(1)
Department of Clinical Radiology, Amiri Hospital – Kuwait City, Kuwait City, Kuwait
5.1 Acute Chest Pain
5.1.1 Acute Coronary Syndrome
5.1.2 Acute Pulmonary Embolism
5.1.3 Aortic Dissection
5.2.1 Thoracic Aortic Aneurysm
5.2.2 Pulmonary Hypertension
5.2.3 Coral Reef Aorta
5.3.2 Dilated Cardiomyopathy
5.4 Endocarditis
5.4.1 Infective Endocarditis
5.1 Acute Chest Pain
Acute chest pain is one of the most common complaints encountered in medical emergency departments. Chest pain is divided into cardiac and noncardiac chest pain. Causes of cardiac chest pain include angina pectoris (stable and unstable), myocardial infarction (MI) (ST-segment elevation and non-ST-segment elevation), myocarditis, etc. Noncardiac chest pain includes diseases of the great vessels, esophagitis, pneumonia, etc.
This topic discusses the use of radiology in detecting acute chest pain and how the radiologist can contribute in assessing causes of acute chest pain in emergency departments.
Acute Coronary Syndrome
Acute coronary syndrome (CAS) is a term used to describe symptoms and manifestations of myocardial ischemia induced by coronary artery disease.
The most important components of CAS are angina pectoris and its severe complication MI. Angina pectoris is a term used to describe transient myocardial ischemia in the absence of myocardial cell death. In contrast, MI is a term used to describe myocardial cell death and necrosis due to ischemia.
Patients with angina pectoris classically present with retrosternal chest pain, which radiates to the neck and the left shoulder, accompanied by a sensation of numbness in the fingers. Associated symptoms include tachycardia, dyspnea, and possibly arrhythmia. The chest pain in angina pectoris typically lasts <10 min in duration. Patients with MI classically present with the symptoms of angina pectoris in a severe fashion. The retrosternal chest pain is severe and associated with autonomic nervous system hyperactivity, causing profound sweating and at times loss of consciousness. The chest pain typically may last up to 30 min in duration.
MI can be transmural involving the whole thickness of the myocardial wall due to complete occlusion of the coronary artery. MI can also be subendocardial, which is classically seen in coronary arterial spasm and hypertension due to hypoperfusion. The vascular supply of the endocardium is the part of the heart wall that is most sensitive to hypoperfusion.
Cardiac CT is used in patients with acute chest pain to rule out three main conditions (triple rule out): acute MI, pulmonary embolism (PE), and aortic dissection. Cardiac CT can also be used to detect calcium plaques within the coronary arteries in a technique known as “calcium scoring.”
Calcium scoring is a method that quantifies the atherosclerotic plaques within the coronary vessels. The calcium score is used to assess the risk of heart events, not to detect coronary stenosis. The basic idea of calcium scoring is to perform a noncontrast CT of the heart to detect calcified plaques (Fig. 5.1.1). Once a calcified plaque is identified in the coronary arteries, the examiner encircles the plaque by a cursor, and a special program will measure the plaque attenuation and express it as a number in Hounsfield units as a score (Agatston score). Each coronary branch is measured separately and then the numbers added to give a total calcium burden score. The score predicts the probability of heart attacks in the next 5–10 years on the current status of the patient without treatment modifications.
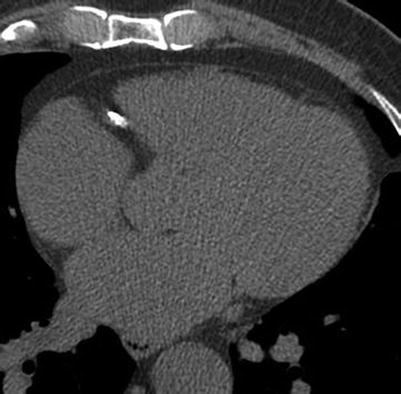
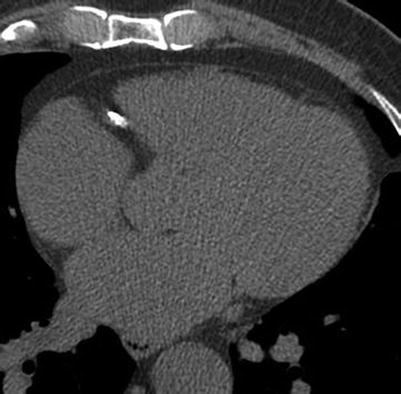
Fig. 5.1.1
Axial nonenhanced cardiac CT examination for calcium scoring shows calcified plaque in the right coronary artery
Uncalcified atherosclerotic plaques take up to 15 years before they are calcified and visualized in a calcium scoring study or noncontrast CT study. The uncalcified (vulnerable) plaque appears inhomogeneous or with low density on CT scan (25 ± 15 HU). Uncalcified and partially calcified plaques are more associated with ACS than are calcified plaques, because they are unstable and can be dislodged, initiating a coronary embolic attack.
