Fig. 18.1
Deaths by cause in individuals aged 0–59 years in LMICs in 2004. Cardiovascular diseases (CVDs) are the leading cause of death of all noncommunicable diseases (NCDs) [4]
These statistics are very alarming because patients with CVD in low- and middle-income countries (LMICs) tend to have poorer outcomes and die younger than their counterparts in the United States (US) and other high-income countries. The burden of disability caused by CVD is extensive (Fig. 18.2), and not surprisingly, emerging evidence indicates that CVD contributes to poverty in LMICs as a result of its negative effects on household and countrywide economics [3]. According to the World Health Organization (WHO), heart disease, stroke, and diabetes together reduce gross domestic product (GDP) by an estimated 1–5 % in rapidly growing LMICs [3].
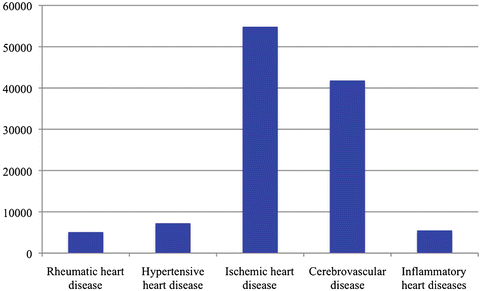
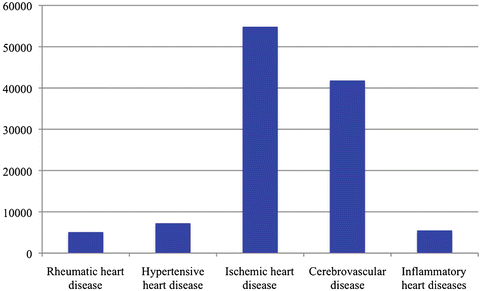
Fig. 18.2
Burden of CVD in disability-adjusted life years (thousands) by type in LMICs in 2004. Inflammatory heart diseases include myocarditis, endocarditis, pericarditis, and cardiomyopathies. As shown here, CVD is a significant contributor to disability and economic drag [4]
Despite the grave impact of CVD on the health and overall well-being of individuals in the developing world, efforts to reduce them are grossly underfunded [4], with many donor countries operating under a policy ban on funding NCD programs. Access to cardiac imaging services is particularly limited. Some equipment is prohibitively costly to install or maintain, and using it properly can be challenging. The WHO estimates that approximately 95 % of imaging technology is imported into developing countries, and over 50 % of this equipment is not utilized due to inadequate servicing or a paucity of trained personnel [5].
Comprehensive training programs in cardiac imaging for technicians and cardiologists are nonexistent in most LMICs. Consequently, images may be captured incorrectly or patients may be exposed to unnecessary radiation. In addition, physicians may order tests inappropriately or incorrectly interpret the results, leading to erroneous diagnoses and poor patient outcomes. In advanced healthcare systems, most cardiac images are now transferred over wireless networks and stored digitally, but many developing nations do not have high bandwidth networks in place. These realities are troublesome because diagnostic imaging plays a very important role in early detection and treatment of CVD. Without accessible or reliable cardiac imaging programs, intervention is delayed.
In order to meaningfully expand the global availability of cardiac imaging, it is necessary to understand the etiologies of CVD in developing regions (Fig. 18.3). In contrast to the industrialized world, rheumatic heart disease (RHD) and infectious diseases with cardiac manifestations (e.g., Chagas disease, tuberculosis, or schistosomiasis) cause substantial morbidity and mortality. RHD reportedly affects 15.6–19.6 million people worldwide and causes up to 492,000 deaths each year, 95 % of which occur in developing countries [6]. Additionally, many children and young adults in developing nations suffer from untreated or unrecognized congenital heart defects. In developing countries with a high burden of human immunodeficiency virus and acquired immunodeficiency disease syndrome (HIV/AIDS), CVDs due to the infection itself or antiretroviral treatment are widespread. As in industrialized nations, ischemic heart disease is a growing problem, and cardiac risk factors are on the rise. India’s population in particular is overwhelmed by these health hazards, with 69.8 million cases of diabetes mellitus and 214 million cases of hypertension projected by 2025 [7]. Adequate control of CVD risk factors (physical inactivity, tobacco use, obesity, diabetes, hypertension, hypercholesterolemia) has been projected to prevent 80 % of premature heart disease, stroke, and diabetes, underscoring the need for earlier identification of patients whose cardiovascular future is still modifiable [2].
