Protein
Function
Collagen type I
Serves as template for matrix deposition and mineralization; binds and orients other proteins that nucleate hydroxyapatite deposition
Gamma–carboxyglutamic acid–containing proteins(Gla proteins)
Osteocalcin
Role in osteoclast recruitment and maturation; it is often used as a marker for the bone formation process; considered as a core of the cross-talk between bone remodeling and glucose metabolism
Matrix Gla protein
Inhibitor of calcification
Glycoproteins
Osteonectin
Regulates calcium-mediated processes, cell–matrix interactions, hydroxyapatite binding sites, and bone remodeling
Alkaline phosphatase
Decreases the concentration of pyrophosphates (mineralization inhibitor) and increases the concentration of the inorganic phosphate (mineralization promoter)
Small integrin–binding ligand N–glycosylated protein (SIBLINGs)
Osteopontin
Mediates attachment of bone cells to mineral crystal structure; regulates bone resorption and bone calcification and is active in immunological reactions, tumorigenesis, and angiogenesis
Bone sialoprotein
Binds to cells; promotes the initial formation of hydroxyapatite crystal; stimulates bone resorption by inducing osteoclastogenesis and osteoclast survival and decreasing osteoclast apoptosis
Dentin matrix protein 1
Presents in three distinct segments that control the mineralization; acts as a transcriptional factor for activation of osteoblast-specific genes
Matrix extracellular phosphoprotein
Predominantly expressed by osteocytes; involved in the regulation of both osteoblast and osteoclast activities during bone remodeling, biomineralization, regulation of PHEX (phosphaturic hormone) activity
Other RGD–containing proteins
Fibronectin
Regulates mineralization binding to other matrix proteins such as collagen
Thrombospondins
Modulate cell–matrix interactions, bind to types I and V collagens; they are potent anti-angiogenic factors; TSP2 expression increases in preosteoblasts and decreases in mature osteoblasts
Vitronectin
Binds to collagen and to heparin and promotes cell attachment, spreading, and migration
Fibrillins
Confer limited elasticity to the bone matrix
Collagen type I (COLL-I) includes approximately 90 % of the entire collagen content of bone and about 80 % of the total proteins present in bone [1]. Other types of collagen, such as types III and V, are present at low levels in bone and appear to modulate the fibril diameter. COLL-I is a member of the wide collagen superfamily which serves as the main source of mechanical strength in connective tissue and the template for matrix deposition and mineralization in the bone [2]. Normal structural and functional COLL-I production and deposition in extracellular matrix need regulation at several steps, and defective synthesis in bone can cause human osteogenesis imperfecta [3]. The collagen molecules are derived from the aggregation of three polypeptide “α” chains, which have the characteristic Gly-XY repeating triplet (in which Gly is glycine, and X and Y are often proline and hydroxyproline) [4]. After their synthesis, each α chain becomes intertwined with two other chains in a triple left-handed helical structure. In particular, COLL-I, initially synthesized as procollagen-soluble precursor, is composed of two α1 chains and one α2 chain containing three distinct domains: a central triple-helical collagenous domain, and N- and C-terminal propeptides. Assembled procollagen trimers undergo several posttranslational modifications including hydroxylation, glycosylation, addition of mannose at the propeptide termini, and formation of intra- and intermolecular covalent cross-links. With the hydroxylation, the chains form a “nucleus” at the C-terminus where they assemble into a triple helix in a zipper-like fashion. This nucleation and “zippering” process must be highly orchestrated and depend on precise alignment of all the chains [5]. When procollagen molecules are secreted into the extracellular spaces, they are converted to collagen by proteolytic cleavage of the N- and C-terminal propeptides. The mechanism through which the collagen molecules assemble into fibrils is due to their spontaneous alignment. These fibrils provide tensile strength to bone and are composed of collagen helices that assemble parallel to each other in a regular quarter-staggered pattern, creating 68-nm gaps between adjacent collagen molecules. Hydroxyapatite crystals fill these gaps and are responsible for the compressive strength of bone [6].
Importantly, during the process of bone resorption, operated by osteoclasts, the release of small collagen fragments containing hydroxyproline occurs; thus, they can be detected in body fluids as a parameter of bone resorption activity.
