Brain arteriovenous malformations (AVMs) are characterized by shunting between pial arteries and cortical or deep veins, with the presence of an intervening nidus of tortuous blood vessels. These lesions present a therapeutic challenge, because their natural history entails a risk of intracranial hemorrhage, but treatment may cause significant morbidity. In this article, imaging features of AVMs on MR imaging and catheter angiography are reviewed to stratify the risk of hemorrhage and guide appropriate management. The angioarchitecture of AVMs may evolve over time, spontaneously or in response to treatment, necessitating ongoing imaging surveillance.
Key points
- •
Brain arteriovenous malformations (AVMs) are rare vascular lesions characterized by arteriovenous shunting between pial arteries and cortical or deep veins, with the presence of an intervening nidus of tortuous blood vessels.
- •
MR imaging and catheter angiography can be used to evaluate the angioarchitectural features of AVMs, stratifying the risk of hemorrhage and guiding the choice of appropriate management.
- •
According to the literature, untreated AVMs carry a 2% to 4% annual risk of hemorrhage; however, in a single prospective randomized trial, treatment of unruptured AVMs was associated with an even higher rate of periprocedural complications.
- •
Management strategies for AVMs include conservative surveillance, radiosurgery, open surgical resection, endovascular embolization, or a combination of the aforementioned. The management pathway is determined best by multidisciplinary discussion after comprehensive imaging characterization.
- •
Long-term follow-up imaging from AVMs generally is appropriate, but the schedule depends on the type of management and patient characteristics.
Introduction
Brain arteriovenous malformations (AVMs) are a type of intracranial vascular abnormality characterized by an abnormal tangle of blood vessels that results in arteriovenous shunting of pial arteries into cortical or deep venous channels. This definition of an AVM is purposefully inclusive, recognizing the variety in angioarchitecture that these lesions can exhibit. Input to the AVM is from 1 or more pial (and very rarely secondarily induced dural) arterial pedicles, feeding a nidus of abnormal blood vessels that contains the site or sites of shunting, with early drainage into veins that also may drain surrounding normal brain. Clinically, AVMs may exhibit a spectrum from incidentally found asymptomatic lesions to life-threatening causes of intracranial hemorrhage.
The purpose of imaging an AVM is first to identify features that indicate an elevated risk of hemorrhage or other morbidity, such as perinidal hypoxemia, and second to inform the choice of treatment or observation that provides the best balance of risk and benefit to the individual patient. Longitudinal imaging surveillance, either with conservative management or after treatment, enables monitoring for resolution of the AVM or the development of adverse findings that may necessitate a change in management.
Epidemiology and Clinical Presentation
Brain AVMs are rare lesions, with an estimated detection rate of 1 to 1.3 per 100,000 person-years over several population-based studies. Of these cases, one-third to two-thirds present with intracranial hemorrhage, whereas the remainder are discovered in the work-up of other symptoms, such as seizures, or incidentally (unrelated to the reason for which imaging is ordered). Many of the studies estimating the incidence of AVMs were performed in the 1990s and early 2000s; the increasing use of computed tomography (CT) and MR imaging since that time likely has resulted in a greater number of incidentally discovered AVMs, but to the authors’ knowledge, this effect has not been quantified.
AVMs may present at any age and occur equally between the sexes. It classically is understood that AVMs are congenital, although there are case reports of AVMs arising de novo in adulthood, fueling the hypothesis that an individual may be born with the propensity to develop a brain AVM during postnatal angiogenesis. Most AVMs occur sporadically, although there are known associations with syndromes, such as hereditary hemorrhagic telangiectasia (HHT) and cerebrofacial arteriovenous metameric syndrome (CAMS).
A recent genomic study of sporadic AVMs from tissue obtained after surgical resection showed activating KRAS mutations in a majority of samples. Expression of mutant KRAS in endothelial cells induced increased extracellular signal-regulated kinase (ERK) activity, expression of genes related to angiogenesis, Notch signaling, and migratory behavior; inhibition of mitogen-activated protein kinase (MAPK)-ERK signaling reversed these effects. These results suggest that sporadic AVMs may arise from somatic mutations that lead to KRAS-induced activation of the MAPK-ERK signaling pathways in endothelial cells.
