Stage
Description
Primary Tumor (T)
Tx
Primary tumor cannot be assessed
T1
≤3 cm tumor, surrounded by lung parenchyma
T1a
≤2 cm tumor
T1b
2.1–3 cm tumor
T2
>3–7 cm tumor, involvement of visceral pleura, invading mainstem bronchus >2 cm from carina, or causing atelectasis to a single lobe of the lung
T2a
3.1–5 cm tumor
T2b
5.1–7 cm tumor
T3
>7 cm tumor, tumor invading mainstem bronchus <2 cm from carina, invasion of diaphragm, chest wall, pericardium, mediastinal pleura, or associated atelectasis or obstructive pneumonitis of entire lung, or satellite nodule in the same lobe
T4
Invasion of great vessels or adjacent organs, or nodules in separate lobe in the ipsilateral lung
Regional Lymph Nodes (N)
Nx
Regional lymph nodes cannot be assessed
N0
No regional lymph nodes metastasis
N1
Ipsilateral hilar or peribronchial nodes
N2
Ipsilateral mediastinal or subcarinal nodes
N3
Any supraclavicular/scalene node or contralateral mediastinal/hilar nodes
Distant Metastasis (M)
M1a
Malignant pleural effusion, pericardial nodules/effusions, or lung nodules in contralateral lung
M1b
Metastasis to distant organs
Table 2
Stage grouping International Association for the Study of Lung Cancer 2007
Stage grouping | T | N | M |
---|---|---|---|
Occult carcinoma | Tx | N0 | M0 |
Stage 0 | Tis | N0 | M0 |
Stage IA | T1a, b | N0 | M0 |
Stage IB | T2a | N0 | M0 |
Stage IIA | T1a, b | N1 | M0 |
T2a | N1 | M0 | |
T2b | N0 | M0 | |
Stage IIB | T2b | N1 | M0 |
T3 | N0 | M0 | |
Stage IIIA | T1, T2 | N2 | M0 |
T3 | N1, N2 | M0 | |
T4 | N0, N1 | M0 | |
Stage IIIB | T4 | N2 | M0 |
Any T | N3 | M0 | |
Stage IV | Any T | Any N | M1a, b |
Some authors (Slotman and Karim 1994; Gauden et al. 1995; Hayakawa et al. 1996; Cheung et al. 2000; Yamada et al. 2003; Chen et al. 2006; Fang et al. 2006; Zhao et al. 2007) found no influence of Karnofsky Performance Status (KPS) and/or weight loss on either OS or DFS/CSS or even LRFS (Chen et al. 2006; Fang et al. 2006), while others (Hayakawa et al. 1992; Rosenthal et al. 1992; Jeremic et al. 1999a, b; Jeremic et al. 2006; Zhao et al. 2007; Firat et al. 2002a, b; Lagerwaard et al. 2002; Kupelian et al. 1996; Jeremic et al. 2005) noted its effect on either OS or DFS/RFS as well as on DMFS (Jeremic et al. 2005, 2006). Clear explanation for the lack of stronger evidence of influence of both PS and weight loss is missing, possibly because there is frequent interdependence of the two. The magnitude of the effect of these two factors is usually a consequence of the existing disease, i.e. tumour burden. In early stage NSCLC, with the least tumour burden, it is likely that the effect of these two factors should be less pronounced than in locally advanced NSCLC. In current studies, however, they appear to be the strongest predictors of outcome, an important finding for future research.
We have previously (Jeremic et al. 2005, 2006) investigated influence of tumor location and observed improved OS for patients having peripheral tumours. Similarly, Chen et al. (2006) found improved OS for peripheral tumours, but no impact on CSS or LRFS. In patients treated with hyperfractionated (Hfx) RT alone (Jeremic et al. 2006), peripheral tumour location also independently predicted improved LRFS, but not DMFS. It remains unknown what may be the cause of this discrepancy, although perhaps central tumour location may lead to more regional and/or distant seeding. As an indirect evidence, Emami et al. (2003) suggested that irradiation of hilar regions may contribute to some improvement in survival in these patients. However, other studies found no such difference (Ono et al. 1991; Hayakawa et al. 1992; Slotman and Karim 1994; Slotman et al. 1996; Cheung et al. 2000; Yamada et al. 2003). Based on recent data, we are unable to confirm an independent influence of tumour location on treatment outcome. A possible explanation may lie in a presumed low incidence of regional or distant spread in peripheral tumours. In addition, tumour location did not influence DMFS presumably, again, because of the small metastatic potential of early stage NSCLC.
