Fig. 1.1
Specific gene expression during osteoblast-to-osteocyte ontogeny. Different stages of osteoblast differentiation, from mesenchymal stem cells to mature mineral matrix-embedded osteocytes, are illustrated. Specific genes involved in both osteoblastogenesis and osteocytogenesis are schematically summarized
1.1.2 Transcriptional Regulation of Osteoblast Differentiation
The differentiation of osteoblasts from mesenchymal progenitors, which also originate chondrocytes, myoblasts, from adipocytes, and tendon cells, requires the activity of specific transcription factors that are expressed at distinct time points during the differentiation process, thereby defining various developmental stages of the osteoblast lineage.
Runt domain–containing transcription factor (Runx2 ) is a master switch for osteoblast differentiation. Levels of Runx2 gradually increase in subsequent stages of osteoblast differentiation, with maximum expression observed in the mature osteoblasts. Homozygous deletion of Runx2 in mice resulted in a complete lack of osteoblasts [7]. Runx2 is both necessary and sufficient for mesenchymal cell differentiation toward the osteoblast lineage [8]. It was demonstrated that Runx2 controls bone lineage cells by binding to the Runx regulatory element in promoters of osteoblastogenic genes. Runx2 target genes include genes expressed by both immature and differentiated osteoblasts. Such as TGF-β receptor, ALP, collagen type I alpha 1 and alpha 2 chain, OPN, OSTC, Vitamin D receptor, BSP, ON, and collagenase [9]. Thus, Runx2 is necessary for both osteoblast differentiation and function.
Osterix is another DNA-binding transcription factor that is absolutely required for osteoblast differentiation. Osterix/SP7 is a member of the zinc-finger-containing SP family and is abundantly expressed throughout osteoblast differentiation. Genetic inactivation of Osterix in mice results in the absence of mineralized bone matrix, defective osteoblasts, and perinatal lethality [10]. Similar to Runx2, forced expression of Osterix in non-bone cells, promotes expression of both early and late marker genes of osteoblasts. However, molecular and genetic studies revealed that Runx2 is expressed in mesenchymal tissues of Osterix null mice [10]. Thus, Osterix acts downstream of Runx2 in the transcriptional cascade of osteoblast differentiation. Consistently, Osterix expression is positively regulated by direct binding of Runx2 to a responsive element in the promoter of the Osterix gene.
Activating Transcription Factor 4 (ATF4 ), a member of the basic Leu zipper family of transcription factors, has important roles in the more mature osteoblast lineage cells. Misregulation of ATF4 activity has been linked with the skeletal abnormalities seen in human patients with Coffin–Lowry syndrome and neurofibromatosis type I [11]. ATF4 may function in osteoblast lineage cells through two distinct mechanisms. First, it directly regulates the expression of OSTC and RANKL [11]. Second, ATF4 promotes efficient amino acid import to ensure proper protein synthesis by osteoblasts [11].
Activator Protein 1 transcription factor family (AP1 ) is composed of heterodimers of Fos-related factors (cFos, Fra1, Fra2, and FosB) and Jun proteins (cJun, JunB and JunD). Multiple Fos and Jun proteins are highly expressed in proliferating osteoprogenitors. Their expression decreases during differentiation, except for Fra2 and JunD that are the primary AP1 components present in mature osteoblasts [12]. Some observations indicated that c-Fos [12], Fra1, ΔFosB (an alternative splice variant of FosB) [12], and JunB [13] proteins promote bone formation. In contrast, JunD promotes a decrease in bone mass repressing other AP1 protein expressions in osteoblasts [14]. A number of direct targets of AP1 in osteoblasts have been identified, and include the OSTC, collagenase-3, BSP, and ALP promoters [12].
1.1.3 Regulation of Osteoblast Differentiation by Secreted Molecules
Skeletal cells produce numerous growth factors and cytokines that signal in both autocrine and paracrine way to control cell proliferation, differentiation, and survival. These secreted factors and signaling pathways either promote or suppress the expression of transcription factors essential for osteoblast differentiation.