Classification
The Spetzler-Martin grading system for AVMs, introduced more than 30 years ago, classifies AVMs on a 5-point scale based on the size of the nidus, location in eloquent or noneloquent regions of the brain, and the pattern of venous drainage. It correlates with the risk of neurologic deficit after surgical resection of the AVM. The Spetzler-Ponce model simplified the classification into 3 tiers, with recommendation for surgical resection for class A lesions (Spetzler-Martin grades 1 and 2), multimodality treatment of class B lesions (Spetzler-Martin grade 3), and observation for class C lesions (Spetzler-Martin grades 4 and 5). These classification schemes are useful for surgical risk estimation but help little in understanding the natural history of brain AVMs or the likelihood of adverse events following other treatment modalities.
Therefore, classification schemes also have been devised that are suited to other treatment modalities, including endovascular embolization and radiosurgery. Radiosurgical models like the Pittsburgh modified radiosurgery-based AVM score (RBAS) and Virginia Radiosurgery AVM Scale evaluate the overall rate of favorable outcomes (lesion obliteration and post-treatment complications); they generally have a more finely graded dependence of AVM nidus volume but also incorporate the location of the AVM, as well as other clinical factors, such as history of hemorrhage or age. Endovascular scores, such as the Toronto, Puerto Rico, Buffalo, and embocure scores, focus more on the angioarchitecture of the AVM, including factors, such as the number and configuration of the arterial pedicles and draining veins.
Imaging in the diagnosis of arteriovenous malformations
Imaging in the evaluation of a suspected AVM has several goals:
- 1.
To make the correct diagnosis, distinguishing brain AVMs from other intracranial vascular malformations, and identify any complications at the time of presentation that may require immediate management
- 2.
To stratify the risk of future complications, especially hemorrhage, perilesional hypoxemia, or venous congestion, and thereby establish the appropriateness of conservative management or treatment
- 3.
If AVM treatment is indicated, to choose the most effective modality for treatment with the lowest risk of adverse events
- 4.
To follow the AVM over time to monitor response to treatment or ensure stability with conservative management
Imaging Modalities and Protocols
The diagnostic imaging pathway leading to the discovery of an AVM depends on a patient’s clinical presentation. The initial diagnosis generally is made on CT or MR imaging, performed either emergently for the patient presenting with seizure or intracranial hemorrhage or electively for the patient presenting with more indolent symptoms like chronic headache.
Noncontrast CT inherently is limited in detecting AVMSs or evaluating their architectural features, but it is an excellent first line test to exclude acute hemorrhage. The pattern of AVM-related hemorrhage can vary, from purely intraparenchymal to mixed compartment to purely subarachnoid ( Fig. 1 ). Even in the absence of hemorrhage, an AVM may be detectable on noncontrast CT by serpentine or rounded mildly hyperattenuating structures, representing nidal or perinidal blood vessels, which should prompt recommendation for vascular imaging. Noncontrast CT also can assess for some other AVM complications, such as hydrocephalus from mass effect related to the nidus. Calcification of the nidus or perinidal brain tissue occasionally is observed. Rarely, spontaneous thrombosis of draining veins or perinidal edema may be observed.
