Abnormal chest X-ray
• Workup of patient with suspected COPD for consideration for lung volume reduction or transplant
• Assessment of possible aortic dissection, aneurysm, or aortic ulcer
• Characterization of diffuse lung disease
Chest X-ray is normal or noncontributary
• Identification of unrecognized lung disease in symptomatic patients
• Detection of metastatic disease or a suspected solitary pulmonary nodule
• Demonstration of pulmonary embolism
• Visualization of aortic dissection
• Investigation of the patient with fever, hemoptysis, shortness of breath, or wheezing
• Clinical disease likely to be thoracic in etiology, e.g., myasthenia gravis, carcinoid syndrome, or paraneoplastic syndromes
Radionuclide Imaging
Ventilation/perfusion (V/Q) scans are designed to diagnose pulmonary embolism (PE) and are the most commonly obtained nuclear study of the lungs [7, 8]. It involves simultaneous imaging of pulmonary blood flow and alveolar ventilation. According to a recent American College of Radiology Appropriateness Criteria Committee report, these scans are particularly sensitive for the diagnosis of chronic pulmonary thromboembolic disease. Today, they are also used to diagnose acute PE in patients who cannot undergo a contrast CT.
Magnetic Resonance Imaging
Imaging with MR presents unique challenges because of the low inherent MR signal intensity of lung and the deleterious effects of respiratory and cardiac motion on MR image acquisition. The tissue-air interfaces in lungs cause image distorting artifacts and loss of coherent signal. Motion artifact and image ghosting are more evident in studies performed on high field strength imagers compared with lower field strength magnets. Although careful manipulation of technical factors such as cardiac and respiratory gating can improve image quality, pulmonary MR remains difficult to achieve and for this reason indications for pulmonary MR remain limited [9]. Nonetheless, because MRI has excellent soft tissue resolution, it is useful for identification of tumor invasion into the chest wall and mediastinum. It is also useful to differentiate solid from cystic masses and to demonstrate diaphragmatic abnormalities [10].
MRI along with magnetic resonance angiography (MRA) has been shown to be highly accurate for the diagnosis of thoracic aortic disease, with sensitivities and specificities that are equivalent to those for MDCT and transesophageal echocardiography (TEE). Velocity-encoded phase contrast flow quantification techniques enable measurement of the differential flow velocity in the true and false channels and evaluation of aortic valve motion. Since no radiation is involved, MRI may be preferred over MDCT for patients who require repeated imaging. MRI is also useful to diagnose various causes of chest pain and may be helpful to characterize both ischemic and non-ischemic cardiomyopathies such as hypertrophic and dilated cardiomyopathy. Infiltrative cardiomyopathy due to sarcoidosis, amyloidosis, or tumor among other conditions may be characterized by MRI (Table 3.2).
Table 3.2
Clinical indications for thoracic MRI
• Aorta: Aortic dissection, aneurysm, aortitis, coarctation, cardiac valvular flow and function |
• Pulmonary arteries: Pulmonary embolism, vasculitis, pulmonary hypertension |
• Lung: Pancoast tumors, neoplastic chest wall invasion |
• Heart: Myocardial and pericardial disease |
• Mediastinum: Identification and characterization of neoplasm |
Angiography
In recent years, there has been a shift in the imaging strategy for diseases of the aorta from invasive catheter aortography to noninvasive approaches. MDCT angiography (CTA), echocardiography and MRI are currently the first line imaging tests for the diagnostic work-up of thoracic aortic pathology.
CT arteriography (CTA) is the most commonly requested diagnostic test for suspected acute or chronic thoracic aortic disease. Because aortic disease is commonly diffuse or multifocal, the scanning field typically extends from thoracic inlet to the aortic bifurcation. The imaging field may be extended further to involve the proximal femoral vessels, if intervention is contemplated. A non-enhanced scan is usually performed first to look for (a) high-attenuation acute intramural hematoma, (b) distribution of calcification, and (c) as a baseline to access enhancement of the aortic wall and para-aortic tissue. After rapid IV injection of iodinated contrast for the CTA portion of the study, images are acquired during patient “breath holding” to reduce respiratory artifacts. Electrocardiographic (ECG) gating is commonly used to reduce cardiac motion artifacts, particularly when evaluating the ascending aorta. In addition, ECG gating can facilitate visualization of the proximal coronary arteries. Electrocardiographic gating is turned off at the diaphragm to reduce both breath-hold time and radiation dose. Two dimensional (2D) and three dimensional (3D) reformatting techniques, such as multiplanar reconstruction (MPR), maximum intensity projection (MIP), volume rendering (VR), and direct endoluminal viewing, are used according to the clinical indications for the study (Table 3.3).