Cardiac MRI in CAS patients is mainly used to study myocardial wall motion (myocardial function study), perfusion (same as the thallium perfusion study), viability, and ejection fraction measurement like cardiac Doppler study (phase-contrast flow quantification). The role of cardiac MRI postinfarction is to identify viable (salvageable) myocardium, which is mainly detected by the (myocardial viability study).
The basic concept of the myocardial viability study is to detect how much viable (alive and contractile) myocardium is left after MI. The technique depends upon the fact that gadolinium diffuses into the myocardial interstitial spaces after its injection into the body. As long as the myocardial membrane (sarcolemma) is intact, the gadolinium is pumped out of the intracellular compartment and concentrated in the extracellular compartment until it is washed out 10 min after its injection. This scenario occurs with normal and viable myocardium. If the myocardium is diseased or infarcted, the gadolinium will diffuse inside the extra- and intracellular compartments, which makes its clearance take longer time than 10 min. Myocardial contrast enhancement that exceeds 10 min from gadolinium injection is called “late gadolinium enhancement,” which is considered pathological, and the test is considered positive for nonviable myocardium.
Loss of cardiac wall motion, which is assessed in the myocardial function study, is another important sign of nonviable myocardium. However, there are two situations where the myocardium is viable but is not contracting: myocardial stunning and hibernating myocardium. Myocardial stunning is a situation where the cardiac muscles are viable, but they do not contract as a transient phenomenon after MI (like penumbra after stroke). Hibernating myocardium is a situation where the cardiac muscles are viable, but are not contracting after reestablishing coronary perfusion due to a long period of chronic perfusion abnormalities. This situation is typically seen in patients with long-standing, compromised coronary perfusion who have undergone coronary artery bypass surgery (CABG). Although the perfusion is normally established after a long period of hypoperfusion, the muscles are not contracting due to a long period of cardiac muscle ischemia and hypofunction.
Signs on Chest Radiographs
Indirect signs of CAS include aortic calcification of aorta or coronary arteries calcification.
If the MI is complicated by heart failure, signs of pulmonary edema may be detected such as upper lobe vessel cephalization and enlarged cardiac silhouette (Fig. 5.1.2).
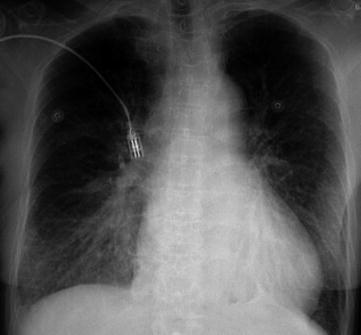
Fig. 5.1.2
Anteroposterior chest radiograph of a bedridden patient with myocardial infarction (MI) shows congested hilar vessels and beginning of pulmonary edema
Signs on Cardiac CT
In a patient with acute chest pain, detection of calcified plaques on the nonenhanced coronary vessels with absence of signs of aortic dissection or embolism confirms the diagnosis of CAS. However, absence of the coronary calcified plaques does not rule out CAS because uncalcified plaques can be present. Up to 50 % of patients with sudden cardiac arrests show calcified lesions in their coronary arteries (Fig. 5.1.1).
MI may be seen as a subendocardial ventricular hypodense area depending upon the blocked coronary artery and its vascular territory.
Post-myocardial infarction calcification and ventricular dilatation may occur (Fig. 5.1.3).
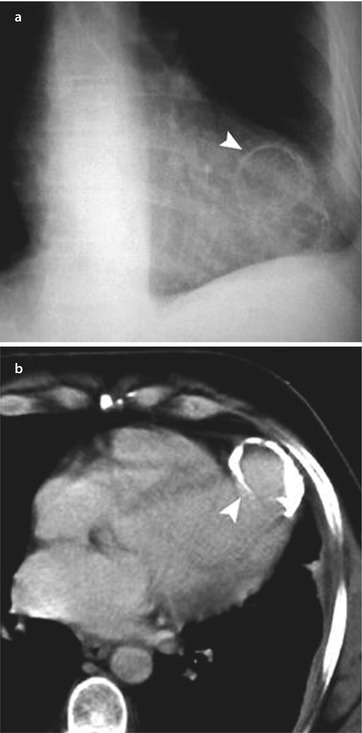
Fig. 5.1.3
Posteroanterior chest radiograph (a) and thorax CT (b) shows focal apical left ventricular dilatation with calcified rim due to old MI of this region (arrowheads)
Signs of MI on MRI
Wall motion abnormalities (akinesia or hypokinesia) on cine MRI.