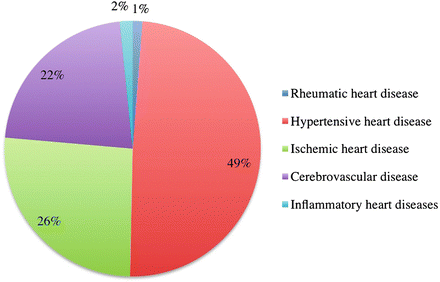
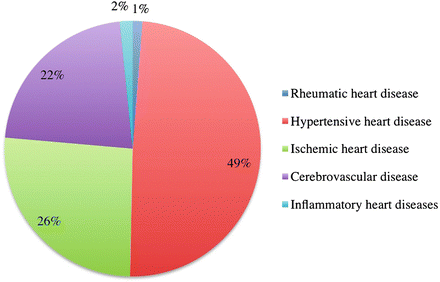
Fig. 18.3
Percentage of deaths from CVDs in LMICs in 2004 by disease type. Inflammatory heart diseases include myocarditis, endocarditis, pericarditis, and cardiomyopathies [4]
This chapter will provide a review of advanced techniques in cardiac imaging and then highlight approaches that have been successfully implemented in developing countries. Appropriate referral for cardiac imaging and follow-up after testing will be noted. Finally, future directions for the continued improvement of cardiac imaging globally will be discussed.
Current Cardiac Imaging
The field of cardiac imaging is rapidly evolving, with frequent introduction of new or improved technologies into clinical practice. This section will survey the tools employed in advanced healthcare systems.
Echocardiography is the most common diagnostic imaging procedure performed in cardiac patients [8]. Echo can be used to obtain two-dimensional (2D) or three-dimensional (3D) images, which can be augmented by color and spectral Doppler recordings or injection of intravenous (IV) contrast. 2D echo reliably measures cardiac chamber volumes and ejection fraction [9–11], but 3D echo may have an incremental advantage in the assessment of congenital heart disease (CHD) and the right ventricle [12].
Doppler provides hemodynamic information based on changes in frequency that result from the reflection of sound waves off of red blood cells. Color flow Doppler is included in most echo exams in order to screen for disturbed or turbulent blood flow [13]. Doppler can also be used to evaluate myocardial contractile performance, either by quantifying regional strain (tissue Doppler imaging) or by tracking individual myocardial speckles of grayscale images [14].
Another application in cardiac echocardiography is the use of contrast-enhanced ultrasound (CEUS). This modality utilizes the property that, in ultrasound, contrast is produced via the different ways in which sound waves are reflected from interfaces between substances or tissues. Gas-filled microbubbles can be administered intravenously to the systemic circulation that can be visualized with ultrasound as they travel through the heart or other structures due to their high degree of echogenicity (the ability of an object to reflect the ultrasound waves). In this way, CEUS can be used to image blood perfusion in organs, measure blood flow rate in the heart and other organs, and has other applications as well. In the case of contrast-enhanced echocardiography, IV microbubbles are injected to opacify the left ventricle (LV) so that areas of abnormal myocardial contraction can be identified [13]. In most cases, transthoracic echo (TTE) sufficiently characterizes cardiac function and anatomy. However, because the esophagus is adjacent to the left atria and thoracic aorta, transesophageal echo (TEE) is a better tool to visualize these posterior cardiac structures, prosthetic cardiac valves, and structures less than 3 mm in size [15, 16]. Additionally, TEE is especially valuable in the diagnosis of infective endocarditis (Fig. 18.4), aortic dissection, and in guiding interventional procedures [17, 18].