Table 3.3
Advantages and disadvantages of CT angiography
Advantages |
1. Easy availability, most often within or near the emergency department |
2. Short imaging time, easy patient monitoring while scanning |
3. Ability to image the entire aorta and its branches as well as organ perfusion |
4. Ability to study the aortic wall, and periaortic tissues |
5. Evaluate the rest of the chest and abdomen for a possible alternative diagnosis |
Disadvantages |
1. Motion artifact may mimic aortic pathology |
2. Image degradation by implanted devices, catheters, and leads |
3. Exposure to ionizing radiation and IV iodinated contrast |
Echocardiography
The primary role of transthoracic echocardiography (TTE) is to image the aortic root and ascending aorta. It does not consistently visualize the mid or distal ascending or the descending aorta. TEE has higher sensitivity and specificity than TTE [11]. TEE can visualize more of the aorta wall than TTE, but it may not visualize the distal ascending aorta, proximal arch, and the proximal abdominal aorta. TEE can be performed both at the bedside with the patient under sedation and intraoperatively. Ultrasound does not expose the patient to radiation and no injection of contrast material is necessary. Beyond establishing the presence of aortic pathology, echocardiography can also be used to evaluate concomitant cardiac disease. Given the semi-invasive nature of TEE, MDCT scan is favored for the routine imaging of stable patients.
Clinical Scenarios
Shortness of Breath
Shortness of breath (dyspnea), whether acute or chronic, most often is either pulmonary or cardiovascular in etiology. Frequent cardiovascular causes of dyspnea include acute coronary syndrome (ACS), congestive heart failure, and a variety of cardiomyopathies. Pulmonary etiologies include chronic obstructive pulmonary disease (asthma, emphysema, and chronic bronchitis), pulmonary hypertension, pneumothorax, PE, airway obstruction, and interstitial lung disease [12]. Distinguishing clinically between cardiovascular and pulmonary etiologies can be difficult. At times, disease involving both systems may contribute to a patient’s shortness of breath.
Chest radiography and ECG are usually the initial studies of choice in the workup of a patient with acute shortness of breath [13]. The results of these studies can help to direct further workup. MDCT is currently the best imaging tool to assess diffuse lung disease, especially when clinical evaluation and laboratory studies as well as plain film radiographs are non-diagnostic [14, 15]. In some cases performing the study prone and/or in the expiratory phase may be helpful to evaluate the pattern of air trapping. Many conditions such as bronchiectasis, emphysema, sarcoidosis, and lymphangetic spread of neoplasm have characteristic imaging features on MDCT that permit a specific diagnosis or at least a limited differential, even when the chest radiograph is normal [16]. Computed tomography is the most sensitive technique for the identification of emphysema in cigarette smokers and can provide unique phenotypic information in COPD [17].
Contrast enhancement is useful to assess many cases of acute dyspnea because it can help to identify vascular etiologies such as PE, aortic dissection, and coronary artery disease. ECG gating is essential for the evaluation of both dissection and coronary artery disease because cardiac motion causes artifacts that limit the diagnostic capabilities of CT.
Pneumothorax
Pneumothorax is defined as collapse of all or part of a lung replaced by air in the pleural space. Pneumothorax may be primary without an identifiable cause or secondary to an iatrogenic procedure, trauma, or underlying lung disorders such as interstitial lung disease or emphysema. Since the advent of CT, most primary pneumothoraces have been shown to arise from rupture of small apical blebs that are not apparent on chest radiographs. A tension pneumothorax, because of the increased pressure in the pleural space on the side of the pneumothorax, causes mass effect on the cardiomediastinum and displaces it toward the contralateral hemithorax.
Because of lower cost, radiation, and ready availability, a chest radiograph is most commonly used to diagnose a suspected pneumothorax (Fig. 3.1). The sensitivity of radiographs for the detection of pneumothoraces is greatly influenced by patient positioning. Whenever possible, an upright PA view of the chest should be performed. This allows air not contained by loculations to track superiorly toward the lung apex such that the classic appearance of a visceral pleural line can be detected. If the radiograph fails to show the pneumothorax, a lateral decubitus (affected side up) or upright expiratory views can be acquired. It should be noted that the use of expiratory radiographs is controversial and when used alone can limit chest X-ray interpretation by creating false linear opacities. CT is the gold standard for the diagnosis of a pneumothorax with near 100 % sensitivity, but it gives a higher dose of radiation, costs more, and is not as available as radiography.