Contrast enhancement on delayed images (>10 min). There are four patterns of late contrast enhancement of MI: First pattern is subendocardial enhancement with sparing of the subepicardial region (Fig. 5.1.4a). Second pattern is full-thickness myocardial wall enhancement (Fig. 5.1.4b). Third pattern is full-thickness myocardial wall enhancement, with subendocardial hypointense area, representing a severe edema compressing the intramural vessels (Fig. 5.1.4c). Fourth pattern is seen as a dark hypointense area that represents the infracted area surrounded by a rim enhancement (Fig. 5.1.4d). The fourth pattern is seen in extensive MI with less viable myocardium.
Stunned and hibernating myocardium is visualized as wall motion abnormalities (akinesia or hypokinesia) in cine MRI with no contrast enhancement on delayed images (>10 min).
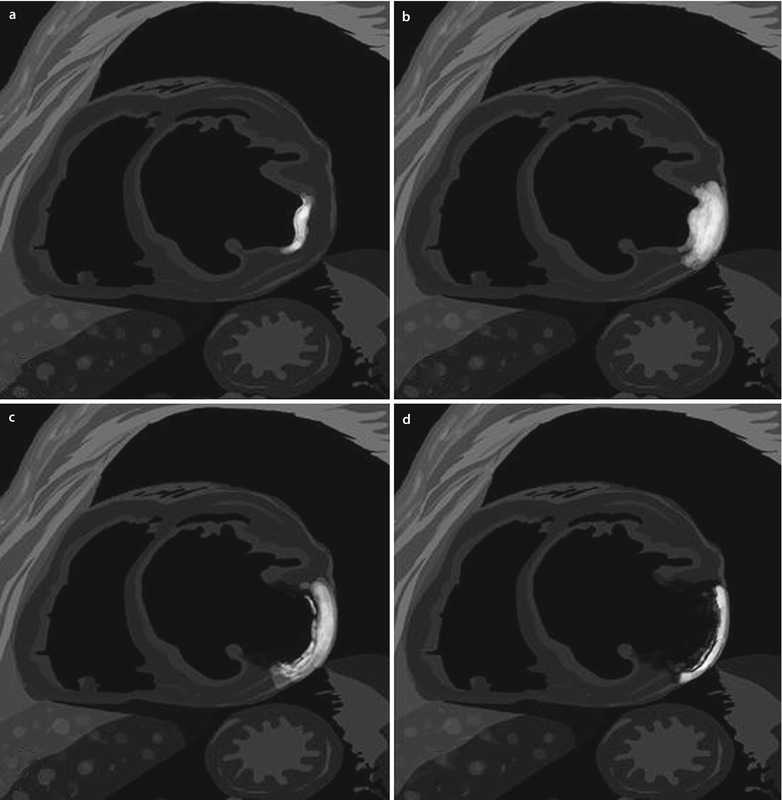
Fig. 5.1.4
Short-axis dark-blood T1W postcontrast cardiac MR illustrations show different pattern of myocardial enhancement after MI: (a) subendocardial enhancement with sparing of the subepicardial region; (b) full-thickness myocardial wall enhancement; (c) full-thickness myocardial wall enhancement, with subendocardial hypointense area; and (d) dark hypointense area represents the infarction surrounded by a rim enhancement
Why does atherosclerosis not develop in the veins and is only seen in the arteries, although the cholesterol circulates in the blood in both veins and arteries?
This occurs because the arterial pulsation assists in the deposition of the cholesterol molecules within the intima. Normally, the pulmonary arteries do not pulsate, but when pulmonary hypertension develops, the high pressure blood within the arteries evokes the arterial wall to pulsate, resulting in developing atherosclerosis within the pulmonary arteries.
Acute Pulmonary Embolism
Acute pulmonary embolism (PE) is an emergency situation characterized by closure of a pulmonary artery by an embolus causing pulmonary ventilation–perfusion mismatch or, in a worse scenario, pulmonary infarction.
The bronchial circulation only supplies nutrients and does not participate in gas exchange in normal situations. However, in PE, the bronchial circulation responds with enlargement and hypertrophy and participates in blood oxygenation due to decreased pulmonary flow and ischemia.