While some investigators observed independent influence of T stage (Kaskowitz et al. 1993; Gauden et al. 1995; Graham et al. 1995; Jeremic et al. 1997a, b; Zhao et al. 2007), others did not (Morita et al. 1997; Cheung et al. 2000; Jeremic et al. 2005, 2006), and in some studies the results remained unchanged when tumour volume was additionally investigated. Kupelian et al. (1996) could not document that T stage influenced OS, DSS or LRFS, but found that tumours >5 cm had better DSS and those >4 cm had better LRFS. Yamada et al. (2003) found independent influence of tumour size (<3 cm vs. >3 cm) on LRFS but not on CSS. In addition to that, Hayakawa et al. (1996) found that maximal tumour diameter <5 cm independently predicted improved OS. In the study of Fang et al. (2006), tumour size (>3 and >4 cm, respectively) did not independently influence OS. However, T2 tumours predicted poor OS and LRFS, while tumours >4 cm predicted poor DMFS. If there is an influence of T stage/size on the outcome, this should happen first at local (T) level. Then, through improved LRFS, possible improvements of OS could be achieved, providing a causal relationship between LC and OS, especially in tumours with low metastatic potential.
As described previously (Jeremic et al. 2006), stage grouping (stage I vs. stage II, i.e. N0 vs. N1) did not influence any endpoint in our study. The same was observed when other investigators looked at different endpoints (Hayakawa et al. 1992, 1996; Yamada et al. 2003). Although N component (N0 vs. N1) should play a role in determining outcome, this may occur first at the level of isolated regional recurrence free survival (RRFS) or perhaps combined local–regional recurrence free survival, then on OS and/or DMFS. However, RRFS may not be an important endpoint since there is very low incidence of isolated nodal recurrences, 0–7 % in a comprehensive review of the literature (Jeremic et al. 2002). Unfortunately, also the vast majority of series concentrated on stage I NSCLC exclusively, while those including both stages did not undertake additional subgroup (N status) analysis.
Regarding histology, Sibley et al. (1998) found an improvement in CSS for squamous cell carcinoma (SCC). Gauden et al. (1995) observed improved OS and RFS for the mixed (adenocarcinoma (ADC)/SCC) histology, while Lagerwaard et al. (2002) observed an independent and favourable influence of unknown histology (vs. SCC and non-SCC) on OS. Our previous analysis (Jeremic et al. 2006) showed that SCC favourably predicted both OS and LRFS, but not DMFS. In the study of Fang et al. (2006) non-SCC histology did not independently predict OS, but predicted improved LRFS. Ishikawa et al. (2006) showed no effect of histology on OS, but ADC had better LRFS and worse DMFS than SCC. Unfortunately, no multivariate analysis was done to confirm the adverse influence of ADC. All other studies observed no such effect (Sandler et al. 1990; Hayakawa et al. 1992; Rosenthal et al. 1992; Dosoretz et al. 1992; Dosoretz et al. 1993; Slotman and Karim 1994; Slotman et al. 1996; Hayakawa et al. 1996; Jeremic et al. 1997a, b; Firat et al. 2002a, b; Jeremic et al. 2005; Chen et al. 2006). SCC had long been suspected of being more localized and having somewhat lower metastatic potential, when compared with other NSCLC. This was already brought to attention in locally advanced tumours (Cox et al. 1999); SCC was more likely to progress at the primary site than large cell carcinoma (LC), whereas ADC and LC progressed more frequently in the brain. Treatment intensification led to better OS, CSS and LRFS, but not DMFS. This may have been due to predominantly local failures in these patients, but also due to fewer events in the DMFS analysis. Higher RT doses were frequently shown to independently predict improved outcome of patients with early stage NSCLC (Cooper et al. 1985; Hayakawa et al. 1992; Dosoretz et al. 1992; Graham et al. 1995; Hafty et al. 1988; Zhang et al. 1989), although not unequivocally (Sandler et al. 1990; Kaskowitz et al. 1993; Slotman and Karim 1994; Slotman et al. 1996; Kupelian et al. 1996; Morita et al. 1997; Sibley et al. 1998). This was presumably due to somewhat narrow dose ranges used in some studies, as well as due to a mixture of different RT doses and tumour sizes/volumes in some reports. Some studies found an improvement in OS for higher RT doses, but the influence was marginally insignificant either on CSS or LRFS (Lagerwaard et al. 2002; Yamada et al. 2003; Chen et al. 2006). Similarly to own study findings, the addition of chemotherapy (CHT) to RT did not yield a benefit in the CSS analysis in the study of Yamada et al. (2003). Table 3 shows recent data from stereotactic radiotherapy trials. Such treatment results in high local control rates, even in patients with considerable comorbidity who are not candidates for surgery, and is currently being investigated in randomized clinical studies.
