5
Brain Metastases
Mohammad Hasan and May N. Tsao
INTRODUCTION
Demographics
Metastatic disease to the brain is the most common intracranial tumor.1 The incidence of brain metastases is at least 10% for patients diagnosed with lung cancer, renal cancer, colorectal cancer, breast cancer, and melanoma,2 with the highest incidence being 20% for lung cancer. With an aging population it is expected that the number of patients with metastatic disease will increase, including the number of patients with metastatic disease to the brain.
Prognosis
From a clinical standpoint, survival estimates for patients with brain metastases are important for patients and families to help guide health care decisions and may provide the impetus for patients to focus on work and personal (including social and financial) planning. From a research perspective, survival comparisons in trials for patients with brain metastases depend not only on the intervention but also on prognostic factors. Outcomes comparing interventions for patients with brain metastases are only valid when the trial arms are balanced with known and unknown prognostic factors.
The Radiation Therapy Oncology Group recursive partitioning analysis (RTOG RPA)3 examined statistically significant prognostic factors for survival for newly diagnosed patients treated in three RTOG whole brain radiation therapy (WBRT) trials, examining different dose fractionation schemes alone or with radiosensitizers. Published in 1997, the RTOG RPA consisted of three classes. Class I patients were patients with Karnofsky performance score (KPS) over 70, whose age were less than 65, and with a controlled primary and the brain as the only sites of visible cancer. RPA Class I patients had a median survival of 7.1 months. Class III RTOG RPA patients were those with a KPS less than 70. These patients had a median survival of 2.1 months. Class II RTOG RPA patients were brain metastases patients who do not meet the criteria for either Class I or Class III categories. Their median survival was 4.2 months.
However, the recognition that other prognostic factors (such as histology and number of brain metastases) were not captured in the RTOG RPA emerged. In 2010, the diagnosis specific graded prognostic assessment (DS-GPA) was published.4 Characteristics from a retrospective database of 5,067 patients with newly diagnosed brain metastases from 11 institutions treated between 1985 and 2007 were analyzed. Unlike the RTOG RPA, the DS-GPA depends on histology. A score is given for each significant prognostic factor. Survival estimates were obtained based on the sum of scores given a specific histology (Tables 5.1 and 5.2).
In 2012, a further refinement in prognosis for breast cancer metastases to the brain was made based on a multi-institutional retrospective database of 400 breast cancer patients5 treated for newly diagnosed brain metastases (Tables 5.3 and 5.4).
TABLE 5.1 Graded Prognostic Factor Scoring Table4
TABLE 5.2 Median Survival (in Months) Based on Overall GPA Score4
TABLE 5.3 Graded Prognostic Index for Breast Cancer Brain Metastases5
TABLE 5.4 Median Survival in Months for Breast Graded Prognostic Assessment5
Breast-GPA | Median Survival (Months) |
0–1 | 3.4 |
1.5–2 | 7.7 |
2.5–3 | 15.1 |
3.5–4 | 25.3 |
GPA, graded prognostic assessment.
MANAGEMENT
Steroids
Steroids provide temporary relief of symptoms due to intracerebral edema from brain metastases. The steroid of choice is dexamethasone and the starting dose of 4 to 8 mg per day has been suggested in clinical practice guidelines.6 However, if a patient experiences severe symptoms of increased intracranial pressure, higher doses such as 16 mg per day or more should be considered.
The only contemporary randomized trial that has examined the use of optimal supportive care versus optimal supportive care and WBRT was the QUARTZ trial.7,8 An interim analysis (reporting on 151 patients out of a planned 534 patients) indicated no detriment in quality of life, overall survival, or quality-adjusted life years (QALYs) between the two arms.7 In this interim analysis, 8% (12/151), 42% (64/151), and 50% (75/151) were classified as RTOG RPA classes I, II, and III, respectively. Overall survival between the two arms was similar for 49 days for optimal supportive care and WBRT versus 51 days for optimal supportive care alone (hazard ratio [HR] 1.11, 95% CI 0.80–1.53). The final trial results have been published in abstract form.7 For the 538 patients recruited, there was no significant difference in overall survival [HR 1.05 (95% CI 0.89–1.26)]. The median survival of optimal supportive care and WBRT versus optimal supportive care alone was 65 versus 57 days, respectively. Overall quality of life or steroid use was no different between the two arms.