PE is categorized according to severity into two main types: acute sub-massive and acute massive PE. Acute sub-massive PE is characterized by <50 % occlusion of the pulmonary vascular bed, whereas acute massive PE is characterized by >50 % occlusion of the pulmonary vascular bed.
Patients with acute PE often describe acute sudden chest “gunshot-like” pain with progressive dyspnea, tachycardia, and cyanosis. Many patients have a history of deep venous thrombosis (DVT), varicose veins, immobilization, or recent pelvic surgery. A dislodged part of the initial thrombus, mostly from the lower limbs, travels through the venous circulation until it blocks an arterial pulmonary vessel in the chest as an embolus, causing pulmonary vascular congestion. If this congestion persists, pulmonary infarction occurs.
In small percentage of patients, the unresolved thrombus after treatment can be incorporated into the vessel wall and covered by a layer of epithelium. This thrombus organization causes intravascular stenosis of the affected lumen, resulting in the development of pulmonary hypertension and cor pulmonale.
Acute PE is best diagnosed by V/Q scan (ventilation–perfusion nuclear scan study) in circulatory stabilized patient. The scan typically shows pulmonary ventilation–perfusion mismatch. In unstable acute PE patients, CT pulmonary angiography is the initial examination of choice.
Signs on Chest Radiograph
Radiographs are normal in 12 % of cases.
Pulmonary infarction can appear as a patchy radio-opaque shadow on chest radiograph, which cannot be differentiated from patchy pneumonia or pulmonary contusions. Therefore, the radiographic signs have to be correlated with the history and the clinical data.
Hampton’s hump: is a wedge-shaped radio-opaque patch that is round shaped, located at the lung periphery, and directed from peripheral toward the hilum (Fig. 5.1.5). This patch represents wedge-shaped infarction of the peripheral lung parenchyma.
PE can be accompanied by Hemorrhagic pleural effusion.
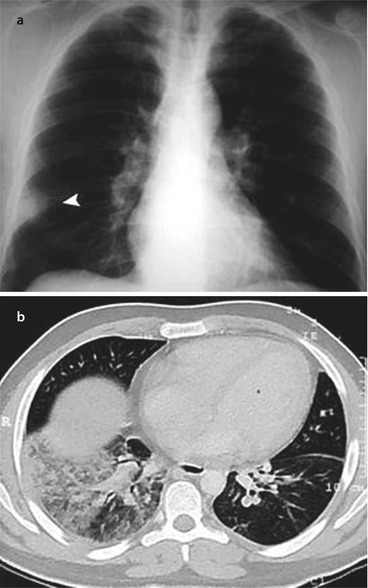
Fig. 5.1.5
Posteroanterior chest radiograph (a) and chest HRCT in two different patients with pulmonary infarction show Hampton’s hump in (a) (arrowhead) and basal area of pulmonary parenchymal consolidation due to infarction in (b)
Signs on Doppler Sonography
Doppler sonography should be performed for patients with PE or patients with high risk of DVT who show signs of respiratory distress (e.g., bedridden patients).
DVT is diagnosed on Doppler sonography when an intravenous echogenic material is detected (e.g., thrombus), the vein is distended and noncompressible (most specific and diagnostic sign), and there is loss of color duplex signal within the vein. The thrombus should be followed by the probe to detect its free edge, and an observation of labile, freely moving edge on real-time sonography should be reported. A labile, free edge thrombus has a high risk of embolization (Fig. 5.1.6).
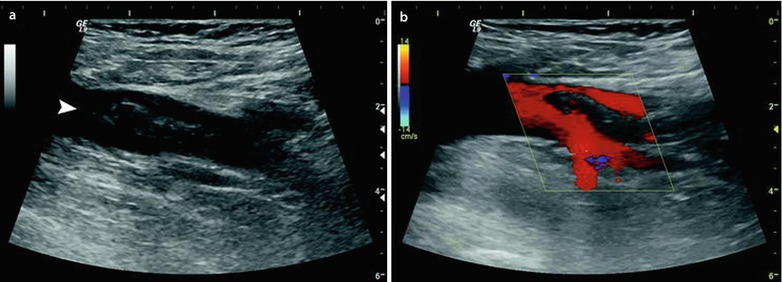
Fig. 5.1.6
Sagittal, Doppler sonography (a) and Duplex (b) images of a patient with DVT show hypoechoic material within the external iliac vein with free labile edge
Signs on CTA
PE is detected as complete filling defect with failure to enhance the entire lumen (complete thrombosis). The thrombosed vessel may be enlarged, and the thrombus may appear hyperdense on non contrast-enhanced images.