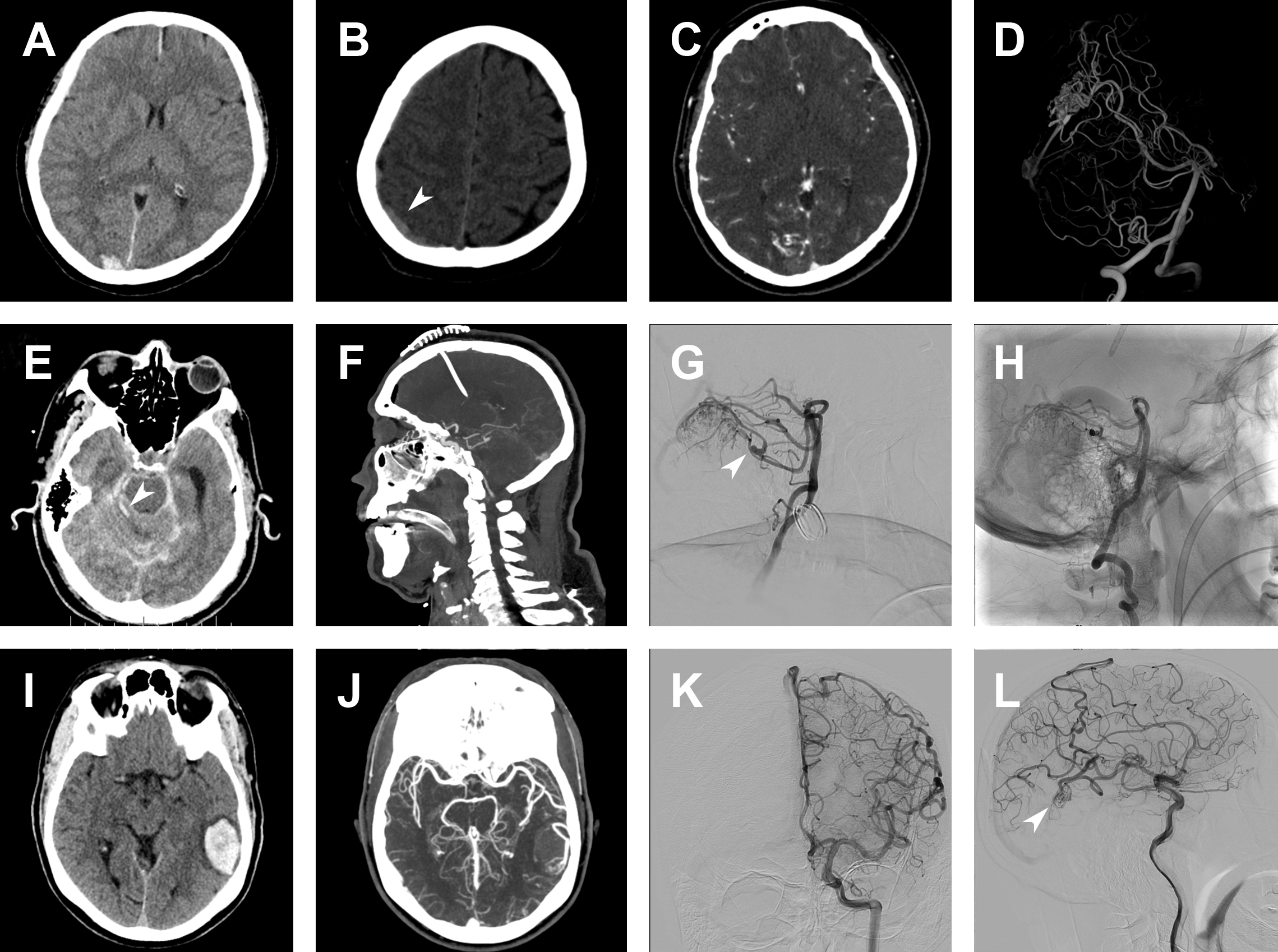
CT angiography (CTA) benefits from widespread availability and can be performed immediately on detection of intracranial hemorrhage, to assess for a cause and prompt referral to a neurovascular center. The AVM nidus typically is observed as a tangle of dysplastic blood vessels, which may be displaced or effaced by a hematoma cavity if present. Perinidal or intranidal aneurysms and stenoses are well visualized on CTA due to its high spatial resolution. In ruptured AVMs, the nidus should be scrutinized for an intranidal aneurysm pointing into the hemorrhagic cavity because this helps identify the rupture point. Arterial supply and venous drainage of the nidus generally can be inferred by tracing vessels approaching and departing the nidus, but the limited temporal information provided by conventional CTA reduces the ability to resolve the extent of fistulization within the AVM and identify minor feeders and drainage that may be prognostically important. Whole-brain 4-dimensional CTA and CT perfusion have been explored as methods to evaluate AVM hemodynamics, including cerebral circulation times, although at present these techniques are not in widespread use.