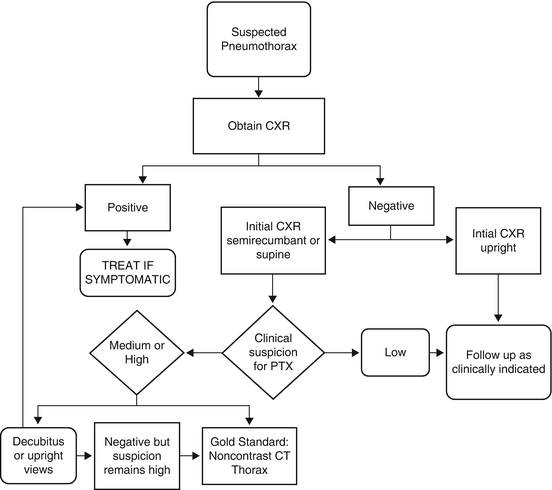
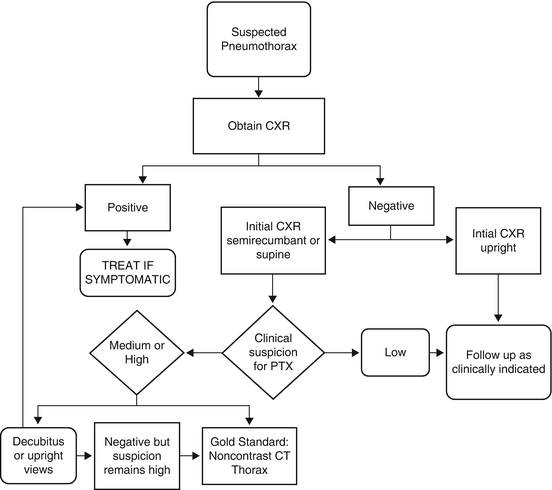
Fig. 3.1
Evaluation of pneumothorax
Unstable patients or those debilitated by trauma may not be able to tolerate a standard PA chest radiograph. In these cases a portable AP view of the chest may be performed at the bedside [18]. Should the patient remain in a fully upright position, little is lost in terms of sensitivity. The sensitivity for detection of pneumothorax on supine or semi-recumbent films decreases to around 28 % compared with 92 % on a fully upright PA view of the chest. This is the consequence of air tracking to the anteromedial pleural space when a patient is recumbent.
An occult pneumothorax is one that is not seen on conventional radiographs and only detectable on more advanced imaging. By comparing CT with supine AP radiographs in patients who have had trauma, the incidence of occult pneumothorax has been calculated to average approximately 5 %. The incidence varied considerably, ranging from 4 % in injured children, 22 and 17 % percent in blunt and penetrating trauma, and 64 % in multi-trauma intubated patients [19, 20].
Thoracic Trauma
After head and musculoskeletal injuries, thoracic trauma is the leading cause of morbidity and mortality, particularly in younger individuals. In a series of 24,000 patients with multiple injuries at a Level 1 trauma center, 70 % included CNS injuries, 90 % musculoskeletal injuries, and over 50 % thoracic injuries [21]. The major causes of thoracic trauma include blunt trauma typically related to a fall, crush injury, explosion, or most commonly a motor vehicle accident. In 2001, for example, 45 % of blunt traumatic injuries were related to MVA [22]. Penetrating trauma is usually caused by knife or bullet injuries. These cause focal lung and soft tissue damage such as laceration, pneumothorax, pneumatocoele, pulmonary contusion, tracheobronchial, vascular, cardiac, and pericardial injury [23].
The plain chest radiograph is usually the first study performed for thoracic trauma. Since the plain chest X-ray is usually performed in the recumbent position, life -threatening injuries such as cardiac bruise and aortic rupture leading to pseudoaneurysm may be difficult to identify. For these reasons CT has become the study of first choice in patients with both blunt and penetrating multiple organ system trauma. As the spatial and temporal resolution of CT has improved, the rationale for performing radiographs first has waned [24, 25].
Diaphragmatic Injury
Although diaphragmatic tears can occur as a result of penetrating trauma, most diaphragmatic injuries are associated with blunt trauma of the chest and abdomen. In one series, 3–6 % of patients with blunt trauma developed a tear in one or both diaphragmatic leaflets [26]. Possibly because of the protective nature of the liver, diaphragmatic ruptures are much more common on the left than the right side. Because of the geometry of the diaphragmatic muscle, lateral impact is a greater source of rupture than frontal or posterior injuries [27].
Plain radiographs may suggest the diagnosis of diaphragmatic tear or rupture because of diaphragmatic elevation, flattening of the costophrenic angle, superior-medial shift of the dome of the diaphragm, pleural effusion, or herniation of abdominal contents into the thorax with associated atelectasis. MDCT with reformatted images in the coronal and sagittal plane will show the diaphragmatic defect accurately and so confidentially establish the diagnosis of rupture. Occasionally when the MDCT does not diagnose the suspected defect, MRI is used. It is essential to recognize a diaphragmatic rupture because of greater than 15 % mortality rate associated with acute rupture and possible complications of massive bleeding, bowel obstruction, strangulation, or cardiovascular compression [26].