Partial filling defect of a pulmonary vessel surrounded by areas of contrast material enhancement (Fig. 5.1.7) may be seen.
Pulmonary infarction is visualized as a wedge-shaped area of lung parenchyma with high density located in the periphery of the lung, with the base lying along the pleura (Fig. 5.1.5).
Areas of lobar atelectasis in PE may show contrast enhancement.
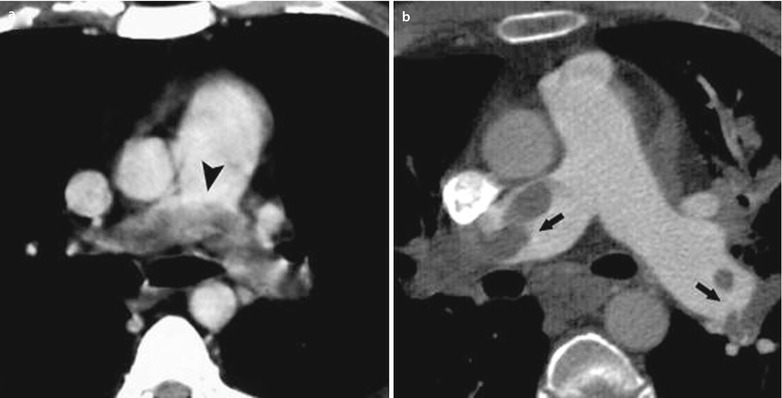
Fig. 5.1.7
Axial pulmonary CTA of two different patients (a and b) with pulmonary embolism (PE) shows saddle thrombus in (a) (arrowhead) and distal complete thrombosis of the right pulmonary artery with partial thrombosis of the distal part of the left pulmonary artery (arrow in b)
Chronic PE is visualized as a peripheral intra-arterial wall filling defect. Calcification of the organized thrombus may be seen.
Saddle thrombus is a term used to describe a big thrombus that abuts over the bifurcation of the main pulmonary arteries (Fig. 5.1.7).
Signs of right ventricular enlargement might be seen in CT with displacement of the ventricular septum toward the left ventricle, as a sign pulmonary hypertension.
Areas of mosaic lung parenchyma pattern with pruning of the pulmonary vessels may be seen (Fig. 5.1.8).
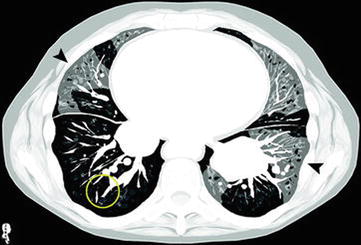
Fig. 5.1.8
Axial chest HRCT lung window illustration shows mosaic pulmonary parenchymal pattern (arrowheads) and pruning of the pulmonary arteries (yellow circle)
Aortic Dissection
The term acute aortic syndrome is applied to multiple acute chest pain presentations that are caused by thoracic aortic diseases, including aortic dissection, aortic intramural hematoma (IMH), and penetrating atherosclerotic ulcer.
Aortic dissection is a condition characterized by separation of the aortic intima with presence of blood in a false lumen between the intima and the medial layers of the aortic wall.
The intima is the innermost layer of the aortic wall. Aortic wall intimal tear starts typically at sites of highest intramural pressure and wall tension. After intimal tear, the blood flow inside the tear dissects its way between the intima and the media layer, creating a false lumen. The structure between the true and the false lumen is called “intimal flap,” which is the key diagnosis of aortic dissection on radiological examinations.
The most common predisposing factors of aortic dissection are systemic hypertension, bicuspid aortic valve, aortic coarctation, and Marfan’s syndrome. Patients typically present with sudden acute chest pain that is described as “tearing” sensation and classically radiation to the back.
Aortic Dissection Is Classified According to the Stanford and Debakey Classifications
Stanford Classification
Type A: this type involves the ascending aorta, and it is managed surgically. This type carries the risk of spontaneous rupture into the pericardium resulting in pericardial tamponade, or it can continue dissection to involve the coronary arteries (right coronary more than the left). Patients with this type can also develop aortic regurgitation (50 % of cases).
Type B: this type involves the descending aorta only. The site of dissection is typically just distal to the subclavian artery, near the insertion of the ligamentum arteriosum. When the dissection involves both the descending and the ascending aorta, it is classified as type A. This type is managed medically; however, in the current era, even type B is managed with endovascular stent across the origin of the dissection.