Transforming Growth Factor–β (TGF-β) is one of the most abundant cytokines in bone matrix and plays a major role in development and maintenance of the skeleton, affecting both cartilage and bone metabolism [15]. By binding to its specific receptors, TGF-β induces activation of Smad-2 and Smad-3 intracellular proteins that transduce extracellular signals from TGF-β to the nucleus, regulating osteoblast gene expression [16]. TGF-β is important in the maintenance and expansion of the mesenchymal stem/progenitor cells. TGF-β signaling also promotes osteoprogenitor proliferation, early differentiation and commitment to the osteoblastic lineage through the Smad2/3 pathways, and the cooperation between TGF-β and PTH, Wnt, BMP, as well as FGF signaling.
Bone morphogenetic proteins (BMPs) belonging to the TGF-β superfamily were originally identified as the active components in bone extracts capable of inducing ectopic bone formation. BMPs are expressed in skeletal tissue and are required for skeletal development and maintenance of adult bone homeostasis and play an important role in fracture healing [17]. Genetic studies have demonstrated an important role for BMP2 and BMP4 in promoting osteoblast differentiation and function [18]. Recent studies have also shown that BMP3 inhibits the signal transduced by BMP2 or BMP4 [19], working as a negative regulator of osteoblast differentiation.
The Wingless (Wnt ) family of glycoproteins has recently emerged as central regulators of bone mass. Upon engaging various membrane receptors, Wnt ligands activate numerous intracellular pathways that are either dependent or independent on β-catenin. In the β-catenin-dependent signaling, Wnt binds to Frizzled receptors and their co-receptors low-density lipoprotein receptor-related protein 5 or 6 (LRP5/6) to stabilize cytosolic β-catenin that enters the nucleus and stimulates the transcription of Wnt target genes such as Runx2 and Osterix [20]. Thus, activation of the canonical pathway promotes the differentiation of osteoblast progenitor cells into mature osteoblasts. Wnt signaling is tightly regulated by a delicate balance of extracellular agonists and antagonists. There are different antagonists, such as soluble frizzled-related proteins (sFRPs), which inhibit Wnt signaling by binding to and sequestering Wnt ligands and others belonging to the Dickkopf (DKK), family members or the SOST gene product (sclerostin) that bind to and sequester the Wnt co-receptors LRP5/6 [21]. In human, receptor mutations that render Wnt signal constitutively active result in a generalized increase in bone mass [22]. Loss-of-function mutations in the gene encoding the Wnt co-receptor LRP5 cause osteoporosis-pseudoglioma syndrome [23], a form of juvenile-onset osteoporosis. Conversely, mutations in LRP5 that inhibit the interaction between the co-receptor and DKK1 or sclerostin cause high-bone mass syndrome [24, 25]. In addition, loss-of-function or loss-of-expression mutations in SOST result in the bone-thickening diseases, sclerosteosis or Van Buchem disease, respectively [26, 27]. β-catenin-independent Wnt signaling has also been implicated in promoting osteoblast differentiation [28]. In particular, Wnt5A is thought to promote osteoblast differentiation by inhibiting the activation of adipogenic genes [29]. Thus, both β-catenin-dependent and β-catenin-independent Wnt signaling are able to control differentiation of osteoblast progenitors into mature osteoblasts.
Fibroblast Growth Factors (FGFs ) are a large family of proteins (23 different ligands) that transduce their signal through one of the four FGF receptors (FGFR). FGFs initiate condensation of the mesenchyme and proliferation of progenitor cells. In particular, FGF2 is important for preosteoblast proliferation and maturation [30], while FGF18 is essential in mature osteoblast formation [31].
1.2 Osteocytes
Osteocytes account for about 95 % of cells in the mature bone tissue. They are entombed individually in lacunae of the mineralized matrix and have cytoplasmic processes that radiate from the cell body and give osteocytes the aspect of neuronal cells. The processes run along canaliculi and are linked by gap junctions with the processes of neighboring osteocytes, as well as with cellular elements of the bone marrow, endothelial cells of the bone marrow, and bone surface cells including the lining cells. In contrast to osteoclasts and osteoblasts, which are relatively short-lived and transiently present only on a small fraction of the bone surface, osteocytes are long-lived cells and are deployed throughout the skeleton.
