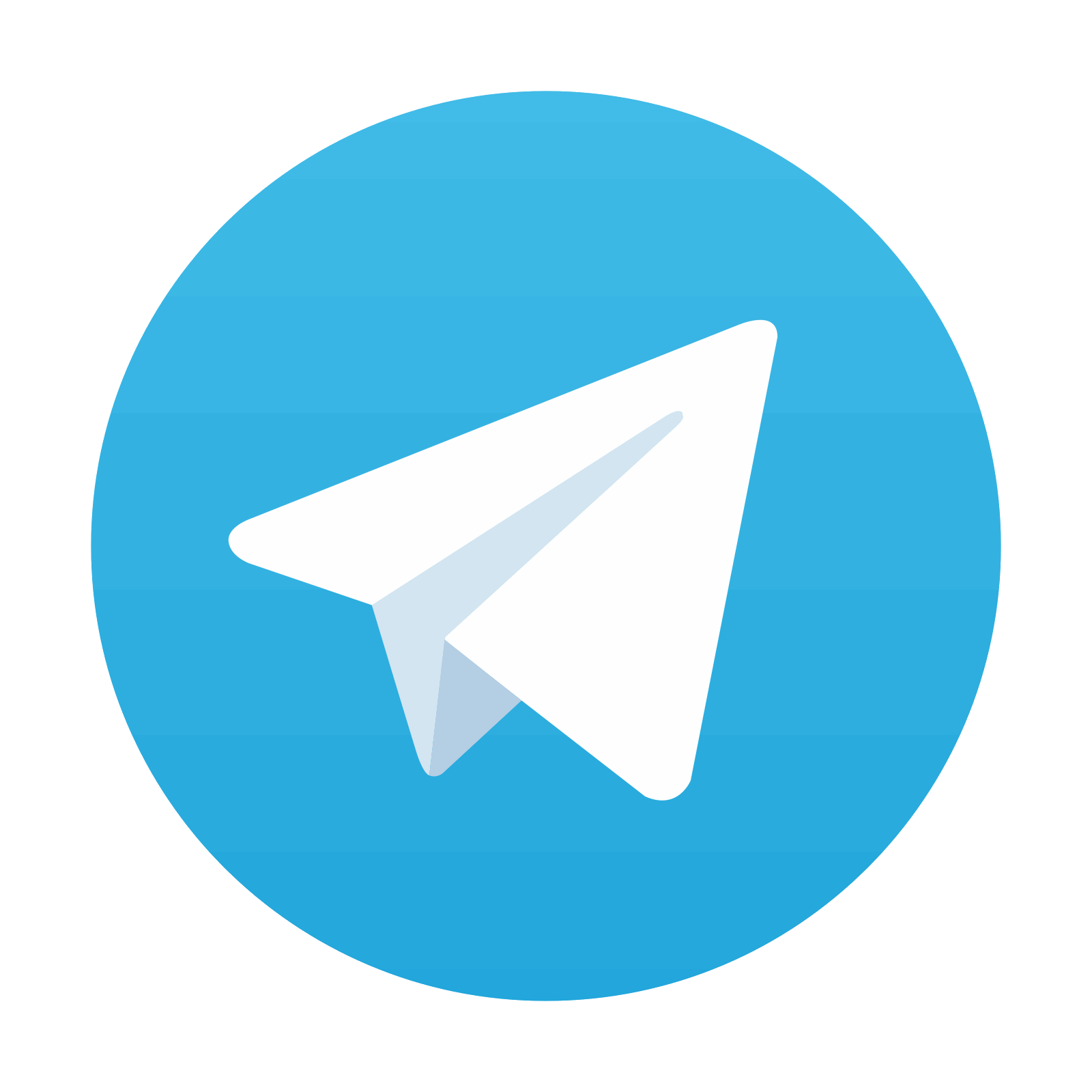
Stay updated, free articles. Join our Telegram channel

Full access? Get Clinical Tree
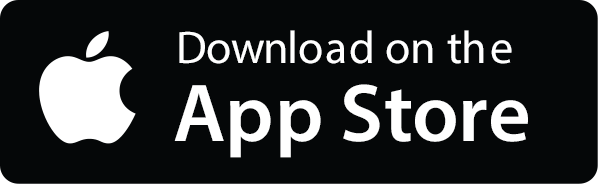
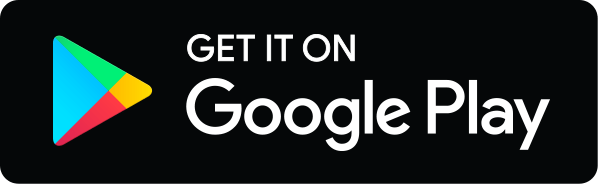