An older trial9 randomized patients to prednisone with or without WBRT. The proportion of patients with improved performance status was similar between the two arms and median survival was 10 weeks in the steroid alone arm versus 14 weeks in the combined arm (P value not stated). The increase in median survival in the WBRT and prednisone arm is most likely attributed to the efficacy of radiation therapy in delaying the growth of brain metastases.
As such, there are subsets of brain metastases patients who have poor prognoses (such as patients with poor performance status and progressive extracranial disease despite systemic therapy) who are unlikely to benefit from radiotherapeutic intervention.
Single Brain Metastasis
The benefit of surgery for the management of selected patients with resectable single brain metastasis emerged from randomized trials published in the 1980s and 1990s (Table 5.5a–d). Randomized trials support the use of surgery to improve survival as compared to WBRT alone in selected patients with controlled systemic disease and a resectable single brain metastasis.10 The histologies excluded in the trials on the use of surgery were radiosensitive histologies such as small cell lung cancer, lymphoma, leukemia, and germ cell tumors. The use of postoperative WBRT for patients with resected brain metastases improves overall brain control but does not improve survival.11
TABLE 5.5 Randomized Trials for Single Brain Metastasis
(a) Surgery for Single Brain Metastasis (WBRT and Surgery vs. WBRT)
(b) Surgery Versus Surgery and WBRT for Single Brain Metastasis
(c) Radiosurgery for Single Brain Metastasis (WBRT and SRS vs. WBRT)
(d) Radiosurgery for Single Brain Metastasis (SRS vs. WBRT and SRS)
In a meta-analysis of three randomized trials on resection and WBRT versus WBRT alone for single brain metastasis,20 no significant difference in survival was noted, HR 0.74 (95% confidence interval [CI] 0.39–1.40, P = .35). However, there was a high degree of heterogeneity between trials. In particular, the Mintz et al. trial14 included a higher percentage of patients with active extracranial disease. One trial12 reported that surgery and WBRT increased the duration of functionally independent survival (FIS) HR 0.42 (95% CI 0.22–0.80, P <.008). There is a trend for surgery and WBRT to reduce the number of deaths due to neurological cause odds ratio (OR) 0.57 (95% CI 0.29–1.10, P = .09).
However, the use of WBRT has been shown to negatively affect neurocognition and quality of life.19,21 For small (<4 cm) single brain metastasis, particularly those located in eloquent areas not resectable, radiosurgery alone is currently being used to control the targeted metastasis and to avoid the side effects of WBRT. In addition, experimental strategies (e.g., surgery for single brain metastasis followed by postoperative cavity radiosurgery or focal postoperative cavity radiation) to avoid WBRT have emerged.
Four or Less Brain Metastases
The use of radiosurgery alone versus radiosurgery and WBRT has been studied in numerous randomized trials.16,18,19,21 These trials included good performance status patients with 1 to 4 brain metastases less than 4 cm in size and inactive extra-cranial disease. Overall there is improved whole brain control with the use of adjuvant WBRT added to radiosurgery. In all but one trial,19 overall survival is not different with the use of radiosurgery and WBRT as compared to radiosurgery alone. Two trials19,21 reported worsening in neurocognitive function at 3 to 4 months after adjuvant WBRT as compared to radiosurgery alone.
In 2014, the American Society for Radiation Oncology’s (ASTRO) “Choosing Wisely” campaign recommended against the routine use of adjuvant WBRT with stereotactic radiosurgery (SRS) for patients with a limited number of brain metastases. Randomized trials do not demonstrate a survival benefit in this setting. WBRT is associated with diminished cognitive function, worse quality of life, and more fatigue. Patients treated with SRS alone can be surveilled for the development of metastases in the brain. Adjuvant WBRT can be carefully considered at the time of recurrence to promote the best quality of life.1
Patients who are ineligible for radiosurgery, due to the size of the metastatic deposits, poor performance status, and/or active extra-cranial disease may be treated with WBRT and/or supportive care.