Nowadays, osteocytes are considered the choreographers of the remodeling process. They are able to direct the homing of osteoclasts to the site that is in need of remodeling [32] and to control and modify mineralization of the matrix produced by osteoblasts [33]. Moreover, they produce factors that influence mineral homeostasis and also mediate the homeostatic adaptation of bone to mechanical loading. Osteocytes are considered mechanosensory cells because of their peculiar location in bone and complex dendritic network. It was demonstrated that osteocyte may sense load through the cell body, the dendritic processes and the cilia [34]. Moreover, it was demonstrated that 20 % of the osteocytes are active in bone formation [35] and that could surprisingly directly remove minerals from their lacunae and canaliculi, playing a role in mineral homeostasis during a calcium-demanding condition such as lactation [36]. Literature data also demonstrate the ability of osteocytes to negatively regulate osteoblast formation and activity through the secretion of sclerostin, a well known Wnt inhibitor [37]. Recently, the ability of osteocytes to influence osteoclast formation through the secretion of the osteoclastogenic cytokine RANKL was also showed [38].
1.2.1 Osteocyte Differentiation
It has been known that osteocytes are derived from osteoblasts. However, the precise mechanisms by which an osteoblast becomes an osteocyte remain elusive. At the end of the bone formation phase, osteoblasts can either become embedded in bone as osteocytes, become inactive osteoblasts or bone lining cells, or undergo programmed cell death (apoptosis) [39]. It remains unclear whether osteocyte formation is a process induced by a specific pattern of gene expression in a subset of osteoblasts and also whether this is a cell autonomous response rather than a response modulated via the surface cells receiving signals from already embedded osteocytes. It is also not known whether every osteoblast has equal possibilities of becoming an osteocyte or whether there are specific subpopulations with predefined fates.
Osteocytogenesis is now considered an active rather than a passive process that includes different changes in cellular features. The formation of dendritic processes and the reduction in cytoplasmic volume take place in the embedding cell (Fig. 1.1). Generation of cell processes such as lamellipodia, pseudopodia, and dendrite formation is a dynamic event that required the cleavage of collagen and other matrix molecules [40]. Thus, the cell undergoes a dramatic transformation from a polygonal cell to a cell extending polarized dendrites toward the mineralizing front, which is followed by dendrites extending toward the vascular space or bone surface. Once embedded in bone, the osteocyte appears to maintain its polarity with regard to the directionality of its dendrites.
1.2.2 Osteocyte-Specific Genes
Within the past two decades, a lot of osteocyte markers have been identified. In particular, early embedding young osteocytes are high producers of E11/gp38 (also known as podoplanin), a protein that play an important role in dendrite formation (Fig. 1.1). In fact, it was demonstrated that conditional deletion in bone cells in vivo resulted in decreased canaliculi and increased trabecular bone [41]. Controversially, more mature, deeply embedded osteocytes express high levels of sclerostin, the protein product of the SOST gene [37] known to be a negative regulator of bone formation (Fig. 1.1). Mutation of SOST causes high bone mass in humans and deletion results in high bone mass in mice [42].
It was also demonstrated that as the osteoblast transitions to an osteocyte, ALP is reduced, and OSTC and casein kinase II, a serine/threonine protein kinase that controls the mineralization process, are elevated [41].
Several osteocyte-specific markers play critical roles in phosphate homeostasis. These include phosphate-regulating gene with homologies to endopeptidases on the X chromosome (PHEX), matrix extracellular phosphoglycoprotein (MEPE), dentin matrix protein 1 (DMP-1), and fibroblast growth factor 23 (FGF-23) [41] (Fig. 1.1). Autosomal recessive mutations in DMP-1 in humans provoke hypophosphatemic rickets. DMP-1-null mice have a similar phenotype to Hyp mice carrying a PHEX mutation that of osteomalacia and rickets owing to osteocyte elevated FGF-23 levels. Both DMP-1 and PHEX appear to down-regulate FGF-23 expression, which allows resorption of phosphate by the kidney, thereby maintaining sufficient circulating phosphate levels [43]. Thus, osteocyte network can function as an endocrine system, targeting distant organs such as kidney.