Table 3
Prognostic factors from the literature of stereotactic body radiotherapy (SBRT) for stage I non-small cell lung cancer
Author | Number of patients | SBRT regimen | Analysis | Prognostic factors |
---|---|---|---|---|
Matsuo et al. (2011) | 101, histologically confirmed | 4 fractions | Multivariate | Tumour diameter (LP, DP, OS) Age (DP) Gender (OS) |
Fakiris et al. (2009) | 70, histologically confirmed | 3 fractions | Univariate | No impact of tumour volume, T stage or localisation (OS) |
Andratschke et al. (2011) | 92, histologically confirmed | 3–5 fractions | Multivariate | No impact of tumour volume, T stage or localisation (OS) T stage (LP) |
3 Prognostic Factors in Locally Advanced (Stage III) NSCLC
Of patient-related factors, age as prognostic factor was evaluated using multivariate analysis with conflicting results. While two Radiation Therapy Oncology Group (RTOG) data base evaluations (Komaki et al. 1998; Werner-Wasik et al. 2000a, b) showed that age > 70 years is an adverse prognostic factor for survival, some studies (Socinski et al. 2004; Basaki et al. 2006) did not find any influence, including one of our own studies which used 60 years as cut-off point. Contrary to this our first analysis (Jeremic et al. 1995) showed that age < 60 years (vs. > 60 years.) carried better prognosis, which was confirmed in our later study (Jeremic et al. 1996). Interestingly, subsequent analysis of RTOG 9410 (Langer et al. 2001) showed better survival for elderly, and subgroup analysis of a large meta-analysis (Auperin et al. 2006) confirmed this finding. In the absence of proven influence on increased treatment-related toxicity and given the current lack of any information suggesting that tumours may have different biological characteristics and clinical course in elderly versus non-elderly, age cannot be considered as important prognosticator in this disease. Investigation of gender as prognostic factor also brought conflicting results. While CALGB (Socinski et al. 2004) and RTOG analyses (Komaki et al. 1998; Werner-Wasik et al. 2000a, b) did not find any influence, another RTOG analysis which focused on patients with supraclavicular node metastasis treated with combined modality therapy showed that female gender carried improved prognosis (Machtay et al. 1999). This was also a consistent finding in our own studies (Jeremic et al. 1995, 1996). Again, this prognostic factor can be considered a weak one, especially in the absence of any explanation why this disease could be easier to cure in females. Performance status seems to be the most frequently investigated prognostic factor. While initial observations of its importance were made more than 30 years ago (Edmonson et al. 1976; Stanley 1980), subsequent studies regardless of the stage of the disease or treatment used, generally confirmed these observations. In particular, in two major RTOG studies using recursive partitioning analysis (RPA), performance status was always the strongest prognosticator which then was used to develop classes (Komaki et al. 1998; Werner-Wasik et al. 2000a, b). In the largest analysis so far, performance status confirmed its leading role in predicting survival of patients treated with RT and/or CHT in 1999 patients enrolled in several studies (Werner-Wasik et al. 2000a, b). Data from CALGB (Socinski et al. 2004) also showed its strong independent influence on survival, as did studies by Firat et al. (2002a, b) and Jeremic et al. (1995, 1996). Compared to other parameters, PS is likely the most important determinant of outcome (Pfister et al. 2004), frequently reflected in the fact that clinical trials designed in the last decades usually use it to stratify patients treated with different approaches, including RT-CHT. Another factor, which has frequently been evaluated, is weight loss. Different cut-off values were used such as 5 or 8 %. While Stanley (1980) identified weight loss as an important prognostic factor in 5000 patients with lung cancer, some contemporary studies failed to observe this correlation (Socinski et al. 2004; Ball et al. 2002). The majority, however, did so (Komaki et al. 1998; Werner-Wasik et al. 2000a, b; Pfister et al. 2004), including our previous studies (Jeremic et al. 1995, 1996).
Among tumour-related factors, stage was frequently investigated. Similarly to our previous findings (Jeremic et al. 1995, 1996) more recent studies showed improved survival for stage IIIA patients. This can be seen as indirect confirmation of earlier observations by Stanley (1980), although a CALGB study (Socinsky et al. 2004) surprisingly showed better outcome for stage IIIB patients. As the authors correctly pointed out, even current staging systems and stage groupings are not perfect, for example regarding the dependence on size and not volume of the tumour and/or nodes in the designation process. In addition, tumour volume may actually be a more important means of investigating the potential influence of T and N components and, therefore stage. It was clearly shown that both total tumour volume or primary tumour volume (Basaki et al. 2006) can be considered as independent prognostic factors for survival. However, in that study other endpoints (local/regional PFS) were not used to prove such relationship in a causal way, as improvement in local/regional control should lead to an improved OS. The most recent staging classification of the International Association for the Study of Lung Cancer (Goldstraw et al. 2007) (Table 2) provides a new framework for further evaluation of the T, N, and M stages as well as stage grouping. While still being imperfect, it represents an ongoing effort of the community of thoracic oncologists to revise stage groupings based on a large body of data. One of the tumour-related factors that have occasionally been investigated upon its independent influence on survival is histology. While one RTOG study (Machtay et al. 1999) showed no influence of histology, similarly to the observation by Basaki et al. (2006), another RTOG study (Werner-Wasik et al. 2000a, b) clearly identified non-LC histology as the one having better prognosis. In our own experience LC carried an unfavourable prognosis while differentiation between SCC and non-SCC histology proved to be important. In a pooled RTOG studies analysis (Cox et al. 1999), histology was related to pattern of failure. ADC had greater risk for distant metastasis than SCC, although in our study (Jeremic et al. 2011) LC fared even worse. Also, it was shown by the RTOG authors that ADC progressed at the primary site more often than either SCC or LC carcinoma, which is only partially in agreement with our findings (Jeremic et al. 2011). Regardless of this, the RTOG study (Cox et al. 1999) clearly emphasized the importance of histology for treatment outcome and suggested separation of SCC from both ADC and LC carcinoma.