Debakey Classification
Type I involves ascending aorta only.
Type II involves the ascending and the descending aorta.
Type III involves the descending aorta only.
Differential Diagnoses and Related Diseases
Vascular Ehlers–Danlos syndrome is a disease characterized by joint hypermobility, skin abnormalities (e.g., easy bruising), fragility of intestinal and genitourinary organs, and vascular fragility leading to dissection or rupture of medium to large muscular arteries. The disease has an autosomal dominant mode of inheritance and caused by mutation in collagen type 3 gene (COL3A1). The dissection arises in vascular Ehlers–Danlos syndrome that occurs typically without preceding aneurysm.
Signs on Radiographs
There is mediastinal widening with obliteration of the aortic knuckle on plain radiographs.
Signs on CTA
The key diagnostic finding in aortic dissection is identification of the intimal flap, which appears as a thin “line” of soft tissue within the aortic lumen separating the false lumen from the true lumen (Fig. 5.1.9).
The true lumen shows higher enhancement than the false lumen, because filling of the false lumen is slower than the true lumen. Moreover, the false lumen may show signs of intravascular thrombosis.
In the ascending aorta, the false lumen is typically the more anterior lumen, while in the descending aorta, it is typically the more posterior lumen.
Coronary artery dissection can be suspected when the intimal flap is detected at or near the site of a coronary ostium. When this sign is identified, coronary CTA should be performed to detect the extension of the dissection.
Pericardial hemorrhagic effusion may be detected as highly attenuated fluid within the pericardial space (40–50 HU).
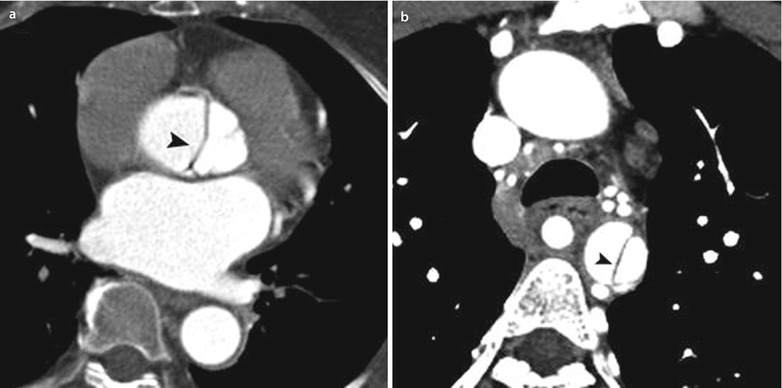
Fig. 5.1.9
Axial cardiac CTA of two different patients with aortic dissection Stanford type A (a) and Stanford type B (b) shows the classic intimal flap (arrowheads) separating the true from the false lumen
Aortic Intramural Hematoma
IMH is a condition characterized by rupture of the vasa vasorum, the network of vessels that supply the aorta itself, resulting in bleeding within the aortic wall, mostly within the media layer.
IMH is clinically indistinguishable from aortic dissection. Patients present with signs of acute aortic syndrome consisting of sudden chest pain that is radiating to the back or chest depending on which part of the aorta is affected. IMH accounts for 10–30 % of cases of acute aortic syndrome, and it may be caused by hypertension, blunt trauma, or penetrating atherosclerotic ulcer. In contrast to aortic dissection, no intimal tear flap is identified in this condition. However, the hematoma can progress into a true dissection if the aortic wall continues to enlarge in thickness by the hematoma >5 cm.
Signs on CTA
On contrast-enhanced scan, the aortic wall show a crescentic area of wall thickening that may show high attenuation if the bleeding is fresh. There is no intimal flap (Fig. 5.1.10).
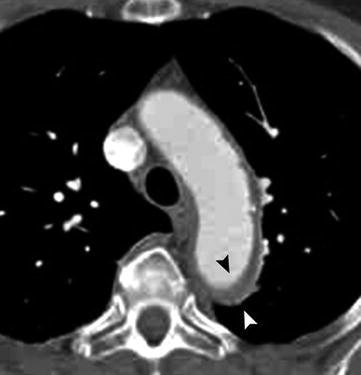
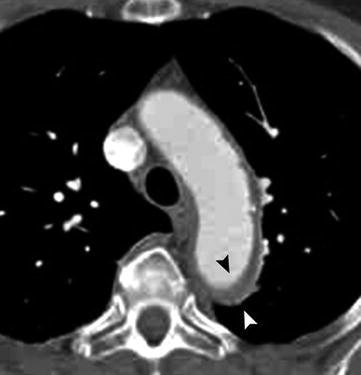
Fig. 5.1.10
Axial cardiac CTA shows posterior aortic arch focal area of aortic wall thickening due to intramural hematoma (arrowheads)
Penetrating Atherosclerotic Ulcer
Penetrating atherosclerotic ulcer is a condition that results from ulceration and break of an aortic atherosclerotic plaque resulting in an intimal defect. This defect causes bleeding within the aortic wall surrounding the ulcer, which will result in IMH formation or pseudo-aortic aneurysm formation.