2.1.1 Non-collagenous Proteins
Non-collagenous proteins represent 10–15 % of the total bone protein content. These proteins are multifunctional, having roles in organizing the extracellular matrix, coordinating cell–matrix and mineral–matrix interactions, and regulating the mineralization process. This large family of proteins will be discussed below and divided into gamma-carboxy glutamic acid-containing proteins, glycoproteins, small integrin-binding ligand N-glycosylated proteins, and other adhesive proteins containing RGD domain.
2.1.2 Gamma-Carboxy Glutamic Acid-Containing Proteins (Gla Proteins)
These proteins are named after the observation that the vitamin K-dependent, Ca2+-binding, γ-carboxyglutamic acid (Gla) amino acid is a constituent of their molecule. The Gla residues enhance calcium binding so that Gla proteins may function in the control of mineral deposition and remodeling [7]. They include osteocalcin, which is bone specific, and matrix Gla protein, which is also found in many connective tissues [8].
Osteocalcin (OSTC), a 6-kDa protein, is synthesized by the cells of the osteoblastic lineage; indeed, its expression has been considered as a marker of osteoblast differentiation in vitro.
OSTC is the second most abundant protein in the bone matrix, and it is highly conserved among all vertebrate species. The biological function of OSTC is probably related to the regulation of bone turnover and/or mineralization [9]. Osteocalcin-deficient mice are reported to have increased bone mineral density compared with normal mice[9], but with age, the mineral properties did not show the changes that occurred in age-matched controls, suggesting a role of OSTC in osteoclast recruitment and maturation [10, 11].
OSTC embedded in bone matrix is released during bone resorption as intact carboxylated molecules and fragments. In fact, part of OSTC, synthesized or derived from resorption activity, could be found in the blood. This circulating OSTC has been widely used in clinical investigations as a marker of bone formation, whereas protein expression as index of osteoblastic phenotype and bone formation in vitro [12]. Furthermore, serum OSTC levels are also considered as a marker of bone turnover rather than bone formation.
Circulating OSTC exists in two forms: carboxylated on 3 glutamate residues and undercarboxylated; recently, it is emerged that the latter form is able to enhance insulin secretion by β-cells, insulin sensitivity, and energy expenditure [13], suggesting that bone cells regulate energy metabolism through an endocrine mechanism and leading to the identification of OSTC, as a core of the cross-talk between bone remodeling and glucose metabolism [14].
Matrix Gla protein (MGP) is a small ubiquitous matrix protein containing carboxyglutamic acid, initially isolated from bone [15] but also found in all soft tissues. MGP shares many features with OSTC, but MGP and OSTC do not cross-react and they must be kept distinct [16]. It has been thought that MGP affects differentiation in developing cartilage and bone. In fact, some authors found that MGP overexpression in developing limb leads delayed chondrocyte maturation and blocked endochondral ossification [17]. Its mechanism as inhibitor of calcification is not fully understood, but studies in MPG-deficient mice demonstrated that they develop vascular calcifications and die [18].
2.1.3 Glycoproteins
Many glycosylated proteins with diverse functions are present in bone, and the most well-known among these proteins are osteonectin and alkaline phosphatase which will be examined below.
Osteonectin (OSN), the most abundant non-collagenous glycoprotein in bone, can bind to both collagen fibrils and hydroxyapatite. OSN concentration in bone matrix is variable and appears to be inversely correlated with the degree of calcification [19]. Although the role of OSN in bone needs to be better clarified, currently it has been recognized as a protein with a general multifactorial function with particular reference to the regulation of calcium-mediated processes, cell–matrix interactions, hydroxyapatite binding sites, and regulation of bone remodeling [20].
Alkaline phosphatase (ALP) is a member of a family of membrane-bound zinc metalloprotein enzymes that catalyze the splitting off a terminal phosphate group from an organic phosphate ester in an alkaline environment (pH 10) [21]. ALP is present not only on the cell membrane and in matrix vesicles, but also in the mineralizing matrices of cartilage and bone [22]. The isoform found in bone, the tissue non-specific alkaline phosphatase (TNAP), is surely decisive for the occurrence of a normal mineralization process as shown by the skeletal defects that develop in congenital hypophosphatasia and TNAP knockout mice. In both cases, in fact, bone is characterized by excessive amounts of osteoid tissue [23]. ALP promotes mineralization by decreasing the concentration of inorganic pyrophosphate, known inhibitor of mineralization, and increasing the concentration of the mineralization promoter, the inorganic phosphate (Pi). Recently, it has become recognized that pathologic calcification of the cardiovascular system follows an osteogenic mechanism [24, 25]. In all these models, expression of ALP is a key part of the process, and presumably acts similarly to its action in hard tissues, i.e., by decreasing the inhibitor of mineralization and increasing Pi levels, which promote mineralization process. In this regard, it has been shown that polyphenols, which are reputed to be cardioprotective, inhibit ALP activity in vascular smooth muscle cells, perhaps inhibiting artery calcification [26].