MR imaging is the preferred modality to assess for the effects of the AVM on the surrounding brain parenchyma. The authors’ routine institutional MR imaging protocol for AVMs includes axial and coronal T2 (for the size and location of the nidus), axial fluid-attenuated inversion recovery (FLAIR) (for perinidal edema or gliosis), axial susceptibility-weighted imaging (SWI) or gradient-recalled echo (GRE) (for evidence of prior hemorrhage), axial diffusion-weighted imaging (DWI) (for acute ischemia), and sagittal T1 sequences (for overall anatomy and detection of acute or subacute blood products). Vessel wall imaging has been used to help localize the site of bleeding from an AVM, which can help guide urgent treatment to prevent rebleeding. More recently, the authors have employed vessel wall imaging also in unruptured brain AVMs, especially those presenting with perilesional edema and found significant wall enhancement in partially thrombosed venous ectasias associated with edema, which may point toward an underlying thrombosis-related inflammation as a potential sign for impending rupture.
MR angiography provides similar information to CTA and is a suitable initial modality to identify the presence of an AVM for further investigation. Dynamic MR angiography, such as TRICKS (GE, Milwaukee, USA) or TWIST (Siemens, Erlangen, Germany), provides additional time-resolved information not available in single-phase MR angiography and can be used to identify arteriovenous shunting in an AVM but suffers from relatively low spatial and temporal resolution (although this is improving with newer MR angiography protocols ). MR angiography is performed routinely for imaging follow-up after AVM resection. Especially in children, its lack of radiation exposure and noninvasiveness are significant advantages, but the combination of TOF and contrast-enhanced MR angiography yielded only 75% sensitivity and 91% specificity in detecting recurrence in a pediatric population of treated AVMs. Conventional angiography, therefore, generally still is necessary to definitively diagnose AVM recurrence.
Digital subtraction angiography (DSA) remains the gold standard for diagnosis, evaluation, and surveillance of brain AVMs because it offers spatial and temporal resolution not yet matched by noninvasive techniques. The arteriovenous (AV) shunting characterizing an AVM is best demonstrated on DSA, and the intracranial arteries can be selectively interrogated to map the angioarchitecture in detail. Initial evaluation of an AVM generally requires a 6-vessel DSA, because perinidal angiogenesis can lead to recruitment of secondary vascular supply from other intra-axial and meningeal arterial territories. In addition, in certain syndromic forms of brain AVMs (Wyburn-Mason syndrome or cerebrofacial metameric syndromes) that may present with the concurrence of brain AVMs and facial AVMs, a 6-vessel angiography also is used to exclude AVM multiplicity, which is a hallmark finding in HHT. Follow-up angiograms then can be more focused on the vascular territories with known involvement. Three-dimensional and multiplanar reconstructions from rotational angiograms can help identify perinidal aneurysms and stenoses that sometimes may be difficult to resolve due to superposition on planar angiograms ( Fig. 2 ).