Sternal Injury
Sternal fractures occur in up to 4 % of patients with severe blunt trauma. They most often occur near or at the angle of Louis and may be associated with a retrosternal hematoma. Because of the orientation of the fracture it will be best demonstrated with a lateral chest X-ray or by CT. Sternal fractures may be associated with anterior rib fractures, cardiac contusion, pericardial herniation, hemopericardium, and aortic or other vascular injury [28].
Tracheobronchial Rupture
Tracheobronchial injury can occur in up to 3 % of patients from sudden tissue compression related to high velocity blunt trauma, such as a steering wheel injury. The usual sites of rupture are the main stem bronchi in 90 % and the distal trachea in 10 % of patients. Findings that suggest the diagnosis of bronchial rupture include pneumomediastinum, tension pneumothorax, and subcutaneous emphysema. Deformity of the involved airway and downward displacement of the detached lung, also termed the “Falling Lung Sign,” are strong clues to the diagnosis. Although the diagnosis of tracheobronchial rupture can occasionally be made on plain radiographs, MDCT is preferred since the tracheobronchial tree can be reconstructed in multiple planes and CT is more sensitive to detect ancillary findings [29, 30].
Acute Thoracic Aortic Injury
The majority of cases of acute aortic injury occur in patients with multisystem trauma, most often motor vehicle accidents. Nearly, 80–90 % of all patients with aortic laceration have a complete tear through the wall of the aorta and exsanguinate and die at the scene of the accident or at the hospital before initiation of treatment [30]. Patients who survive to be imaged often have partial aortic tears with intact adventitia, resulting in a contained rupture or minimal aortic injury affecting only the intimal layer of the aorta [30].
The vast majority of traumatic aortic tears occur at the aortic isthmus, within 2 cm. of the origin of the left subclavian artery. Traumatic injuries to the ascending aorta have a high acute mortality rate and so are uncommonly seen on imaging. These usually occur just distal to the aortic valve and often coexist with myocardial contusion, aortic valve rupture, and/or hemopericardium. Traumatic involvement of aortic arch and branch vessels is also less common, but potentially fatal. The extent of branch vessel injuries, like aortic injuries, ranges from subtle intimal injuries to complete transection and contained rupture. Mid and distal descending thoracic aortic involvement is uncommon, often occurring near the diaphragmatic hiatus.
A portable supine chest radiograph should be obtained initially to identify life-threatening lesions requiring immediate intervention, e.g., massive hemothorax or tension pneumothorax (Fig. 3.2). Another reason to obtain a chest radiograph is to diagnose a mediastinal hematoma, which may suggest a significant vascular injury. A widened mediastinum is the most common finding associated with mediastinal hematoma, others include blurring of the aortic outline, loss of the aortopulmonary window, left apical pleural cap, and right tracheal deviation. Unfortunately, a widened mediastinum on a portable chest radiograph is neither sensitive nor specific for acute aortic injury. Thus, a more definitive diagnostic test should be performed, regardless of the chest radiographic findings.
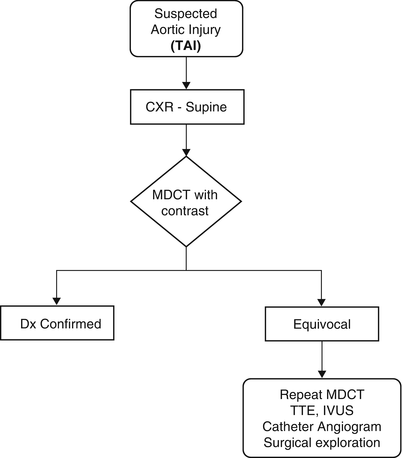
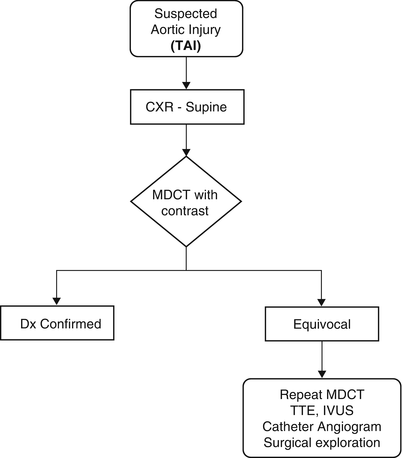
Fig. 3.2
Aortic Trauma Schematic
MDCT/CTA is currently the diagnostic test of choice for the definitive evaluation of acute aortic injury. The imaging protocol is similar to that for aortic dissection. Aortic injury is diagnosed using both direct and indirect signs. The most common indirect finding is a mediastinal hematoma. Periaortic hematoma that is contiguous with the aortic wall is an indeterminate finding for the source of bleeding and so the possibility of an aortic tear should be investigated further. Direct signs of aortic injury include intimal flap, pseudoaneurysm, focal contour abnormality, intramural hematoma, mural thrombus, and abrupt change in luminal caliber (pseudocoarctation). Direct findings are usually definitive, and unless there are extenuating circumstances, no additional imaging is necessary. If the aortic adventitia is breached, frank active extravasation of contrast from the aortic lumen is seen, but this is a rare imaging finding and often portends impending exsanguination.