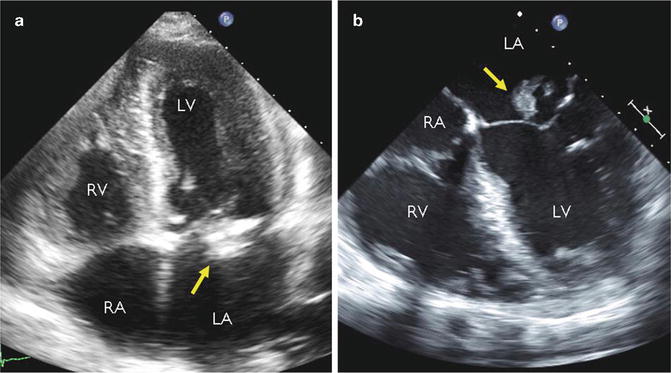
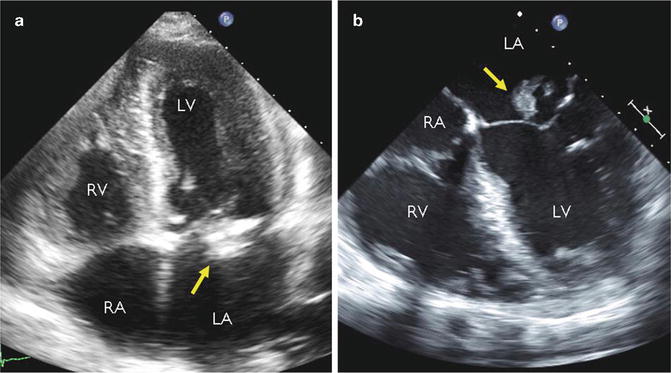
Fig. 18.4
Representative images acquired by transthoracic echocardiography (TTE, a) and transesophageal echocardiography (TEE, b) in the same patient with mitral valve endocarditis (arrowhead points to bacterial vegetation adherent to the anterior leaflet). The proximity of the mitral valve to the esophagus and superior acoustic window results in improved spatial resolution of TEE over TTE. RA right atrium, RV right ventricle, LA left atrium, LV left ventricle
Echocardiography can also be used for stress testing. Images acquired before and after either exercise- or pharmacologic-based stress may point to obstructive CAD by the presence of wall motion abnormalities that indicate inadequate perfusion, but cardiac angiography is the gold standard for viewing coronary anatomy. Stenotic lesions, intracoronary thromboses, atherosclerotic disease, areas of poor perfusion, and collateral vasculature are easily evaluated by this method [19]. Left ventriculography is included in most studies and employs contrast opacification of the LV to reveal wall motion abnormalities, which may be consistent with myocardial infarction. Capturing angiographic data is invasive and technically complex; it involves passage of a catheter through the femoral or radial artery, injection of IV contrast, and circling of an X-ray source and an image intensifier at a 180° angle to each other around the patient. Although complications are rare, the invasive nature of cardiac catheterization entails inherent risks, which can include myocardial infarction, stroke, infection, blood loss, and pain.
Nuclear imaging is very important in the care of patients with CAD. Its main indications include assessing the likelihood of CAD in intermediate-risk patients (high-risk patients are better suited for cardiac catheterization) and estimating the presence or amount of ischemia in patients with known disease. More than 95 % of all nuclear cardiology procedures are gated single-photon emission computed tomography (SPECT) myocardial perfusion imaging (MPI) [20]. SPECT MPI can be performed at rest or with the patient under stress. Images are taken with a scintillation camera that detects IV-injected radiopharmaceuticals distributed within the heart in proportion to myocardial perfusion [20], and perfusion defects may signify obstructive CAD (Fig. 18.5). Commercial software packages can assist in image interpretation, but results should always be checked by a trained individual [21]. Positron emission tomography has similar applications in the diagnosis and risk stratification of CAD, but is less commonly employed. Positron-emitting isotopes of elements can be inserted into biomolecules, and the radioactivity concentrations of these tracers in blood or myocardium can be noninvasively determined over time.