2.1.4 Small Integrin-Binding Ligand N-Glycosylated Protein (SIBLINGs)
Bone cells synthesize different proteins that may mediate cell attachment grouped as members of the small integrin-binding ligand, N-glycosylated protein (SIBLING) family. This family is constituted by osteopontin (OPN), bone sialoprotein (BSP), dentin matrix protein 1 (DMP1), and matrix extracellular phosphoprotein (MEPE). SIBLINGs are defined as small, soluble, and secreted proteins that can be localized through interactions with receptors either on the cell’s own surface, enabling autocrine activities, or nearby in paracrine way. They display in their sequence a proline-rich stretch (basic), consensus sites for casein kinase, an arginine–glycine–aspartic acid (RGD) sequence, and (apart for BSP) one or several acidic serine–aspartate-rich MEPE-associated peptides (ASARM), which have a high affinity for hydroxyapatite and appear to be potent regulators of mineralization. RGD motif is the cell attachment consensus sequence that binds to the integrin class of cell surface molecules even if in some cases, cell attachment seems to be RGD independent. The SIBLINGs can engage a number of cell surface integrins. Although SIBLINGs were thought to be functionally restricted to mineralized tissues, recent results show that they are more widely distributed and expressed in non-mineralized normal tissues, upregulated in a number of tumors associated with pathological microcalcifications, and able to metastasize bone. Future investigations should validate the use of SIBLINGs as prominent molecular tools for diagnostic, prognostic, and therapeutic applications in cancer.
Osteopontin (OPN) is expressed in bone and bone marrow by mature osteoblasts and osteoclasts and their precursors, as well as by osteocytes. OPN expression is modulated during the various stages of differentiation. Relatively undifferentiated early-stage osteoblasts migrate to the resorbed bone surface and secrete matrix components such as OPN which is recognized and bound by integrin on other activated osteoblasts (autocrine); here, OPN is immobilized onto preexisting mineral and/or organic moieties of the underlying bone and may act to initiate a new cycle of mineralization at this site. In this way, OPN has the function to mediate attachment of cells to mineral crystal structures; it has been demonstrated that OPN prevents both nucleation of mineral crystal formation and the growth of preexisting crystals [27, 28]. This inhibitory activity requires phosphorylated form of the protein. The production of the highly phosphorylated OPN is increased with osteoblast maturation. In addition to this, OPN may influence osteoclast activity during resorption [29]. The osteoclasts recognize OPN, adhere to the bone surface, and begin to resorb bone matrix. In many situations, OPN appears enriched in biological fluids, including blood, milk, urine, and seminal fluid having elevated levels of calcium; this is also indicative of a role in preventing spontaneous precipitation of calcium salts. Besides regulating bone resorption and bone calcification, OPN is active in diverse biological processes, such as wound healing, immunological reactions, tumorigenesis, atherosclerosis, and angiogenesis. In fact, some authors found that OPN acts as a potent constraining factor on hemopoietic stem cell proliferation, and its overexpression is a feature of hemopoietic malignancies, such as multiple myeloma and chronic myeloid leukemia, although its exact role in the aetiology and progression of these diseases remains unclear. Through osteoblasts and their cell surface, and expressed proteins, including OPN, bone is able to regulate the tissue that resides within it. In doing so, OPN can be considered a bridge between bone and blood [30].
Bone sialoprotein (BSP), which is a major non-collagenous extracellular matrix protein in bone, is produced by osteoclasts, osteoblasts, osteocytes, and hypertrophic chondrocytes and has long been identified as an early marker of osteogenic differentiation [31].