Signs on CTA
The scan will show an area of intimal defect within the aorta with the formation of saccular pseudoaneurysm, IMH, or periaortic mediastinal hematoma (Fig. 5.1.11).
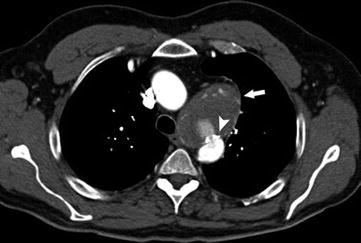
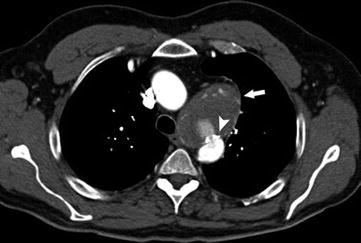
Fig. 5.1.11
Axial cardiac CTA shows an area of aortic wall ulceration of the descending thoracic aorta (arrowhead) with a jet of bleeding into the aortic wall creating a periaortic mediastinal hematoma (arrowhead)
Further Reading
Birchard KR. Acute aortic syndrome and acute traumatic aortic injury. Semin Roentgenol. 2009. doi:10.1053/j.ro.2008.10.002.
Castañer E, et al. Congenital and acquired pulmonary anomalies in the adult: radiologic overview. RadioGraphics. 2006;26:349–71.
Choe YH, et al. Comparison of MDCT and MRI in the detection and sizing of acute and chronic myocardial infarcts. Eur J Radiol. 2008;66:292–9.
De Becker J, et al. Marfan and Marfan-like syndromes. Artery Res. 2009;3:9–16.
Hoffmann U, et al. Cardiac CT in emergency department patients with acute chest pain. RadioGraphics. 2006;26:963–80.
Jeudy J, et al. Nontraumatic thoracic injuries. Radiol Clin N Am. 2006;44:273–93.
Oliver TB, et al. Spiral CT in acute non-cardiac chest pain. Clin Radiol. 1999;54:38–45.
Winter-Muram HT, et al. Suspected acute pulmonary embolism: evaluation with multi-detector row CT versus digital subtraction pulmonary arteriography. Radiology. 2004;233:806–15.
5.2 Diseases of the Great Vessels
The great vessels include the aorta, the superior and inferior vena cava, the pulmonary artery, and the pulmonary veins. There are multiple medical conditions affecting the great vessels that require imaging to assess their complications, establish their diagnosis, or monitor their therapy response. This topic discusses some of the common medical conditions where radiology plays an important role in their diagnosis and assessment.
Thoracic Aortic Aneurysm
Thoracic aortic aneurysm (TAA) is a disease characterized by dilatation of the wall of the aorta affecting its three layers (intima, media, and adventitia). In contrast, pseudo-aortic aneurysm is a condition characterized by saccular dilatation of the outer most layers of the aortic wall (media and/or adventitia) with an intact inner wall layer (intima).
The most common cause of TAA is atherosclerosis, while the most common cause of pseudo-aortic aneurysm is aortic trauma violating the wall integrity. TAA originates in the ascending aorta (50 %), descending aorta (40 %), and the aortic arch (10 %). In contrast, pseudo-aortic aneurysm usually arises at three basic levels: the aortic root, the aortic isthmus, and the aortic diaphragm.
Patients with TAA are typically in their 50s and 70s and usually are asymptomatic. Up to 30 % of patients present with complications due to TAA rupture. Pain or dysphagia due to mass effect over the adjacent mediastinal structure may be seen uncommonly.
TAA expands at a rate of 0.5 cm per year, with an increased risk of rupture when it is >5 cm in diameter. Patients with TAA >6 mm may present with spontaneous bleeding resulting in hemomediastinum or periaortic hematoma formation.