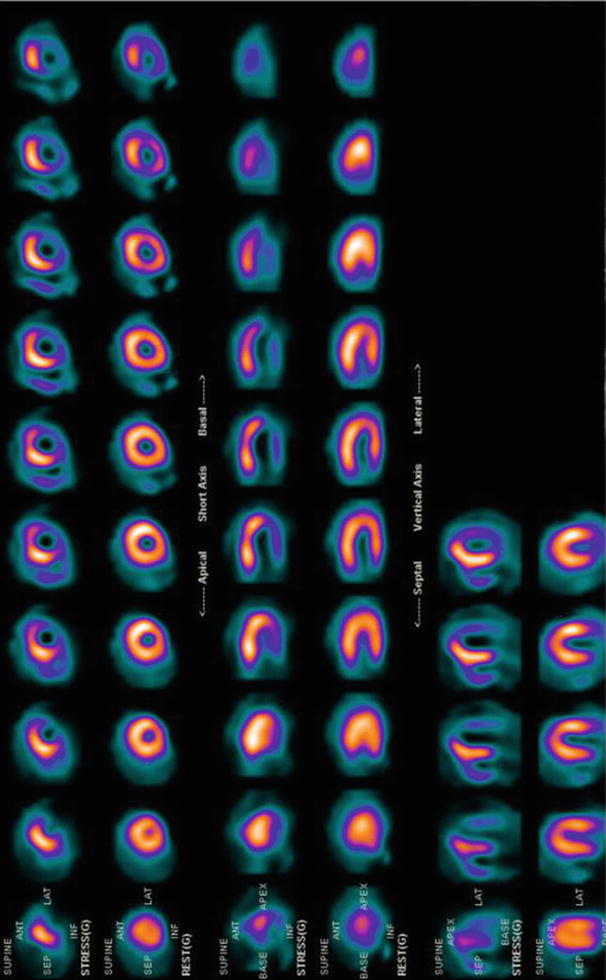
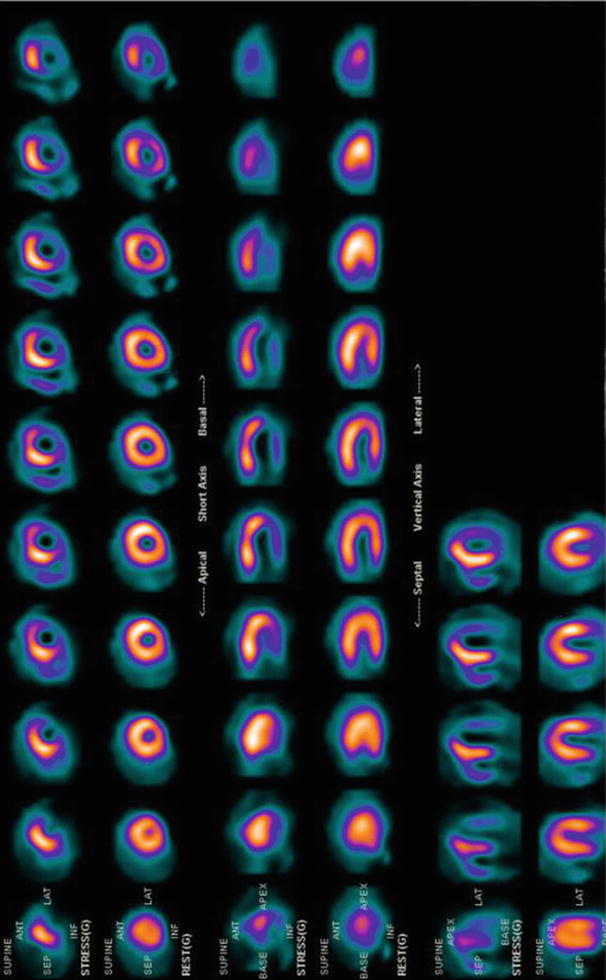
Fig. 18.5
Representative single-photon emission computed tomography myocardial perfusion imaging (SPECT MPI) demonstrating normal perfusion at rest (lower images in each series) and a perfusion defect after stress (upper images in each series). After exercise, a new perfusion defect was observed in the inferior and lateral walls, signifying severe ischemia. This post-bypass patient had a 99 % stenosis of a saphenous vein graft supplying these territories
Cardiac computed tomography (CT) has applications in many cardiac pathologies, including CAD, anomalous coronary arteries and other CHDs, valvular calcification or stenosis, pulmonary artery embolism, pericardial disease, and cardiac masses [22, 23]. Images can be obtained with multidetector CT (MDCT) by advancing the patient through the circular trajectory of an X-ray tube or by generating X-ray images from an electron beam, as in electron-beam computed tomography (EBCT). EBCT is preferred for calculating coronary artery calcium scores (CACS), whereas MDCT produces better images (Fig. 18.6) with cardiac CT angiography (CCTA) of diagnostic quality for the determination of obstructive CAD [24]. CACS not only correlates with the number of conventional CAD risk factors but is also an independent risk factor for future coronary events [25–27].
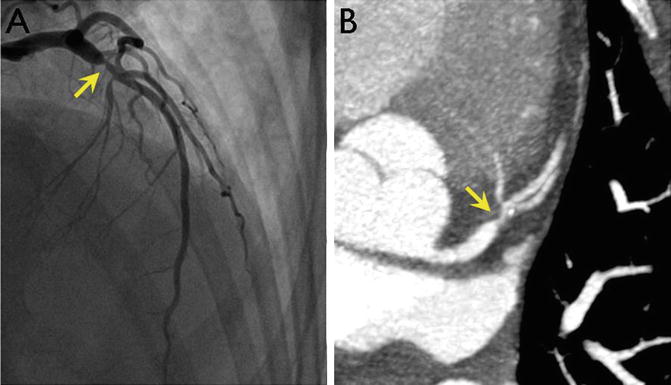
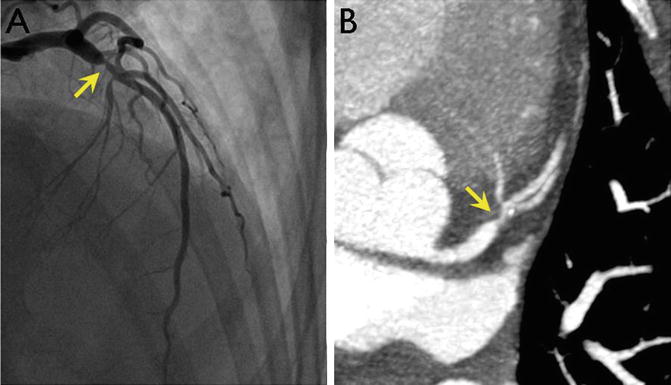
Fig. 18.6
Images of a proximal left anterior descending (LAD) coronary artery stenosis (arrowhead) obtained by cardiac catheterization (a) and coronary CT angiography (b). CT allows noninvasive imaging of the coronary arteries with some loss of resolution but without the complications associated with invasive procedures. Images courtesy of Robert Zeman, MD, George Washington University
Cardiac magnetic resonance (CMR) utilizes sophisticated technology to generate a static magnetic field that is about 30,000 times that of the earth, transmit energy to the patient within the radiofrequency range, and interpret the resulting signal [28]. CMR is the emerging modality of choice for determining ejection fraction, myocardial viability (Fig. 18.7), and evaluation of CHD [29, 30]. CMR with adenosine stress perfusion captures the transit of IV contrast, which is typically gadolinium-based, through the myocardium of the LV in order to evaluate ischemic heart disease, and CMR with dobutamine stress perfusion assesses for wall motion abnormalities that may signify obstructive CAD.