Five or More Brain Metastases
Controversy exists as to whether patients with 5 to 10 brain metastases (all <4 cm in size) should be treated with WBRT or SRS alone. Proponents for SRS alone advocate that in the absence of survival advantage as compared to WBRT, patients should undergo SRS alone as there may be neurocognitive and quality of life sparing with the avoidance of WBRT.22 On the other hand, justification for the use of WBRT includes improved overall brain control as compared to SRS alone. Patients treated with SRS alone are at much higher risk of distant brain relapse which also is associated with neurocognitive decline.23 In terms of expense and resources, SRS is more costly and resource intensive to administer compared to WBRT.
At present, there are no published phase III randomized controlled trials which directly compare SRS alone versus WBRT for patients with five or more brain metastases. Outcomes of interest include survival, treated brain metastases control, overall brain control, quality of life, and neurocognition.
EMERGING THERAPIES
Neurocognitive Protection
Experimental strategies such as hippocampal radiation sparing,24 and the use of medications such as memantine25 and donezepil,26 have been used with the intent to spare neurocognitive function with WBRT.
Hippocampal Sparing
Neural stem cells in the subgranular zone of the hippocampus are believed to play a role in replenishing depleted neurons. It is believed that WBRT adversely affects neurocognition by depleting neural stem cells in the subgranular zone of the hippocampus. The hippocampus is rarely the site of brain metastases and as a result efforts to spare the hippocampus and thereby possibly spare memory decline with WBRT were undertaken.24
In the RTOG-0933 phase II trial, the authors reported that the use of hippocampal sparing WBRT was associated with preservation of memory and quality of life as compared to historical series of patients.27
Pharmacological Intervention for Neurocognition
A randomized double-blind, placebo-controlled trial reported that memantine was well tolerated and had similar toxicity to placebo in patients treated with WBRT. Overall, patients treated with memantine had better delayed time to cognitive decline and reduced rate of decline in memory, executive function, and processing speed as compared to placebo.25
Another phase III randomized placebo-controlled trial examined the use of donepezil in patients treated with partial or WBRT. While donepezil did not improve overall composite cognitive scores, it did result in some improvement with respect to several cognitive functions, particularly among patients with greater baseline impairments.26
Molecular Agents
Over the past decade, targeted agents which inhibit specific molecular targets in cancer cells have been developed. The classification of targeted therapies for cancer includes tyrosine kinase inhibitors, monoclonal antibodies, and cancer vaccines.28 Brain metastases have shown responses to some targeted agents.
Lung cancer
A randomized phase III trial of WBRT and radiosurgery versus radiosurgery alone with either temozolomide or erlotinib (an epidermal growth factor receptor inhibitor) was reported for 126 out of 381 planned patients.29 This trial closed early due to poor accrual. The median survival times were worse for the WBRT and radiosurgery with temozolomide or erlotinib as compared to WBRT and radiosurgery alone (6.3 vs. 6.1 months vs. 13.4 months, respectively), although the differences did not reach statistical significance likely due to the small numbers. The addition of temozolomide or erlotinib was associated with more frequent grades 3 to 5 toxicity as compared to WBRT and radiosurgery alone (41%, 49%, vs. 11%, respectively). Lack of tumor EGFR testing was a major limitation of this trial.
A few small prospective and retrospective series report brain metastases response with the use of EGFR targeted agents alone, without brain radiation.30–32
Breast cancer
The LANDSCAPE prospective multi-institutional phase II trial33 enrolled 45 HER2 positive breast cancer patients with brain metastases (who did not receive prior WBRT) to lapatinib (a tyrosine kinase inhibitor) and capecitabine. Intracranial response (all partial) was seen in 65.9% of patients. However, 49% had grade 3 or 4 toxicity. No grade 5 toxicities were noted.
Other studies have also supported the finding of intracranial response to lapatinib alone.34 In a multicenter phase II trial of lapatinib for salvage of 242 previously treated brain metastases patients with HER2-positive breast cancer, 6% experienced 50% or more volumetric reduction in brain metastases, 21% showed 20% or more volume reduction in brain metastases.