The Arg-Gly-Asp (RGD) sequence close to the carboxy-terminus of BSP protein is a cell attachment site recognized by αvβ3 integrin receptor, while polyglutamic acid regions in the amino-terminus mediate the binding of BSP to hydroxyapatite [32]. Although BSP expression has been shown to be coincident with de novo bone formation, substantial evidences suggested that BSP plays a role also in bone resorption. Some studies showed that BSP significantly stimulates bone resorption in a dose-dependent manner and this stimulation could be partially due to an increase in osteoclast adhesion to bone via its RGD sequence or its acidic sequences [33]. It is also conceivable that it is particularly involved in the process of osteoclastogenesis [34]. It has been suggested that BSP may contribute to the receptor activator of nuclear factor kappa-B ligand (RANKL)-mediated bone resorption by inducing osteoclastogenesis and osteoclast survival and decreasing osteoclast apoptosis. BSP levels are increased in patients affected by bone diseases with high bone remodeling, such as osteoporosis, hyperparathyroidism, Paget’s disease, and rheumatoid arthritis, when compared with controls [35]. Furthermore, serum BSP concentrations are significantly higher in postmenopausal women than in premenopausal ones [36]. Numerous studies have suggested that BSP is also involved in the process of bone metastasis though the underlying mechanisms are not perfectly clear yet. There is a significant correlation between high BSP serum values and both the presence and the risk for the subsequent detection of bone metastases. Therefore, serum BSP could be considered as an early marker and a prognostic factor for the development of bone metastases.
Dental Matrix Protein 1 (DMP1) is a bone- and teeth-specific protein initially identified from mineralized dentin. Two proteolytic fragments, 37 kDa N-terminal and 57 kDa C-terminal, have been purified from rat long bone and dentin extracts, suggesting that these may be the bioactive forms in vivo. Additionally, the presence of a third chondroitin sulfate-linked N-terminal fragment (DMP1-PG) [37] has been demonstrated and recently correlated with an inhibition function in mineralization [38]. However, what is currently known is that both the highly phosphorylated 57-kDa and 37-kDa fragments could act as hydroxyapatite nucleators [39]. In addition, the 57-kDa fragment contains almost all functional sequences and domains identified: the RGD motif, the nuclear localization signal, the ASARM peptide, and the peptide functioning as nucleator. DMP1 is a unique molecule that orchestrates mineralization of bone matrix and stimulates osteoblast differentiation during the later stages of their maturation [40]. DMP1 is primarily localized in the nuclear compartment of undifferentiated osteoblasts where it acts as a transcriptional factor for activation of osteoblast-specific genes, such as the osteocalcin gene. During the early phase of osteoblast maturation, Ca2+ surges into the nucleus from the cytoplasm, triggering the phosphorylation of DMP1 by a nuclear isoform of casein kinase II. This phosphorylated DMP1 is then exported into the extracellular matrix, where it regulates nucleation of hydroxyapatite. The importance of DMP1 in bone comes from studies in DMP1-null mice, in which it has been shown that vertebrae and long bones are shorter and wider with delayed and malformed secondary ossification centers and an irregular and highly expanded growth plate. Additionally, these mice unexpectedly develop a severe defect in cartilage formation during postnatal chondrogenesis and tooth mineralization defects [41, 42].
Matrix extracellular phosphoprotein (MEPE) is a protein predominantly expressed by osteocytes within mineralized bone. This protein is also expressed by differentiated osteoblasts, and its expression is markedly increased during matrix mineralization. Synthetic peptide fragment of MEPE, containing the RGD sequence and SGDG (Ser-Gly-Asp-Gly) glycosaminoglycan-attachment sequence, stimulates new bone formation in vitro and in vivo. MEPE is present in serum, and its levels correlate with bone mineral density and decrease in aged individuals when bone mineral density is low, suggesting a physiological role of MEPE in bone homeostasis [43]. MEPE protein is most likely cleaved by a cathepsin B-like protease to a highly phosphorylated C-terminal acidic serine and ASARM peptide in osteocytes. In vitro, it has been demonstrated that phosphorylated latter peptide inhibits osteoblast mineralization by binding to crystals in bone matrix, and the phosphorylation is necessary for the peptide affinity for crystals. ASARM peptides increase the expression of OPG, in bone marrow stromal cells [44]. MEPE might therefore be involved in the regulation of both osteoblast and osteoclast activities during bone remodeling. Furthermore, specific binding of MEPE to PHEX (phosphate-regulating gene with homologies to endopeptidases on the X chromosome) regulates the degradation of MEPE and the release of ASARM peptides which play a role in phosphate regulation and inhibit mineralization [45] by binding to hydroxyapatite [46]. Because of these properties, MEPE has been considered a bone renal hormone [47].