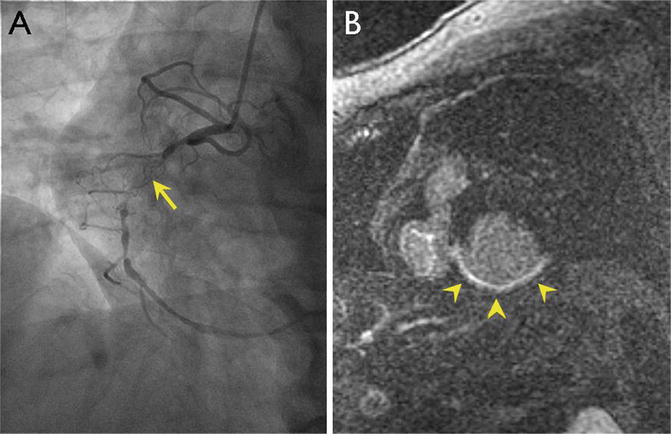
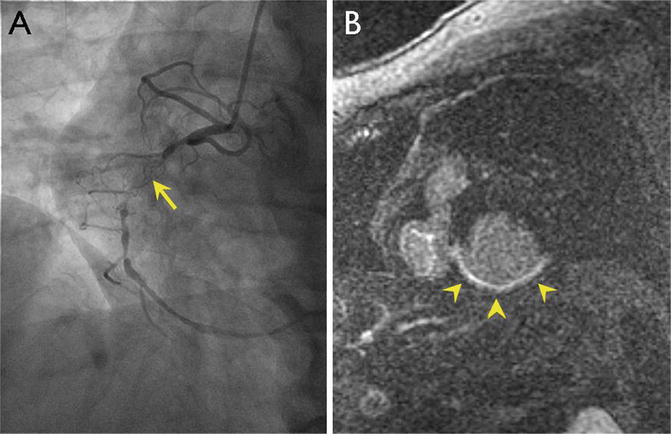
Fig. 18.7
Representative myocardial viability study by MRI. Cardiac catheterization (a) demonstrates a total occlusion (arrowhead) of the right coronary artery (RCA). Assessment of viability was done by MRI. In the short axis view of the left ventricle by MRI, late gadolinium enhancement is seen in areas of nonviable myocardium (arrowheads in b), suggesting that the myocardium supplied by the RCA would not benefit from revascularization. Images courtesy of Robert Zeman, MD, George Washington University
Approaches to Cardiac Imaging in the Developing World
As previously discussed, there are various challenges to the implementation of cardiac imaging technology in developing countries. This section will review effective approaches in LMICs.
Echo is by far the most widely used cardiac imaging tool in developing countries. This modality is well suited for low-resource settings for the same reasons that physicians in the developed world have so readily adopted its use in the clinic or at the hospital bedside. It is small, portable, relatively inexpensive, and does not deliver radiation. Moreover, although the incidence of CAD is growing in LMICs, hypertensive heart disease, cardiomyopathies, and valvular defects as sequelae of infectious etiologies make up a large percentage of cardiac disease and are easily evaluated by echo.
Portable echo in particular has proven to be very useful. A group of German physicians were one of the first to describe their experience with it in a remote tropical setting in 1990 [31]. They used ultrasound to evaluate 67 patients at a hospital in central Sudan. Cardiac abnormalities identified included valvular disease, pericardial effusion, dilated cardiomyopathy, CHD, mitral valve prolapse, and cardiac masses.