Of treatment-related factors, interfraction interval in hyperfractionated (Hfx) RT regimens was shown to be an independent prognosticator of treatment outcome (OS and LPFS) (Jeremic and Shibamoto 1996, Jeremic et al.2004). Contrary to our repeated findings, RTOG (Werner-Wasik et al. 1999) did not confirm any influence of interfraction interval on treatment outcome. However, this study suffered from serious shortcomings which we discussed earlier (Jeremic et al. 2004). First, definition of interfraction interval (mean of all daily intervals) was not optimal because a patient could be assigned an interval of 6 h when half of the treatments were given with 4 h and half with 8 h. Second, a number of CHT regimens were used, including concurrent and induction administration. Third, patients with stage II were included as well, and no separate analysis was provided for them. Fourth, no information on the influence of interval on endpoints other than oesophageal acute and late toxicity was provided. Fifth, only 3 pretreatment prognostic factors were correlated with 2 treatment-related factors (interval and CHT). Finally and most importantly, no information was provided on the influence of interval on local control, a vital part of this analysis, since interval might influence overall survival by influencing local control first. Concurrent CHT was proven to be an independent prognosticator of survival in several analyses. This is in contrast with findings by Ball et al. (2002) and Basaki et al. (2006), but is in agreement with the available data from RTOG and CALGB (Werner-Wasik et al. 1999; Socinsky et al. 2004) and findings from our previous studies (Jeremic et al. 1995, 1996). This has also been recently confirmed by the outcome of a meta-analysis that showed a significant advantage of concurrent RT-CHT over RT alone (Auperin et al. 2006). Only rarely published analyses included not only OS but two additional endpoints, namely LPFS and DMFS. Results of our most recent multivariate analysis (Jeremic et al. 2011) using LPFS as an endpoint mimicked those of OS, and confirmed our previous findings (Jeremic et al. 1995).
In patients not suitable for radical radiotherapy who were included in a randomised study of different palliative RT regimens, PS, weight loss and appetite loss were the most important prognostic factors for survival (Sundstrom et al. 2006). For example, 2-year survival was 22 % (no appetite loss) versus 3 % (appetite loss) in patients irradiated to 42 or 50 Gy.
4 Predictors of Response to Induction Therapy in Locally Advanced NSCLC
Although controversial, induction therapy followed by surgery in locally advanced NSCLC has been evaluated in many studies. The investigators aimed to define the most appropriate group for this approach, i.e. patients benefitting in terms of survival. Among the patients entering a course of induction CHT or RT-CHT, not all can complete the prescribed or planned procedure. Cerfolio et al. (2008) evaluated 402 patients with biopsy-proven, non-bulky N2 disease who underwent neoadjuvant RT-CHT and surgery. Among these patients 81 % completed their neoadjuvant therapy, 50 % returned for definitive pathologic restaging and 37 % underwent thoracotomy for attempted resection. Multivariate analysis found that only age younger than 70 years, more than one lymph node involvement and response to neoadjuvant therapy remained significant predictors of moving forward to the surgical arena after neoadjuvant therapy. The 5-year survival was 8 % for the 253 patients who did not return for restaging but was 47 % for the 149 patients who underwent thoracotomy (p < 0.001). The 5-year survival for selected subgroups of patients who underwent complete resection was 42 % for the 14 patients who had unsuspected residual N2 disease, 49 % for the 65 patients who had a partial response (PR), and 53 % for the 34 patients who had a complete response (CR).
Mediastinal downstaging after induction therapy is one of the most important and evaluated factors for long term survival (Albain et al. 1995; Betticher et al. 2006; Bueno et al. 2000; De Waele et al. 2006). Patients with persisting mediastinal involvement after induction therapy have a poor prognosis and will usually not benefit from surgical resection. Therefore accurate staging of the mediastinum is of utmost importance to determine the appropriate subgroup of patients for surgical resection after induction. Either minimally invasive techniques (transthoracic fine-needle aspiration biopsy—FNAB, transbronchial needle aspiration—TBNA, endobronchial ultrasound with FNAB—EBUS, endoscopic esophageal ultrasound with FNAB—EUS) or invasive techniques (re-mediastinoscopy, video assisted surgery—VATS) can be used for this approach and have a sensitivity between 70 and 80 %. All of these approaches require experience within the field. The non-invasive restaging modalities with CT and PET have a rather low accuracy of 64 and 72 % (Annema et al. 2003; Herth et al. 2008; Kunst et al. 2007; De Waele et al. 2008, 2011; Marra et al. 2008; de Leyn et al. 2007). Betticher et al. (2003) for the Swiss Group for Clinical Cancer Research (SAKK) evaluated 90 potentially operable patients with stage IIIApN2 receiving 3 cycles of cisplatin plus docetaxel followed by surgical resection. Postoperative RT to 60 Gy was administered for positive resection margins or involvement of the uppermost mediastinal lymph node. Seventy-five patients (83 %) underwent resection. The overall response rate was 66 %, with 19 % pathological complete response (pCR). The median survival was 27.6 months and the 3-year survival was 33 %. Mediastinal nodal clearance and complete surgical resection were strong independent predictors of increased survival in a multivariate analysis.