Melanoma
In a multi-institutional phase II trial, dabrafenib (a BRAF inhibitor) was associated with 39.3% intracranial response in those patients who had no prior brain therapy and 30.8% brain response in those patients who had progressive metastases after previous brain therapy.35
A few studies have also examined the use of vemurafenib (a BRAF inhibitor) alone in the treatment of brain metastases from melanoma and intracranial response varies from 37% to 50%.36,37
Ipilimumab blocks cytotoxic T-lymphocyte-associated antigen 4 (CTLA-4) and has been used experimentally either alone or with radiosurgery in a small number of patients with metastatic melanoma to the brain.38,39
Molecular targeted agents have not been used routinely for the initial management of newly diagnosed brain metastases patients. The targeted therapy studies have focused largely on brain responses. Further research in this modality is needed to ascertain whether there is any survival, quality of life, or symptom control benefit with these agents as compared to WBRT and/or radiosurgery.
Radiation Treatment Planning
Whole brain radiation therapy
For WBRT, the CT simulation involves the patient lying supine with the head in a neutral position by a thermoplastic mask and neck rest. The beam arrangement is lateral opposing fields with collimation to shape the beam. Shielding is used to exclude the lens and extra-cranial contents from direct irradiation. The typical fractionations used are hypofractionated regimens such as 20 Gy in 5-daily fractions or 30 Gy in 10-daily fractions. No other WBRT dose fractionation schedule has been found to result in better survival.40
The WBRT fractionation schedules studied in phase III randomized trials include 10 Gy in 1 fraction, 12 Gy in 2-daily fractions, 32 Gy in 20 fractions twice daily with a 22.4 Gy in 14-daily fractions boost to visible brain metastases, 40 Gy in 20 fractions twice daily, and 50 Gy in 20-daily fractions. All these different fractionation schemes were compared to either standard 30 Gy in 10-daily fractions or standard 20 Gy in 5-daily fractions. Forty percent of patients treated with a single 10 Gy to the whole brain developed acute complications (headache, nausea, vomiting, neurologic deficit, or decline in level of consciousness) compared to 27% treated to 30 Gy in 10-daily fractions. Due to the lack of formal neurocognitive testing in these trials, it is unclear which WBRT regimen, if any, might be associated with less neurocognitive decline. There is also no evidence of better symptoms or quality of life outcomes with these different WBRT fractionation schemes as compared to 20 Gy in 5-daily fractions or 30 Gy in 10-daily fractions.
The WBRT dose fractionation is prescribed at the International Commission of Radiation Units and Measurements (ICRU) reference point for radiation plans using dosimetry. Without dosimetric plans, the WBRT dose can be prescribed to midplane. Figure 5.1 demonstrates a WBRT plan in the axial and sagittal planes for a dose of 20 Gy in 5 fractions. Figure 5.2 demonstrates the radiation isodose curves and digitally reconstructed radiographs (DRRs) demonstrating field definition for a patient treated with 30 Gy in 10 fractions. A scalp block has been added using a field-in-field technique to minimize the high-dose region in the scalp, which allows more complete hair regrowth.
FIGURE 5.1 Axial and sagittal isodose lines for a radiation plan delivering 20 Gy in 5 fractions.
FIGURE 5.2 DRRs for the opposed laterals (a, c) and the field-in-field (b, d) beams together with sagittal (e) and axial (f) image planes with the radiation isodose curves. Dose delivered 30 Gy in 10 fractions.