More specifically, echo is very valuable in diagnosing RHD in developing countries. A group of Kenyan physicians who employed echo to identify functional consequences of RHD reported that even in schools without electricity, it was feasible to carry out echo exams using a portable generator [32].
Marijon et al. took advantage of more recently available high-quality portable ultrasound equipment to screen for RHD in a large population of school-aged children in two developing nations, Cambodia and Mozambique [33]. They employed an echo machine with a Philips Sonos 4500 4–7 MHz transducer and recorded images on super-VHS videotape. The results of this study showed that systematic screening with echo, as compared with clinical screening, identifies approximately ten times the number of cases of RHD. This again highlights the usefulness and practicality of echo in the developing world. Similar findings were reported for more than 10,000 children evaluated with echo versus clinical screening in the southern Pacific islands of Tonga and India [34, 35].
Echo screening for RHD is also fast, inexpensive, and accurate even when performed by a nonexpert [36]. A trial of more than 362 children aged 5–14 years in Fiji showed that patients could be evaluated by echo in less than 4 min on average by an individual with no prior echo experience and at a cost of less than 40 US dollars per case of RHD detected. Given all of the advantages of RHD detection by echo, the World Heart Federation has recently published standardized criteria for the echocardiographic diagnosis of RHD [37]. The goal is to provide quicker and more reliable identification of RHD in patients worldwide so that treatment with penicillin is started before irreversible damage is done.
Portable echo is helpful in the evaluation of many other diseases endemic to developing countries. It was used to assess cardiac function and hemodynamic status in 30 children admitted to a Kenyan hospital with severe malaria [38]. Moreover, hand-carried cardiac ultrasound (HCU) devices proved to be a feasible method for diagnosis of various cardiac conditions in a cardiology clinic in rural Mexico [39]. The HCUs were about the size of a laptop computer, battery-operated, and weighed 2.9 kg. They generated 2D echo images as well as color flow Doppler. In more than half of the patients (63 out of 126), a focused exam was performed to investigate signs and symptoms that included chest pain, dyspnea, fatigue, peripheral edema, palpitations, syncope, cardiac murmurs, or abnormal electrocardiographs. Images produced by the HCU clarified the clinical picture in 93 % of cases and confirmed a cardiac diagnosis in 63 % of cases.
Innovation in echo technology continues to expand its utility in the developing world. A pocket-sized ultrasound (PCU) device is a new tool that is approximately the size of a smartphone and has comparable accuracy to traditional echo modalities [40–42].
Our collaborative group at George Washington University in Washington, DC, used a PCU in a remote Honduran village where echo was previously unavailable to make point-of-care diagnoses that were remotely verified by experts in the United States who reviewed the images on a smartphone-based application [43]. PCU assessments on 89 Honduran patients referred by local general providers were performed by a cardiology fellow. The most common indications for examination included arrhythmia, cardiomyopathy, and syncope. The PCU device used was small as its name suggests, measuring 1.4 × 7.3 × 2.8 cm and weighing 390 g, with an 8.9 cm diagonal display. It was equipped with color flow Doppler. Captured images were transmitted directly from the field via a dialup modem or via a broadband connection in an urban center where connectivity was limited. Experts then viewed the images at a different location and modified diagnoses suggested by the fellow as needed (Fig. 18.8). This approach was validated against the standard workstation evaluation and enabled patients to receive sophisticated care in areas with minimal capabilities and expertise.