Moreover, osteocytes, under certain conditions such as lactation, can also express osteoclast markers such as tartrate-resistant acid phosphatase and cathepsin K, in order to remodel their perilacunar matrix [36].
1.2.3 Osteocyte Cytoskeletal Components
Cytoskeleton components and/or molecules involved with cell motility are expressed in a different way in osteocytes compared to osteoblasts. The previously cited E11/gp38 is thought to regulate actin cytoskeleton dynamics, and, in particular, it was suggested that it may play an important role in dendrite osteocyte formation. Moreover, osteocytes are also enriched with CapG and destrin molecules, all necessary for cytoskeletal rearrangement (Fig. 1.1). Differences in distribution of the actin-binding proteins, villin, fimbrin, filamin, and spectrin, have been demonstrated during osteocyte differentiation. An organized expression of tubulin, vimentin, and actin in cell bodies and dendrites of osteocytes, crucial to maintain osteocyte dendritic morphology, was also described [41].
Interestingly, an increase in dendritic processes number in the bone of adult rats compared with juvenile ones was also demonstrated, suggesting that the internal structure of compact bone changes with age [44].
1.3 Osteoclasts
Osteoclasts are terminally differentiated multinucleated cells that are the principal resorptive cells of bone, playing a central role in the formation of the skeleton and regulation of its mass [45, 46]. The osteoclasts are usually found in contact with a calcified bone surface and within a lacuna (Howship’s lacunae) that is the result of its own resorptive activity. The zone of contact with the bone is characterized by the presence of a ruffled border, which consists of complex folds of plasma membrane juxtaposed to bone and surrounded by the actin ring or sealing zone. The ruffled border and the sealing zone appear only in osteoclasts adherent to the mineralized matrix and in a resorptive state. The sealing zone is formed by a ring of focal points of adhesion (podosomes) with a core of actin and several cytoskeletal and regulatory proteins around it that attach the cell to the bone surface, thus sealing off the subosteoclastic bone-resorbing compartment. The attachment of the cell to the matrix is performed via integrin receptors, which bind to specific RGD (Arginine-Glycine-Aspartate) sequences found in matrix proteins, and the αvβ3 is the integrin predominantly expressed by osteoclasts. The plasma membrane in the ruffled border area contains proteins that are also found at the limiting membrane of lysosomes and a specific type of electrogenic vacuolar proton ATPase involved in acidification. The basolateral plasma membrane of the osteoclast is specifically enriched with Na+, K+-ATPase, HCO3 −/Cl−, and Na+/H+ exchangers [47]. Lysosomal enzymes such as tartrate-resistant acid phosphatase and cathepsin K are secreted, via the ruffled border, into the extracellular bone-resorbing compartment and are involved in bone matrix digestion.
1.3.1 Osteoclast Differentiation
The osteoclast derives from cells in the mononuclear phagocyte lineage. Their differentiation requires the transcription factors PU-1 and MiTf at early stages, committing the precursors into the myeloid lineage. Macrophage colony-stimulating factor (MCSF) is then required to engage the cells in the monocyte lineage and ensure their proliferation and the expression of the RANK receptor. At that stage, the cells require the presence of RANKL, a member of the TNF family of cytokines produced by stromal cells, to truly commit to the osteoclast lineage and progress in their differentiation program. This step also requires expression of TRAF6, NFκB, c-Fos, and NFATc1, all downstream effectors of RANK signaling (Fig. 1.2).