Stereotactic radiosurgery
The American Association of Neurological Surgeons (AANS), the Congress of Neurological Surgeons (CNS), and the ASTRO defined SRS in 2009 to include the following criteria:
Use of ionizing radiation to ablate target(s) in the head or spine without surgery
High-resolution stereotactic imaging for target localization
Multidisciplinary team participation (a neurosurgeon, radiation oncologist, and medical physicist)
Delivery of radiation in a single or limited number of sessions (up to 5) with stereotactic immobilization that may incorporate robotics or real time imaging
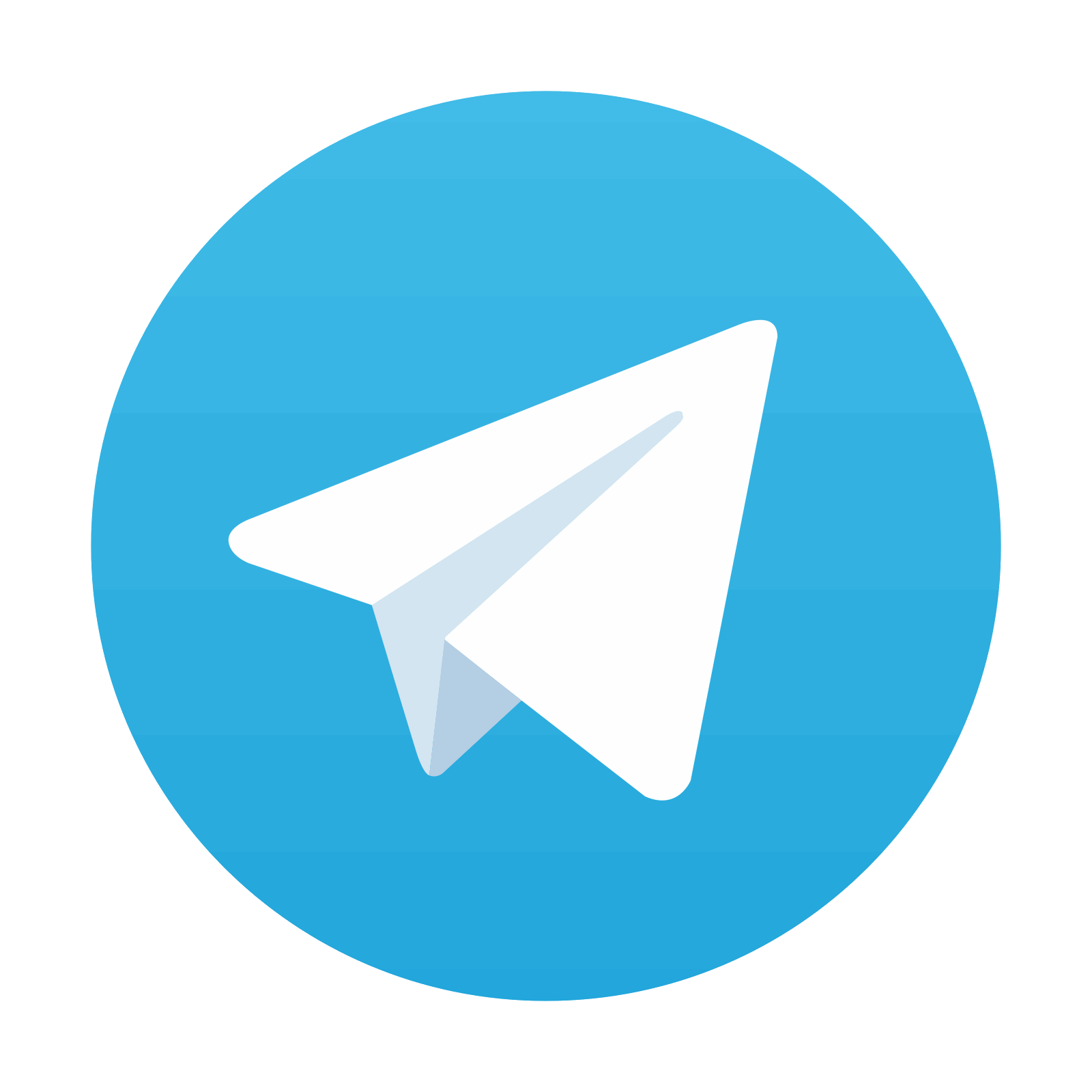
Stay updated, free articles. Join our Telegram channel

Full access? Get Clinical Tree
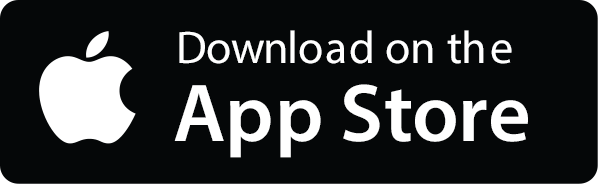
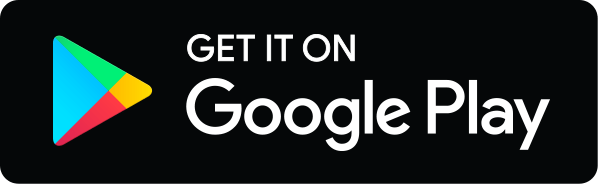