A French group aimed to evaluate whether pCR in early stage NSCLC after platinum-based neoadjuvant CHT resulted in improved outcome, where pCR was defined by the absence of viable cancer cells in the resected surgical specimen. Among the 492 patients analyzed, 8.3 % achieved pCR and in the pCR group, 5-year overall survival was 80 % compared with 55.8 % in the non-pCR group (p = 0.0007). In multivariate analyses, pCR, SCC, weight loss less than or equal to 5 %, and stage-IB disease was found to be favorable prognostic factors of overall survival. Five-year DFS was 80.1 % in the pCR group compared to 44.8 % in the non-pCR group (p < 0.0001). SCC was the only independent predictor of pCR in the study population (Moulliet et al. 2012). All patients with pCR were clinically considered to be responders, either CR (n = 5) or PR (n = 36). These results confirm the previously reported findings, showing that cCR was predictive of pCR, and that CT assessments likely underestimate pCR rate (Milleron et al. 2005).
In a very recent retrospective analysis by Lococo et al. (2013) the long term results of the patients with pCR after induction therapy followed by surgery for locally advanced NSCLC were evaluated. Among the 195 patients treated with induction therapy, 137 were operated with radical intent. Among these, 27 % showed a pCR. Within this pCR group the overall 3- and 5-year long-term survival and disease-free survival rates were 67 and 64 %, and 68 and 71 %, respectively. Initial single N2 station involvement (p = 0.010), resection to a lesser extent than pneumonectomy (p = 0.005) and adjuvant therapy (p = 0.005) were found to be predictors of increased 5-year survival. Most of the recurrences in this cohort were distant.
One of the most important factors for the survival outcomes after surgery preceded by induction therapy is postoperative mortality. Several studies reported increased mortality after surgery with induction treatment, especially with concurrent RT-CHT, and with pneumonectomy. The Memorial Sloan Kettering Cancer Centre group updated their operative mortality and the factors associated with this from their surgical database of 549 patients. All received CHT, and 17 % also had radiation. The most common procedures were lobectomy (71 %) and pneumonectomy (13 %). Hospital mortality was 1.8 %, with only one death after right pneumonectomy. Multivariate analysis showed that predicted postoperative pulmonary function tests (predictive postoperative product, predicted postoperative diffusion capacity, and preoperative percentage of predicted postoperative diffusion capacity) were important indicators for postoperative morbidity and mortality (Barnett et al. 2011).
Perioperative mortality was assessed in a meta-analysis after neoadjuvant therapy and pneumonectomy for NSCLC. Based on 27 studies, 30- and 90-day perioperative mortalities were 7 and 12 % in the entire cohort. Cumulative mortalities were 11 and 5 % for right and left pneumonectomies. Both 30- and 90-day mortality remained greater in right than left pneumonectomy (p = 0.02). Among 11 studies providing both 30- and 90-day mortalities, mortality difference was 5 % (p < 0.0001) (Kim et al. 2012).
The predictive effect of nutritional parameters was investigated in a retrospective European study. Fifty-one patients with locally advanced NSCLC undergoing concurrent RT-CHT followed by surgery were evaluated. Postoperative complications occurred in 49 %. Weight loss ≥5 % during induction period was associated with shorter OS (p = 0.03), and especially overweight patients experiencing weight loss ≥5 % during the induction period had shorter OS and also PFS (van der Meij et al. 2011).
5 Predictors of Toxicity of Definitive Radiotherapy and Radiochemotherapy
5.1 Lung
Lung is one of the most sensitive tissues to ionizing radiation. Damage to normal lung tissue remains the most important limitation for effective treatment with RT or RT-CHT of this disease, apart from rapid progression. Recent decades brought many radiobiologically and/or technologically oriented RT dose intensification approaches. Moreover, concurrent RT-CHT became standard treatment in locally advanced NSCLC and limited disease (LD) SCLC. With these, toxicity was observed more frequently, both acute and late, leading sometimes to not only serious adverse events (grades 3–4), but also toxic deaths (grade 5). Therefore reducing RT or RT/CHT induced normal lung tissue damage is of critical importance in improving the therapeutic window and patients’ quality of life. Acute lung injury starts from 1 to 3 months after RT and the most common symptom is severe dyspnea. Chronic lung damage which is usually irreversible evolves 6–24 months after irradiation. Prolonged follow-up is especially important as it enables better insight into events that may initially not be reported. In one such report, Miller et al. (2005) reported on an unexpected rate of bronchial stenosis using 1.6 Gy bid up to 70.8–86.4 Gy in NSCLC. Eight out of 103 (8 %) patients experienced clinically significant and symptomatic bronchial stenosis 2–48 months after RT, with a 1- and 4-year actuarial rate of 7 and 38 %, respectively. In stereotactic hypofractionated radiotherapy, the radiation tolerance of central structures including trachea and large vessels must also be considered. In general, radiographic abnormalities are seen more frequently than clinical symptoms which can be defined as haziness in the areas irradiated, a shift in the mediastinum and diaphragm of the irradiated area and pericardial and pleural fluid accumulation.