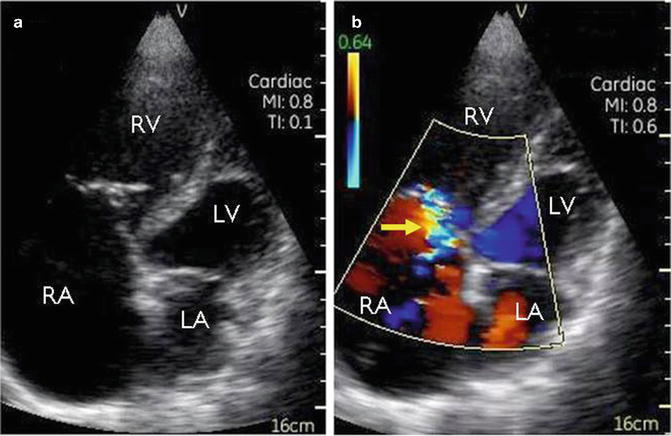
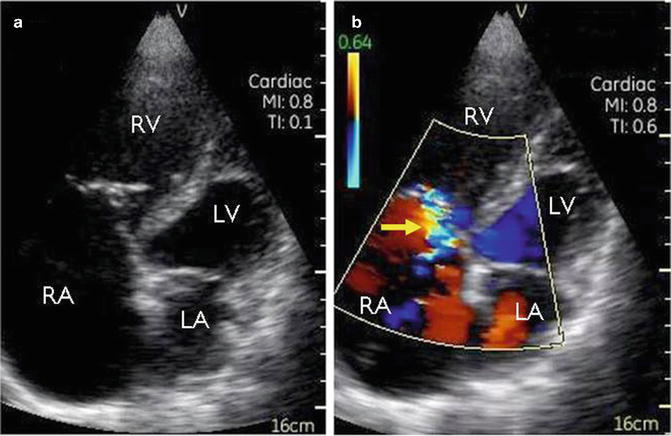
Fig. 18.8
A representative case in which expert remote interpretation corrected point-of-care diagnosis [45]. During a medical mission in a developing country, a cardiology fellow sent for remote evaluation these echocardiographic images taken with a handheld ultrasound device from a 43-year-old man who presented for evaluation of dyspnea. The fellow correctly identified the markedly enlarged right atrium and ventricle (a), but the mosaic pattern seen with color Doppler (b) in the tricuspid regurgitant jet (arrowhead) led the fellow to believe that severe regurgitation was present; however, expert acknowledged that the small jet size was more consistent with high-velocity mild regurgitation. RA right atrium, RV right ventricle, LA left atrium, LV left ventricle
In addition to its clinical applications, echo has great potential as a research instrument in developing countries. Despite having little funding, researchers from low-resource countries have employed it as a means for clinical discovery. For example, in Nigeria alone, several trials have employed echo in the investigation of various CVDs, including hypertensive heart disease, heart failure, dilated cardiomyopathy, and valvular heart disease [44–48].
Speaking more generally, the availability of all imaging modalities, including echo, is concentrated in capital cities [49, 50]. The cardiac imaging equipment in these facilities is similar to what is available in the United States and wealthy European countries. For example, hospitals in urban areas of India have rapidly adopted advanced diagnostic techniques such as CMR, CTCA, or coronary angiography [51]. However, they are often provided on a fee-for-service basis at a cost that is unaffordable for the majority of the population. The average cost to patients of SPECT MPI with a Technetium-99-m tracer is approximately $180. While this is markedly more affordable than in industrialized nations, it is still more than one-third of the average monthly middle-class income of about $500 in India [51].
Regarding nuclear imaging, rates of use reflect a country’s GDP and are highest in developing countries that border “high user” nations, such as the United States or countries in the European Union [52]. This information suggests some degree of diffusion of expertise and resources from neighboring developed countries and has implications for future initiatives in expanding the availability of advanced cardiac imaging tools in LMICs. Additionally, in some countries, novel methods are used to decrease the cost of a nuclear cardiology procedure to even lower than what it would typically cost in the developing world. These include greater use of generic drugs and radiopharmaceuticals as well as cheaper labor costs [52].
Developing countries acquire some equipment through international donation of used equipment. Many LMICs also rely on training programs provided at minimal cost by developed nations. The International Atomic and Energy Agency (IAEA) has created a module about radiation protection (RP) that is tailored to the needs of interventional cardiologists in developing countries [53]. RP is an important subject in patient and operator safety but is overlooked in many developing countries because of the lack of educational resources. In some LMICs, cardiac imaging education is part of the curriculum for cardiology training, but there is often no formal accreditation process to denote expertise in a specific imaging modality as there is in the United States or Europe [49].
In addition to the IAEA, which focuses on the global expansion of nuclear techniques like SPECT MPI, there are several charitable organizations that work, at least in part, to advance cardiac imaging in developing nations. SonoWorld is dedicated to providing free or low-cost educational materials and information on ultrasound, which includes echo and vascular examinations. Its website features video lectures on echo; digital chapters on ultrasound of the carotid arteries, deep venous thromboses, and the vascular systems; and links to commercial websites. Imaging the World is an organization that seeks to increase availability of echo and other types of ultrasound in developing countries, and plans relief trips to these regions. RAD-AID International assists developing nations in expanding their health imaging services, including echo and other cardiac imaging devices. The organization spreads awareness of cardiac imaging technology in low-resource settings through its annual conference and website featuring a bibliography of relevant medical research. RAD-AID also facilitates volunteering opportunities and global distribution of cardiac imaging resources.