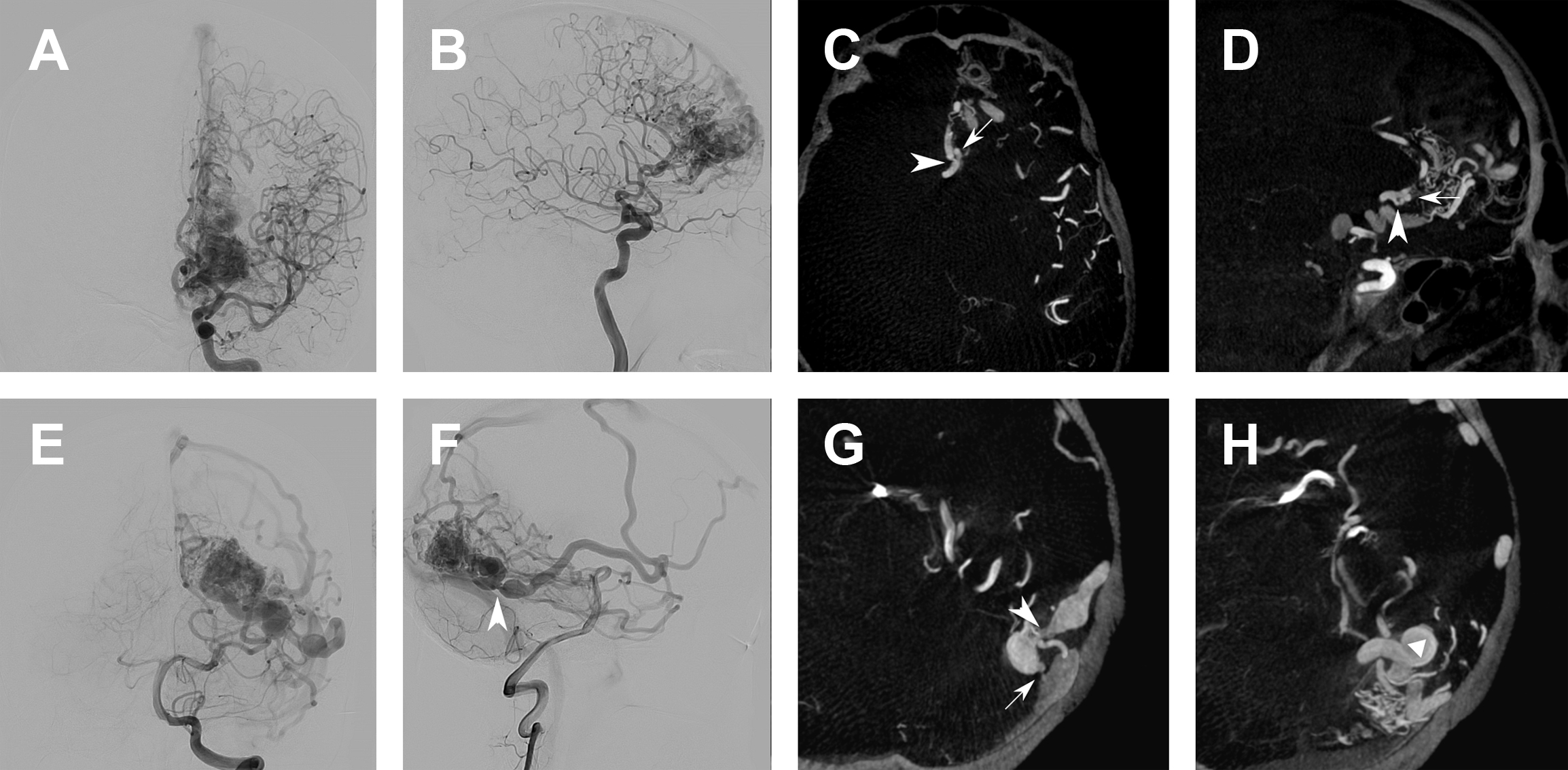
Other imaging modalities occasionally may be useful in the evaluation of AVMs. 18-Fluorodeoxyglucose (FDG)-PET can be used to show perinidal hypometabolism related to vascular steal from the AVM. MR diffusion tensor imaging can map white matter tracts near AVMs for preservation during surgical resection. A clinical trial of functional MR imaging to identify nearby eloquent brain tissue in advance of AVM resection showed no significant clinical benefit. Perinidal brain tissue shows abnormalities on CT perfusion, following 3 distinct patterns : decreased cerebral blood flow (CBF) and cerebral blood volume (CBV) with decreased mean transit time (MTT) (pattern 1), decreased CBF and CBV with increased MTT (pattern 2), and increased CBV and MTT (pattern 3). Pattern 1, a functional arterial steal phenomenon, was associated with seizures, and pattern 2, an ischemic arterial steal phenomenon, was associated with focal neurologic deficits. Pattern 3 was observed in AVMs exhibiting venous congestion.
Differential Diagnosis
It is important to distinguish true brain AVMs from other intracranial vascular lesions, which may appear similar especially on cross-sectional imaging, but have a different natural history and treatment strategy.
- 1.
Pure arterial malformations are rare abnormalities characterized by dilated, coiled or looped intracranial arteries, sometimes arranged as a mass mimicking an AVM nidus but without a venous component. They also may develop aneurysmal outpouchings surrounding the malformation. In a case series of 12 pure arterial malformation with an average follow-up of 29 months, there was no reported hemorrhage or infarction, suggesting a benign clinical course.