Minimal aortic injuries, e.g., intimal flaps, are better visualized using high-resolution diagnostic techniques and multidetector scanners. In contrast to more severe aortic injury, in-hospital mortality from minimal aortic injury usually is not related to the aortic injury itself, and so these injuries may be amenable to conservative management. Nevertheless, close imaging surveillance to detect an adverse course of a minimal aortic injury is mandatory [31].
Acutely, MRI has a limited role in trauma patients because of logistical issues. MRI and MRA may have value once patients are stable when surgery is contemplated and minimal or equivocal intimal injuries are present. Of course, MRI eliminates the problem of radiation exposure in younger trauma victims [32].
When both MDCT and intra-arterial aortographic studies are inconclusive, intravascular ultrasonography (IVUS) can be helpful to diagnose traumatic aortic injury. It is invasive, requiring arterial puncture, and thus is ideally performed concurrently in association with catheter aortography. IVUS is an operator- and experience-dependent modality, and complete evaluation of the aorta can be time-consuming.
TEE is another modality that can be employed to evaluate the aorta when MDCT/CTA or intra-arterial aortographic studies are equivocal. TEE is widely available, minimally invasive, and can be performed quickly at the bedside or in the operating room. Since TEE is performed in real-time, the aortic valve, sinotubular junction, and ascending aorta can be evaluated to a much better extent with TEE than with MDCT [33].
Pericardial and Cardiac Trauma
Injury to the heart and/or the pericardium occurs in more than 2 % of serious chest trauma following a motor vehicle accident resulting from high energy impact followed by rapid deceleration [34]. Pneumohemopericardium, cardiac herniation, myocardial contusion, valve leaflet tear, aortic rupture, and ventricular septal defect have been described. While radiographs may show a sudden change in the size of the cardiac silhouette, CT with intravenous contrast is the preferred modality for diagnosis when pericardial or cardiac CT is suspected.
Imaging the Patient with Fever
While there are many causes of fever in the acute setting, pulmonary etiologies are particularly common. Viral, bacterial, and fungal infections, tuberculosis, tumor and noninfectious inflammatory conditions such as collagen-vascular disease, medications and illicit drugs such as cocaine, and heat exhaustion are among the many sources of respiratory-induced fever.
The role of the radiologist is to confirm the presence of an acute pulmonary abnormality and, if possible, narrow the differential diagnosis. Typically, plain radiographs suffice for this purpose. Lateral decubitus films may be helpful when pleural fluid is suspected but not confirmed by the PA and lateral projections. Decubitus views are especially important when an erect frontal and lateral examination cannot be performed. If the pneumonia is refractory to treatment or is suspected to be secondary to another source of pathology, e.g., neoplasm obstructing a bronchus, a CT scan of the thorax may be of value. CT scanning is also useful when a cavity is identified and a mycetoma is suspected. Furthermore, CT is useful in the diagnosis and staging of empyema, septic emboli, and fistulous tracts as one might see in actinomycosis or tuberculosis. Otherwise, the majority of pneumonias can be diagnosed and followed using standard radiographs. The frequency of and requirement for the performance of follow-up chest radiographs depend on the patient’s clinical status. In routine cases if the patient is recovering with cessation of fever and respiratory complaints, no further films are needed. On the other hand, some patents such as those with altered immune status should be followed at least monthly until the infiltrate has resolved [32].
Imaging the Patient with Hemoptysis
Although in most cases true hemoptysis, that is blood originating from the tracheobronchial tree and not the nasopharynx or GI tract, is not massive or life-threatening, it may be the harbinger of significant pathology. Common causes of hemoptysis include bronchitis (29 %), bronchogenic carcinoma (<29 %), bronchiectasis (25 % of patients), pneumonia (<20 %), pulmonary emboli (10 %), CHF (5 %), and vasculitis (<5 %) [35].
Controversy exists over the most efficacious diagnostic pathway to identify the etiology of hemoptysis. If a chest radiograph is unrevealing, both bronchoscopy and CT have been considered the next procedure of choice. On the one hand, bronchoscopy not only can provide direct visualization of the cause of the hemoptysis, but it also permits biopsy at the time of the procedure. If the bleeding is substantial, however, the airways may be filled with blood making bronchoscopic evaluation of the distal airways difficult. CT is noninvasive and is more likely than bronchoscopy to detect the underlying etiology of the bleeding [34]. In a study of 80 patients with large amounts of hemoptysis by Revel et al., the chest X-ray was normal in 13 %, revealed the cause of bleeding in 35 % and the site of bleeding in 46 %. CT was more efficient than bronchoscopy at identifying the etiology of the bleeding (77 % vs. 8 %) whereas the two modalities were comparable in identifying the site of bleeding [34]. The authors suggested that chest CT should replace bronchoscopy as the first line procedure for the evaluation of large amounts of hemoptysis.