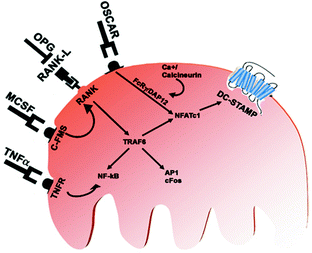
Fig. 1.2
Extracellular ligands, receptors, and intracellular signals activated during osteoclast differentiation
1.3.2 Essential Factors Regulating the Development of Osteoclasts
MCSF is a homodimeric glycoprotein, produced by osteoblasts and bone marrow stromal cells, that binds to high-affinity receptors (c-Fms) expressed on cells of the monocyte/macrophage lineage. Homozygous disruption of MCSF coding sequences in osteopetrotic (op/op) mice severely impairs production of macrophage populations underlying the importance of MCSF for their development [48].
OPG . In 1997, Simonet et al. [49] reported the discovery of OPG, a member of the TNF receptor family that inhibited bone resorption. Tsuda et al. in 1997 [50] independently isolated the same protein [called “osteoclastogenesis inhibitory factor (OCIF)”] as a heparin-binding protein from the conditioned media of human fibroblast cultures and showed that its cDNA sequence was identical to that of OPG [51]. The physiological roles of OPG have been studied in OPG-deficient mice produced by targeted disruption of the gene: OPG knock-out mice were viable and fertile, but they exhibited severe osteoporosis caused by enhanced osteoclast formation and function. Moreover, destruction of growth plates and lack of trabecular bone with an increase in the number of osteoclasts were detected in long bones of these adult mice, and the strength and mineral density of their bones were dramatically decreased [52]. These results have indicated that OPG is a physiological regulator of osteoclast-mediated bone resorption during postnatal bone growth. The discovery of OPG led to understand which was the counterpart molecule acting to induce osteoclastogenesis. At the beginning, it was called osteoclast differentiation factor (ODF) or OPG-Ligand, since it was supposed to bind and block the activity of OPG; later, the molecular cloning of an isolated ODF/OPGL revealed that this molecule was identical to TRANCE (TNF-related activation induced cytokine) and RANKL, which were independently identified by other groups as a novel member of the TNF ligand family.
RANKL is a TNF superfamily cytokine and was originally identified as an activator of dendritic cells expressed by activated T cells [53]. Subsequent studies revealed RANKL to be a key cytokine for osteoclastogenesis [2, 54]. Targeted disruption of RANKL results in the defective formation of lymph nodes as well as severe osteopetrosis due to impaired formation of osteoclasts [55], indicating that this molecule is critical for both the immune and bone systems. The receptor for RANKL (RANK) is expressed in osteoclast precursor cells as well as in mature osteoclasts and dendritic cells. Targeted disruption of RANK also results in severe osteopetrosis [53], suggesting the RANK–RANKL interaction plays an essential role in osteoclast formation in vivo. OPG acts as a physiological inhibitor of RANKL [56], and the dynamic balance between RANKL and OPG regulates the levels of bone resorption.
The signals immediately activated by RANKL have been extensively studied. RANK contains three TRAF6-binding sites [57], and TRAF6-deficient mice exhibit severe osteopetrosis, indicating that TRAF6 is an essential mediator of RANK signaling during osteoclastogenesis. RANKL induces rapid activation of NF-kB, while such activation is severely abrogated in TRAF6-deficient cells [58]. NF-kB is a dimeric transcription factor complex composed of p65 (RelA), c-Rel, RelB, NF-kB1 (p50/p105), and NF-kB2 (p52/p100). Although p50– or p52-deficient mice have no obvious bone phenotype, mice doubly deficient in p50 and p52 develop osteopetrosis due to a defect in osteoclast differentiation, suggesting a critical role for NF-kB in osteoclastogenesis [59].