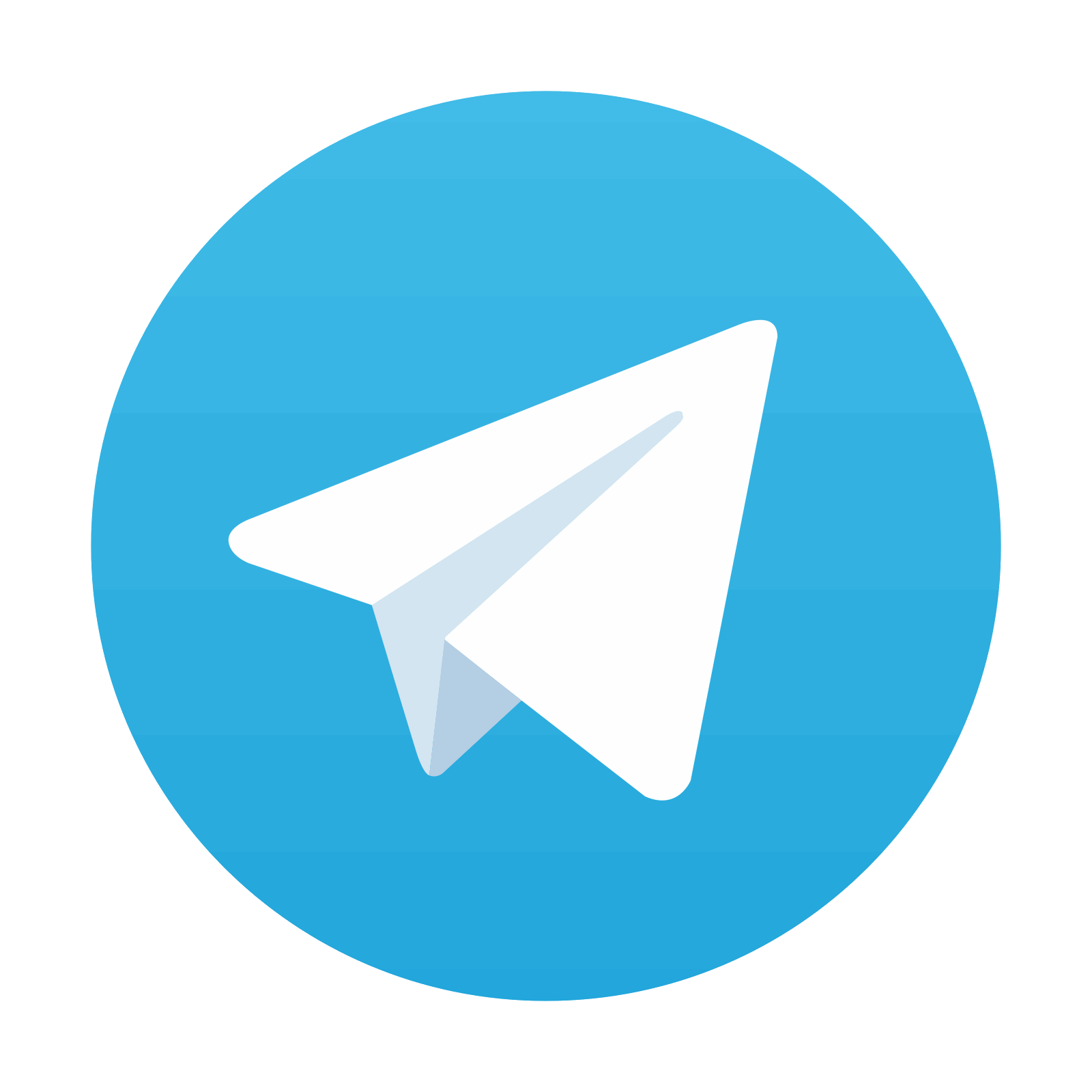
Stay updated, free articles. Join our Telegram channel

Full access? Get Clinical Tree
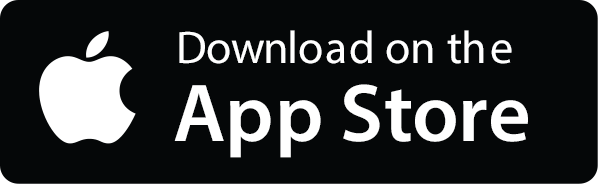
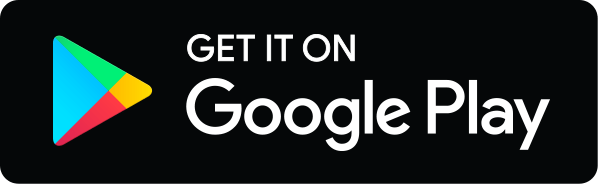