The Solitary Pulmonary Nodule
The most important clinical question regarding a solitary pulmonary nodule (SPN) is whether or not it represents a malignancy [36]. An SPN is an opacity of less than 3 cm in diameter surrounded by normal lung parenchyma without associated atelectasis, pleural effusions, or adenopathy on chest radiography. Often, an SPN is discovered incidentally on radiographs obtained for other reasons. Because CT has better resolution than chest X-ray, an SPN can be characterized with CT as solid, mixed, semisolid, or ground glass. The presence of calcification and cavitation may also be seen to advantage with CT (Fig. 3.3).
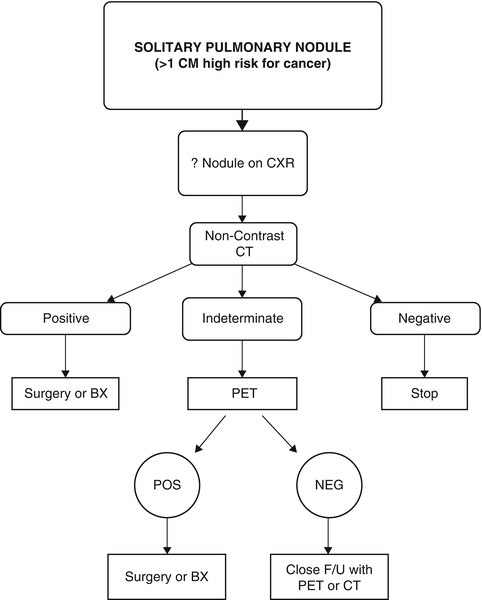
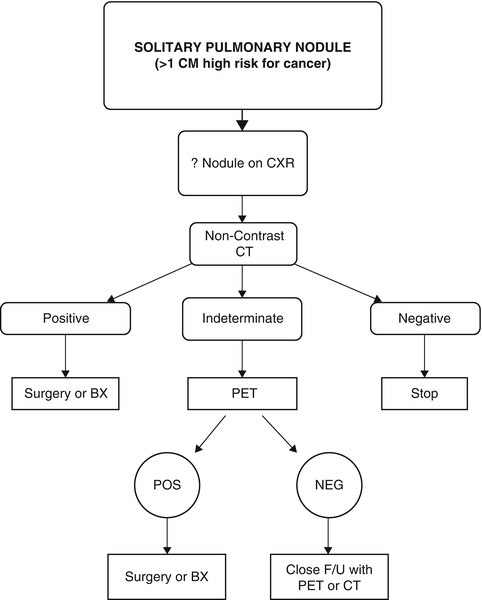
Fig. 3.3
Evaluation of the solitary pulmonary nodule
Only two imaging features of an SPN clearly exclude malignancy: a benign calcification pattern within the nodule and a solid lesion that is stable over a 2-year period. A ground glass or mixed lesion may represent adenocarcinoma in situ or bronchioalveolar carcinoma, which may show imaging stability for 2 years but then occasionally may exhibit later growth. Since other characteristics such as irregular borders, cavitation and shape do not exclude or definitively characterize an SPN as malignant, most lesions remain indeterminate.
Lesion size is a valuable indicator or possible malignancy. An SPN larger than 1 cm in diameter is much more likely to be malignant than a smaller nodule. A lesion of 6 mm is 30 times more likely to be benign than one that is larger [35]. The rate of growth of an SPN is also important. The typical lung cancer will double in volume in 4.2–7.3 months depending on cell type. A few will take up to 24 months and a few will double in as little as 1 month. Doubling times of less than 1 month suggest inflammatory disease, aggressive lymphoma, or aggressive metastases. Doubling times greater than 18 months suggest granuloma, carcinoid, hamartoma, or rounded atelectasis.
Positron emission tomography (PET) combined with CT has been used mainly in lesions larger than 7 mm in diameter. PET/CT may suggest malignancy when the FDG levels are elevated sufficiently. Certain types of adenocarcinoma and typical carcinoids have low FDG levels and may be confused with benign disease. An inflammatory SPN may have elevated FDG and be confused with low-grade malignant lesions. Thus, PET/CT is useful but not foolproof for the diagnosis of malignant versus benign SPN.