A number of clinical factors were investigated in order to determine their influence on the occurrence of bronchopulmonary and other toxicity in lung cancer patients treated with either RT alone or RT-CHT. Our previous multivariate analyses (Jeremic et al. 2004, 2012) in patients with stage III NSCLC treated with Hfx RT and concurrent low-dose CHT identified no clinical variable influencing acute grade ≥3 bronchopulmonary toxicity. Robnett et al. (2000), however, have found correlations with both female gender and KPS, while Rancati et al. (2003) and Claude et al. (2004) did not find that gender influenced bronchopulmonary toxicity. Brooks et al. (1986) and Claude et al. (2004) did not find correlations between toxicity and KPS. Except for Claude et al. (2004) who found adverse influence of age on the rate of symptomatic pneumonitis, other authors (Rancati et al. 2003; Brooks et al. 1986; Hernando et al. 2001; Quon et al. 1999) did not find such predictive influence. Regarding the use of CHT, Robnett et al. (2000), Lee et al. (1996), Inoue et al. (2001), and Hernando et al. (2001) did not find influence of CHT, while Brooks et al. (1986), Robert et al. (1999), Rancati et al. (2003), Yamada et al. (1998) and Byhardt et al. (1998) did so. Similarly to the latter, Singh et al. (2003) found adverse influence of concurrent CHT, and Werner-Wasik et al. (2000a, b) confirmed their finding in a multivariate analysis. In that study, even more profound negative influence of concurrent CHT was found when it was combined with Hfx RT. Moreno et al. (2007) could not identify any of the investigated clinical and therapeutic factors to be associated with bronchopulmonary toxicity, admittedly due to low incidence of these events. Similarly a Swedish group (De Petris et al. 2005) found no influence of age, gender, PS and stage on bronchopulmonary toxicity of concurrent RT-CHT. In other studies, total dose, fractionation and volume were occasionally identified as influencing bronchopulmonary toxicity. Interestingly, Roach et al. (1995) found somewhat favourable effect of Hfx RT on bronchopulmonary toxicity. Weight loss and concurrent CHT independently influenced occurrence of late grade >3 bronchopulmonary toxicity, contrasting our previous study which identified only stage as independent predictor of high-grade late bronchopulmonary toxicity (Jeremic et al. 2004).
Another set of measures of RT-induced lung toxicity include changes in pulmonary function tests (PFTs) such as Forced Expiratory Volume in 1 s (FEV1), Forced Vital capacity (FVC), and carbon monoxide diffusion capacity (DLco). A decline in PFTs was apparent at 6 months and continued well beyond 1 year (Miller et al. 2003), suggesting progressive RT-induced lung disease. In addition, others observed dose-dependent reduction in regional perfusion with prolonged follow-up post-RT, with most of this injury being manifest within 12 months post-RT (Woel et al. 2002).
Over many years various dosimetric parameters have been evaluated for RT-induced pulmonary toxicity. Of these, mean lung dose (MLD), the Vdose and Normal Tissue Complication probability (NTCP) are most commonly reported. MLDs of ≤15 Gy, 17.5–20 Gy, 22.5–25 Gy, and ≥27.5 Gy resulted in 0, 13, 21, and 43 % incidence of all grades of radiation pneumonitis (Oetzel et al. 1995). In a pooled analysis of 540 patients who received thoracic radiation by Kwa et al. (1998), the MLD was found to correlate with an increased risk of pneumonitis. A commonly recommended limit is to keep the MLD ≤ 20 Gy. The cut-off values of the percent volume of the total lung receiving a dose greater than e.g. 10 Gy (V10), 20 Gy (V20), 25 Gy (V25), 30 Gy (V30), 40 Gy (V40) or 50 Gy (V50) were reported by numerous authors, and such values were frequently found to be associated with radiation induced pneumonitis. Unfortunately, different endpoints (severity of pneumonitis) were used (Hernando et al. 2001; Claude et al. 2004; Armstrong et al. 1995; Graham et al. 1999) obscuring the picture. It is commonly recommended to limit V20 to ≤35 Gy (≤20 Gy for preoperative RT-CHT). Correlations between calculated NTCP values and the risk of pneumonitis were documented in several studies. In one study (Martel et al. 1994), NTCP average values were 73 % in patients with pulmonary toxicity and 25 % in patients without it. Similarly, using Grade >2 pneumonitis as an endpoint, patients with and without it had NTCP values of 19.6 and 12.0 %, respectively (Hernando et al. 2001). In a study by Lee et al. (2003), the mean NTCP value for the ipsilateral lung was higher in the group with pneumonitis (66.0 %) versus the group without pneumonitis (26.4 %). In other studies (Oetzel et al. 1995; Armstrong et al. 1995), various cut-off values of NTCP (>12 and 30 %, respectively) were used, showing that values higher than the cut-off value in both studies led to higher incidence of pneumonitis than that observed with NTCP values lower than cut-off value. Due to inconsistent data existing in the literature of these dosimetric factors, some embarked on combining them with clinical and location-related factors into a single predictive model for radiation pneumonitis (Hope et al. 2004). Recently, Hope et al. (2006) showed that models most frequently selected included tumour position, maximum dose, and D35 (minimum dose to the 35 % volume receiving the highest doses) (R = 0.28). The most frequently selected two- or three-parameter models outperformed commonly used metrics, including V20 and MLD (R = 0.18). More recently, in a proposed nomogram Bradley et al. (2007) evaluated a number of possible predictors of pneumonitis. The final model incorporated two effects: greater risk due to irradiation of inferior parts of the lung, and greater risk for increasing normal lung mean dose. Similarly, a multi-institutional study (Kocak et al. 2007) suggested a model which included parameters such as MLD, RT dose to perfused lung and pre-RT lung function to form low versus high risk group of patients experiencing RT-induced toxicity. Though this study was unable to accurately segregate patients into these two risk groups on a prospective basis, considered retrospectively, the data were consistent with prior studies suggesting that dosimetric and functional parameters are predictive of RT-induced pneumonitis.