2.1.5 Other Adhesive RGD-Containing Glycoproteins
Fibronectin (FN) is one of the most abundant glycoproteins produced during early stages of bone formation by osteoblasts and accumulated extracellularly at sites of osteogenesis. The molecule is characterized by specific binding sites for heparin and collagen, and FN-cell recognition occurs via integrin receptor.
In vitro studies have shown a key role for FN in the assembly of collagen [48
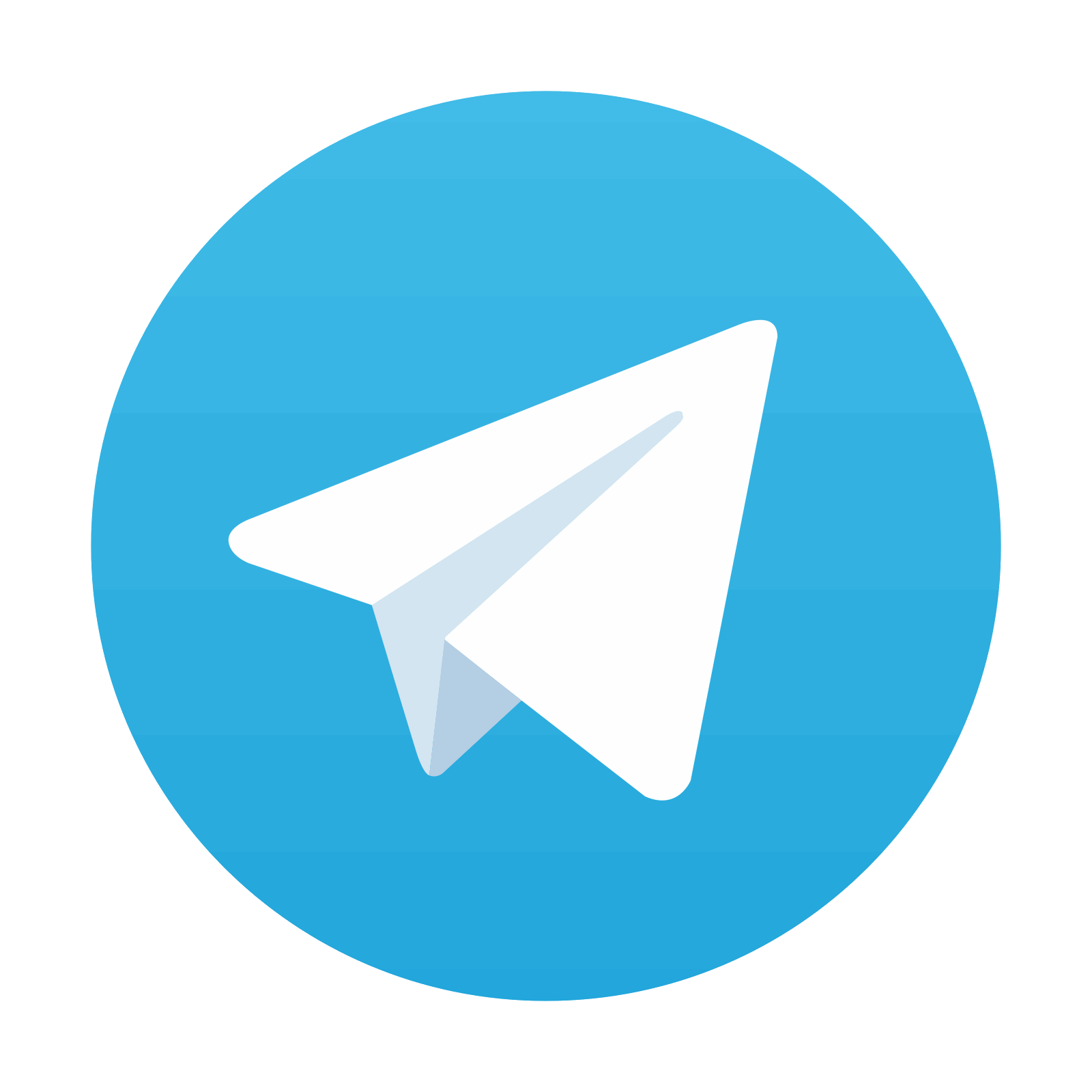
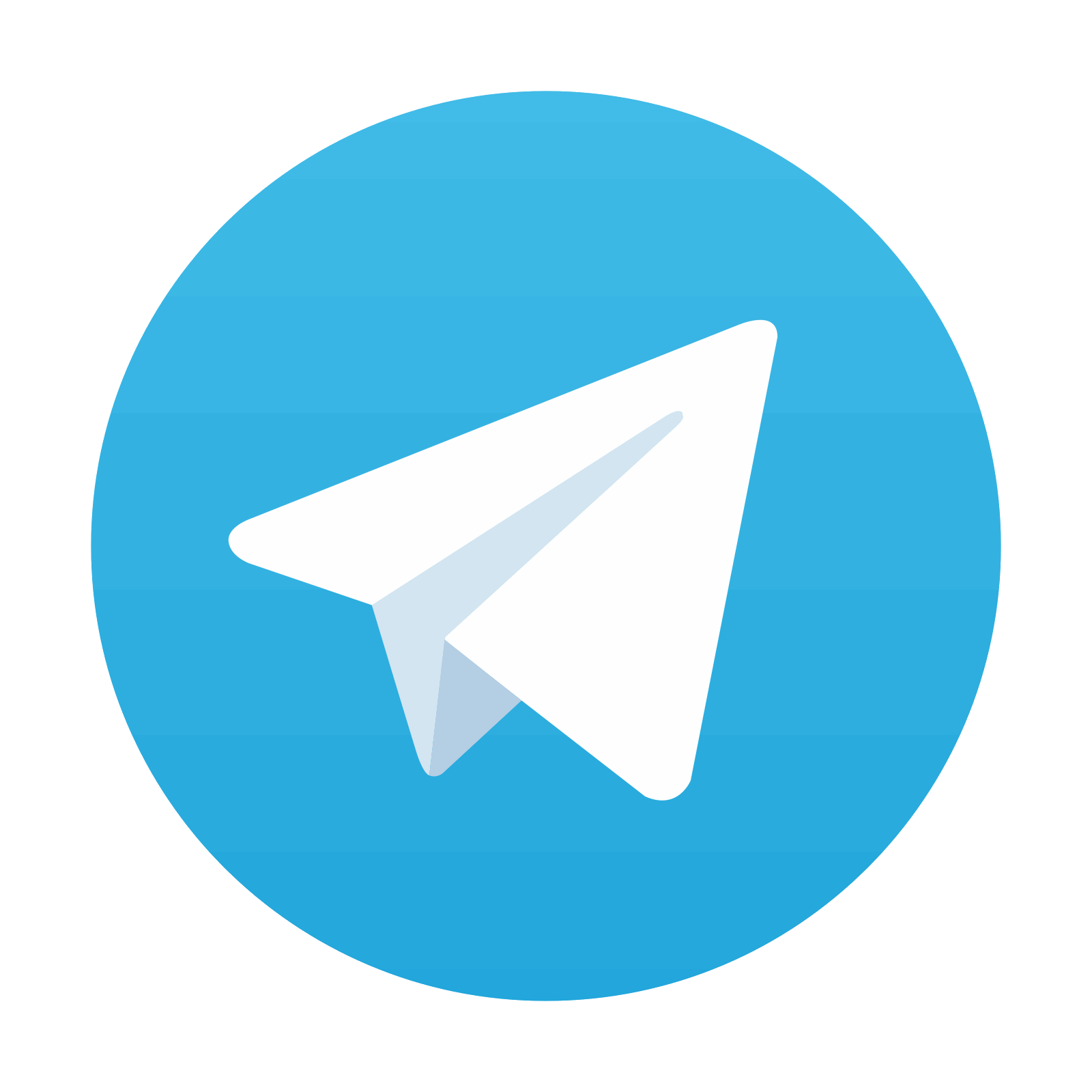
Stay updated, free articles. Join our Telegram channel

Full access? Get Clinical Tree
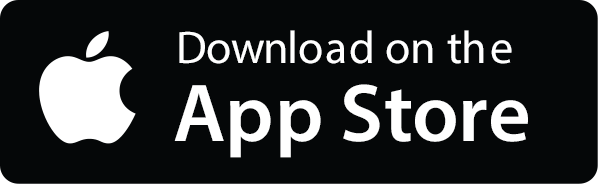
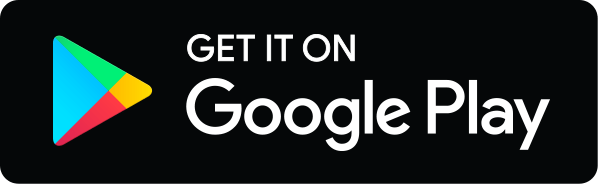