Recommendations for CT follow-up of an SPN smaller than 1 cm have been suggested by the Fleischner Society and have gained widespread acceptance in the radiology community [37]. A series of screening studies have shown that lesions smaller than 4 mm in diameter require at most a 1 year follow-up interval. Lesions larger than 8 mm may require intervention with transthoracic needle aspiration biopsy, thorascopic biopsy, or PET/CT scanning. These recommendations do not apply to patients who either have a known malignancy or who are under the age of 35 years (Table 3.4).
Table 3.4
Guidelines of the Fleischner Society for follow-up imaging of solitary pulmonary nodules
Nodule size (mm) | Low-risk patient | High-risk patient (months) |
---|---|---|
<4 | None | 12 |
4–6 | 12 months | 6–12 |
6–8 | 6–12 months | 3–6 |
>8 | 3, 6, and 12 months or PET/CT or biopsy |
Imaging the Patient with Acute Chest Pain
Chest pain is a common and challenging clinical complaint seen in all age groups. In a recent Center for Disease Control and Prevention Survey, 5.8 million (5.1 %) of emergency department visits were related to chest pain and represented the second leading cause for visits to a physician [38–40
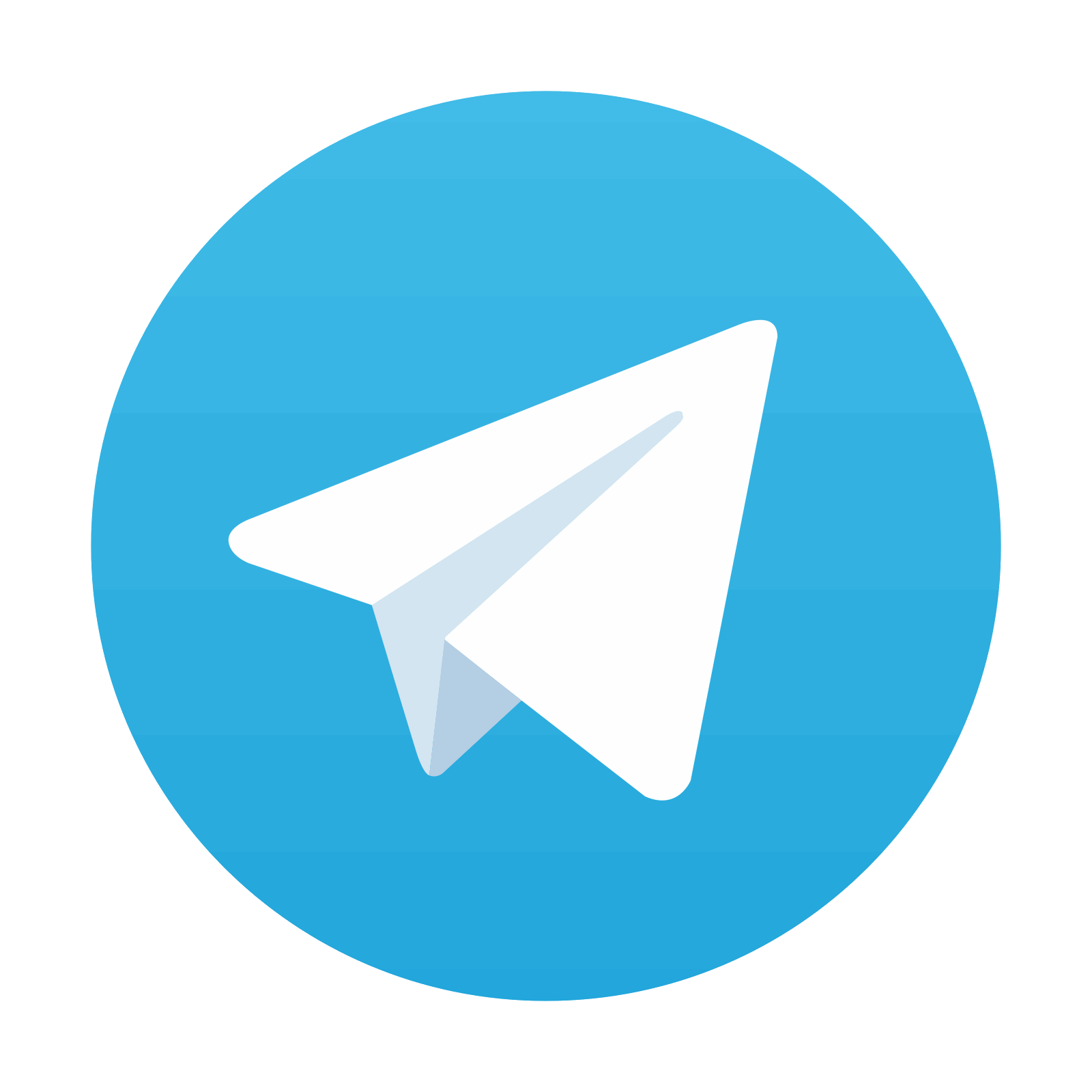
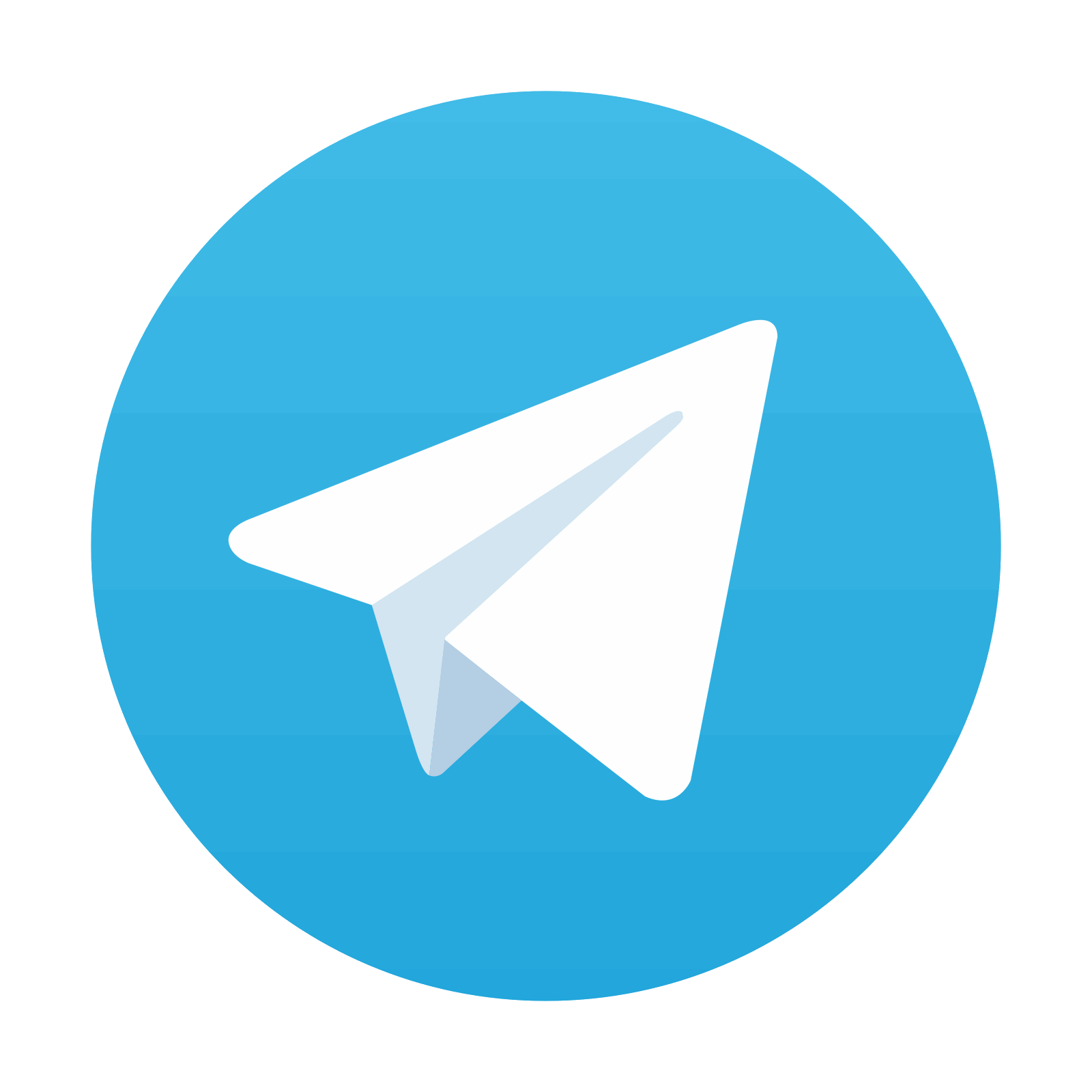
Stay updated, free articles. Join our Telegram channel

Full access? Get Clinical Tree
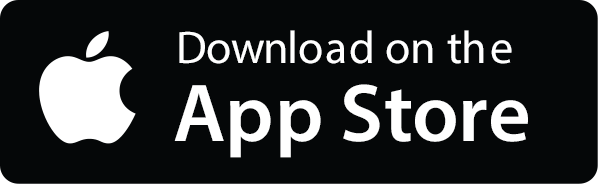
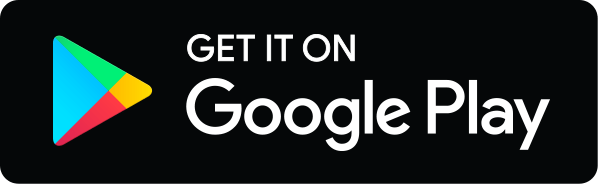