- 2.
Venous varices may occur in isolation from dilation of an intracranial vein without associated arterial pathology (in this sense they are the venous counterpart to the pure arterial malformation). They may occur both extra-axially or intra-axially, can be mistaken on MR imaging for a mass lesion, and occasionally may occur in associated with developmental venous anomalies. There is a small risk of hemorrhage from varices, but, if so, bleeding occurs at lower, venous pressures rather than at the higher, arterial pressures as with AVMs. They also may become symptomatic from mass effect or spontaneous thrombosis (in which case they may not be visible on cerebral angiography).
- 3.
Cerebral proliferative angiopathy (CPA) is a rare entity consisting of diffuse development of intra-axial ectatic blood vessels, often involving a large portion of a lobe or multiple lobes, but unlike an AVM it does not exhibit AV shunting. Patients with CPA often develop seizures, headaches, or neurologic deficits from associated ischemia, whereas hemorrhage occurs only occasionally.
- 4.
Dural AV fistulas share the arteriovenous shunting of brain AVMs and show dilated feeding arteries and draining veins. Unlike brain AVMs, arterial supply is from branches that normally supply the meninges, not the brain parenchyma itself, ie the external carotid artery (ECA) branches but also meningeal branches of the internal carotid artery (ICA) and vertebral arteries.
- 5.
Pial AV fistulas, in distinction to dural AVFs but like brain AVMs, involve branches of the ICA and vertebrobasilar system that normally supply the brain parenchyma. Unlike true AVMs, however, they lack a discrete parenchymal nidus. As discussed later, AVMs exist on a spectrum from nidal-type to fistulous-type, and in a sense a pial AVF sits at the extreme fistulous end of the brain AVM spectrum. They are encountered more commonly in childhood, and RASA1 mutations and HHT often are underlying genetic causes.
- 6.
Developmental venous anomalies, when large, may simulate the nidus of an AVM. They typically do not exhibit arteriovenous shunting like an AVM, but there is a rare subset of DVAs that do show early venous filling due to the dilated capillary bed. Rarely can they be associated with an AVM. They generally are asymptomatic, incidental findings that require no further treatment, although there is a small risk of spontaneous thrombosis with associated venous infarct in the surrounding brain, which drains obligately through the DVA.
- 7.
Cavernous malformations may to some degree resemble the nidus of an AVM on cross-sectional imaging, showing a compact cluster of fluid spaces on T2-weighted MR imaging, but these are distinct from the more serpentine flow voids of an AVM nidus. They are low-flow, nonshunting lesions that are occult on cerebral angiography and show conspicuous hemosiderin staining on SWI or GRE MR imaging sequences. Although cavernous malformations have a propensity for low-grade bleeding, it tends to be self-limited. They typically are managed conservatively unless there are frequent, symptomatic episodes of hemorrhage.
Important Features to Assess
To characterize an AVM, it is helpful to focus separately on the nidus itself, its arterial inflow, venous outflow, and secondary effects on surrounding brain. Each of these components has important features, as listed, that inform risk stratification and treatment selection:
- 1.
Nidus
- a.
Location determines the adjacent brain structures that the AVM places at risk, whether from complications of the AVM itself, such has hemorrhage or ischemia, or from treatment of the AVM. The Spetzler-Martin system considers the following locations to be eloquent, with a risk of disabling neurologic deficit if injured during resection: sensorimotor, language, and visual cortex; the hypothalamus and thalamus; the internal capsule; the brain stem; the cerebellar peduncles; and the deep cerebellar nuclei. AVM location naturally has an impact on surgical accessibility.
- b.
Size also is part of the Spetzler-Martin system, stratifying AVMs based on whether the largest diameter of the nidus measures less than 3 cm, 3 cm to 6 cm, or greater than 6 cm. A 2-fold increase in diameter, however, implies an 8-fold increase in volume, so these categories are broad and lack sufficient precision for radiosurgery evaluation, where nidus volume is a major determinant of feasibility. For radiosurgery treatment, planning detailed contouring of the nidus is needed, although for initial evaluation, measurement of the nidus along 3 orthogonal axes is sufficient.