NFAT NF-kB and AP-1 are activated by RANKL. In the early phase and thus play an essential role in osteoclastogenesis, but these transcription factors are also activated by other cytokines, such as IL-1, which are not capable of inducing osteoclast differentiation per se
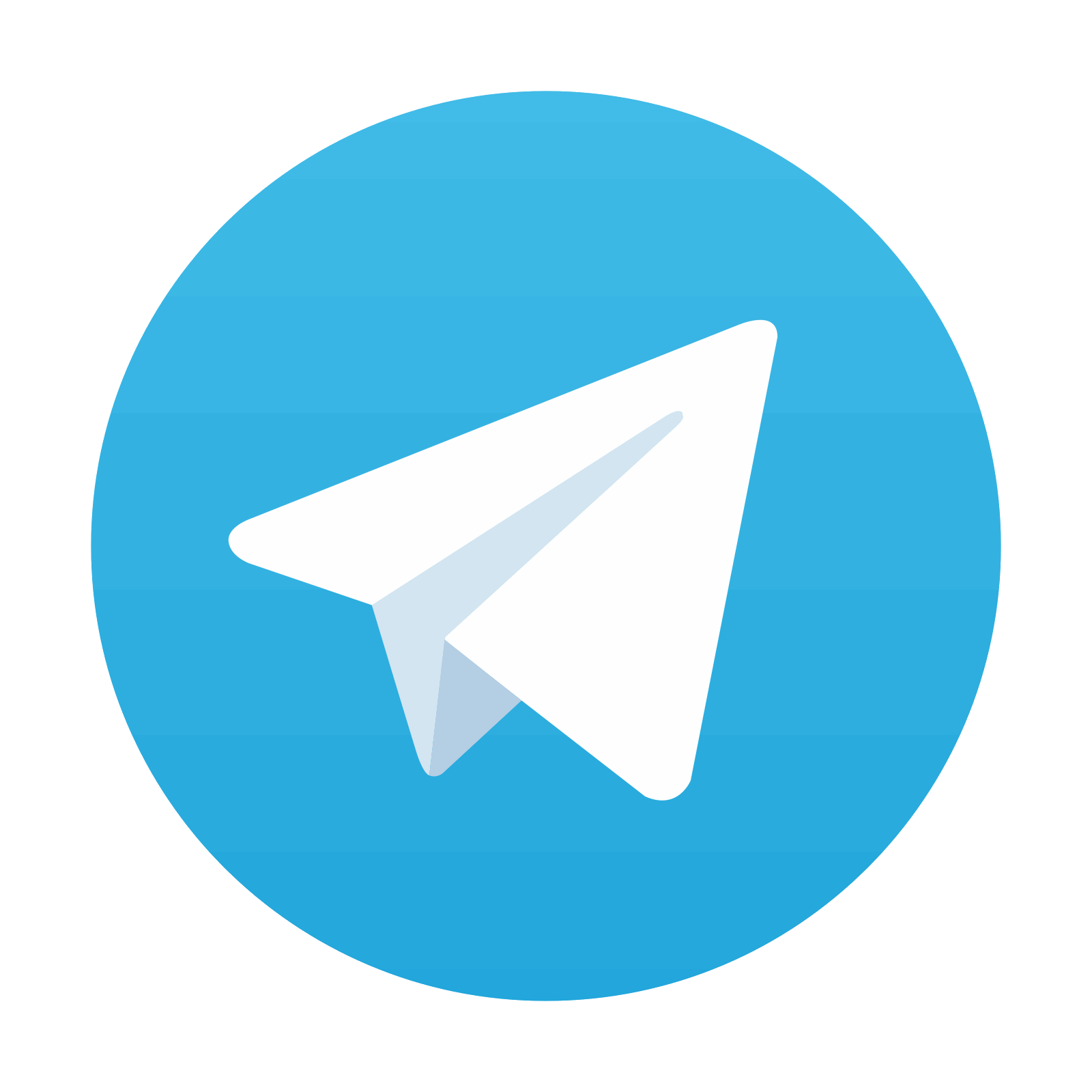
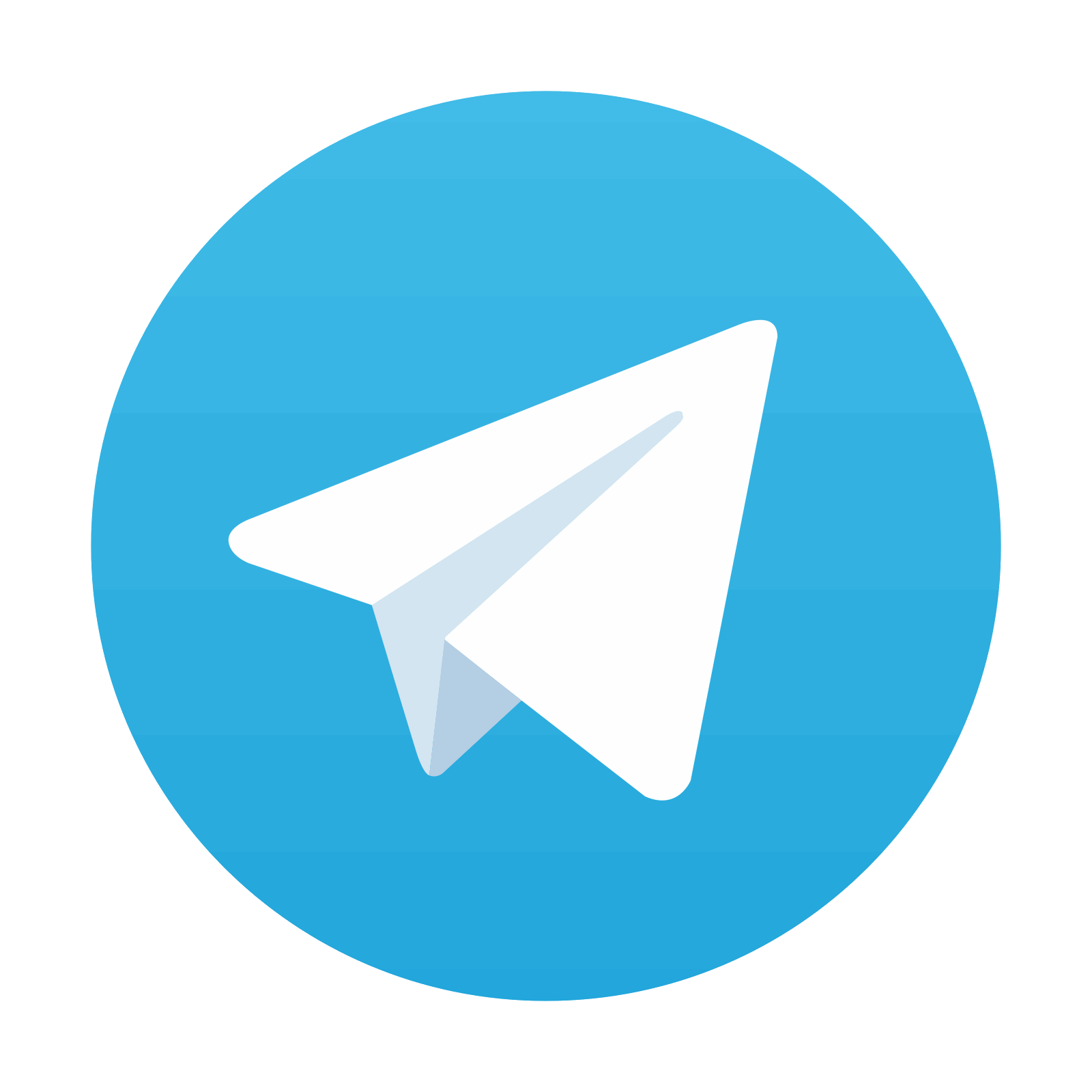
Stay updated, free articles. Join our Telegram channel

Full access? Get Clinical Tree
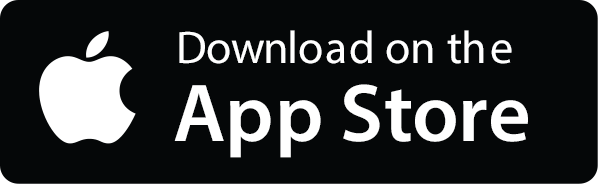
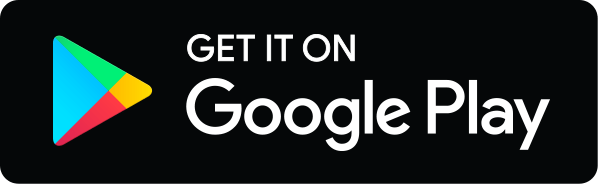