Biochemical detection markers have also been investigated upon their predictive role in RT-related pneumonitis. Interleukin (IL)-1α and IL-6 (Chen et al. 2001, 2002), Soluble Intracellular Adhesion Molecule (SICAM)-1 (Ishii and Kitamura 1999), Serum mucin-like glycoprotein antigen Kl-6 (Goto et al. 2001
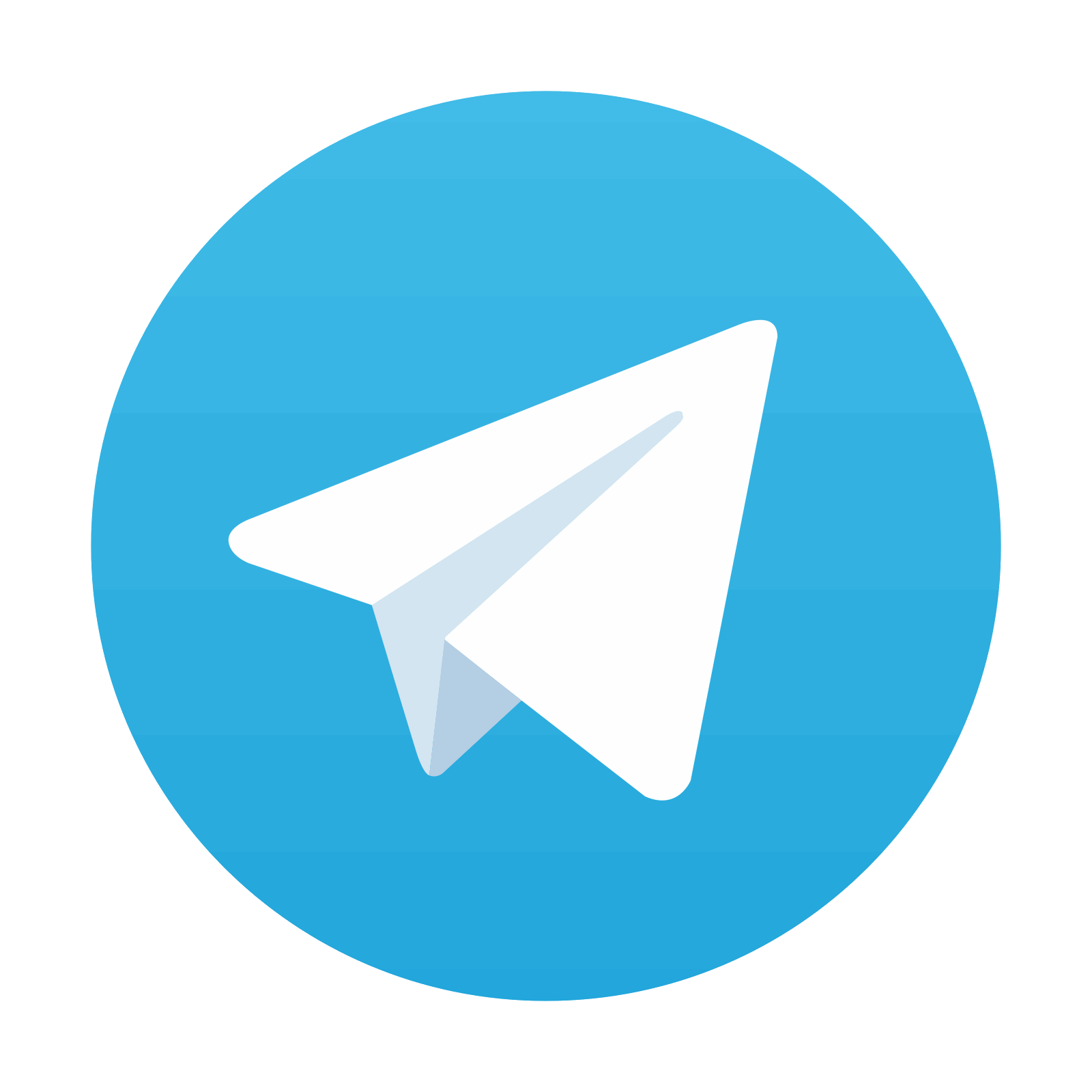
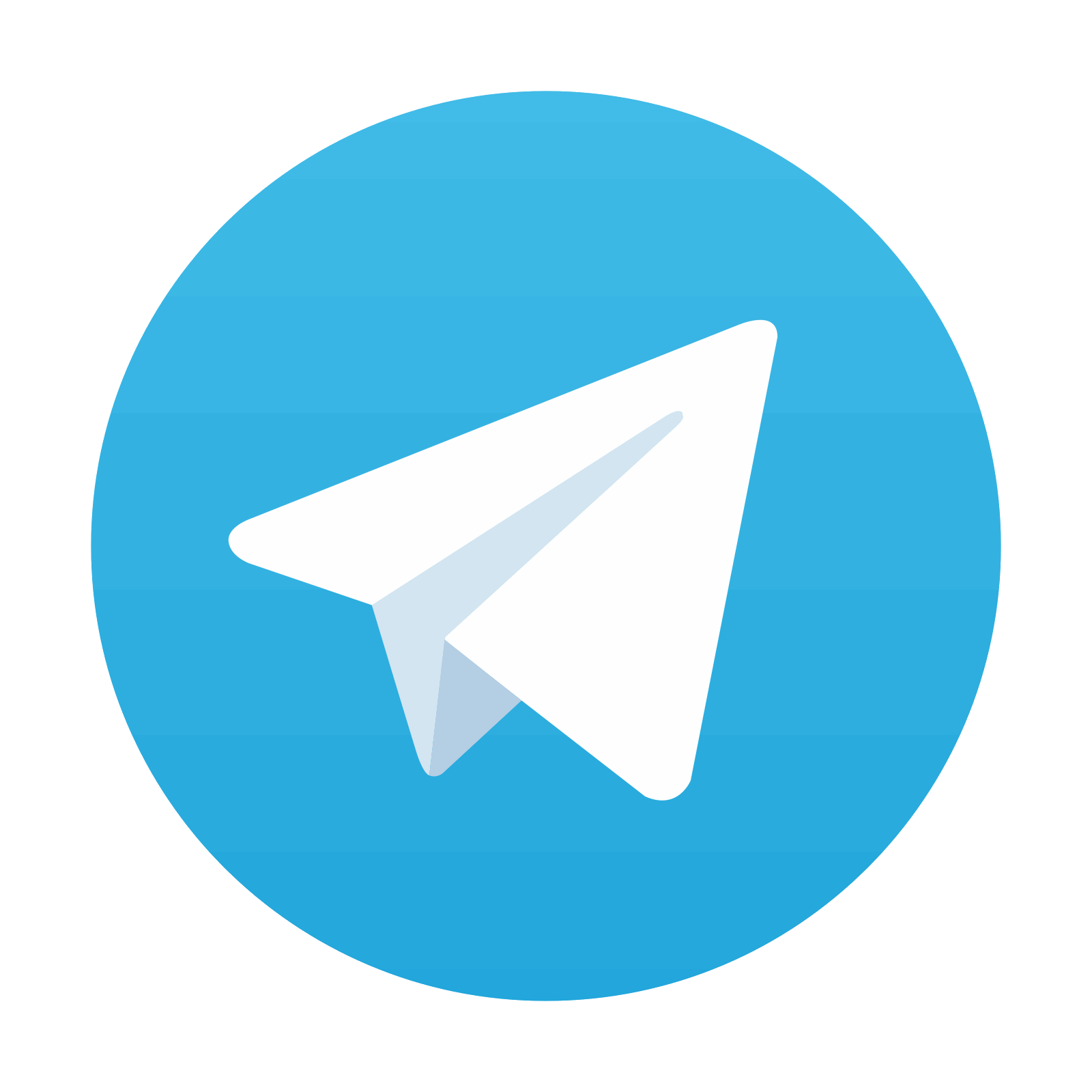
Stay updated, free articles. Join our Telegram channel

Full access? Get Clinical Tree
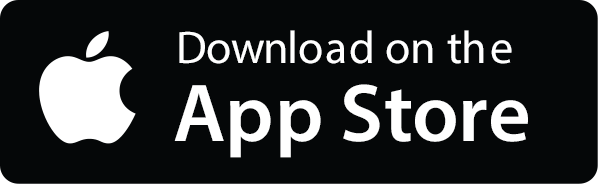
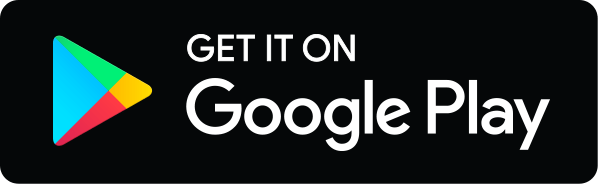