- c.
Compactness refers to whether or not there is neural tissue interposed between the vessels in the nidus: a compact nidus contains only vessels and surrounding fluid, whereas a diffuse nidus has vessels interspersed with normal or gliotic brain ( Fig. 3 ).
Fig. 3
Diffuse versus compact AVM nidus. Anteroposterior ( A ) and lateral ( B ) angiograms pre–Gamma Knife of a 20-year-old man with a right thalamic AVM ( A , B , arrowheads ) that bled the previous year. Enhancement of the nidus is diffuse and the boundary ill-defined. The postcontrast fast spoiled gradient-echo ( C , arrowhead ) MR imaging through the nidus ( C ) shows the AVM vessels interspersed with brain parenchyma in the right thalamus. Axial T2 MR imaging ( D ) through the nidus shows the hemosiderin-stained cavity from the remote ( D , arrowhead ) hematoma. Anteroposterior ( E ) and lateral ( F ) angiograms pre–Gamma Knife of a 32-year-old man presenting with seizure with a left parasagittal parietal AVM with a compact nidus, showing a well circumscribed boundary ( E , F , arrowhead ). Postcontrast ( G ) and T2 ( H ) MR imaging similarly show the compact nidus with AVM vessels at the pial surface without intervening brain parenchyma ( G , H , arrowheads ).
- d.
Shunting is a feature of all AVMs, but the degree of shunting (as evidenced by early venous enhancement on time-resolved studies like DSA) can vary considerably. Nidal-type AVMs show less shunting compared with fistulous-type AVMs that may exhibit a large degree of shunting and associated high-flow angiopathy. In extreme cases of shunting contrast recirculation through the heart can be seen before contrast has fully transited the normal brain parenchyma.
- e.
Perinidal angiogenesis occurs in response to chronic hypoxemia around the AVM induced by the shunting of blood through the nidus without perfusing capillaries necessary to release oxygen to surrounding brain tissue. Arteries from adjacent vascular territories enlarge and proliferate; artery-to-artery anastomoses may form with the nidus itself. Dural arteries from ECA branches also may be recruited ( Fig. 4 ) secondary to the AVM-induced hypoxemia.
Fig. 4
A previously healthy 18-year-old man presented with seizures. Anteroposterior ( A ) and lateral ( B ) angiograms of the left ICA show a large right paramedian parietal AVM nidus supplied by dilated pericallosal and callosomarginal arteries. Venous drainage is into the right basal vein of Rosenthal. Anteroposterior ( C ) and lateral ( D ) angiograms of the right vertebral artery show an additional contribution to the nidus from dilated right posterior cerebral artery. Note the small perinidal aneurysms ( C , D , arrows ) on the P1 segment of the right posterior cerebral artery. This component of the nidus has superficial venous drainage into a dilated venous pouch ( C , D , arrowheads ) and then into cortical veins. Anteroposterior ( E ) and lateral ( F ) angiograms of the right ICA show extensive perinidal angiogenesis from right middle cerebral artery collaterals. Anteroposterior ( G ) and lateral ( H ) angiograms of the right ECA show induced dural supply from branches of the right middle meningeal artery. Axial ( I ) and coronal ( J ) T2 MR imaging images show the flow voids of the AVM nidus, the dilated venous pouch ( I , J , arrowheads ), and the prominent leptomeningeal collaterals ( I , J , arrows ). Axial FLAIR MR imaging sequence through the nidus ( K ) shows high signal in the perinidal brain parenchyma, suggesting chronic ischemia. The nidus shows expected enhancement on axial T1 postcontrast MR imaging ( L ). Microcatheter exploration of the nidus was performed ( M ) and ( O ) to identify fistulous points that could be embolized with glue injection ( N ) and ( P ) to reduce shunting. The patient then proceeded to radiosurgery for treatment of the remainder of the AVM.Stay updated, free articles. Join our Telegram channel
Full access? Get Clinical Tree
- a.