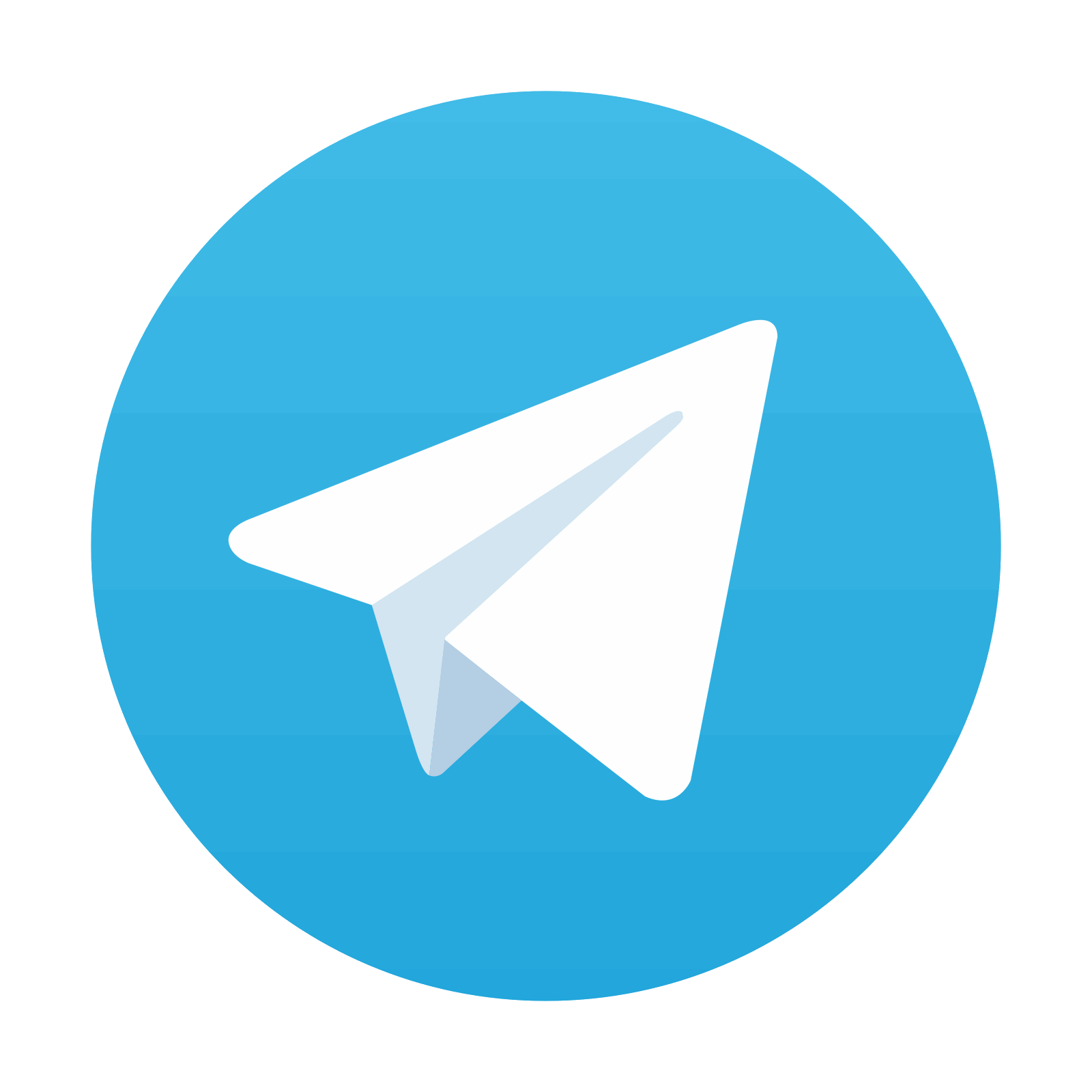
Stay updated, free articles. Join our Telegram channel

Full access? Get Clinical Tree
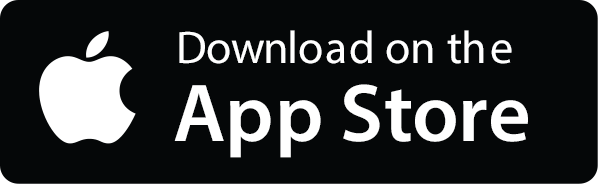
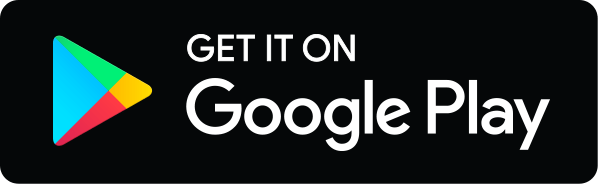